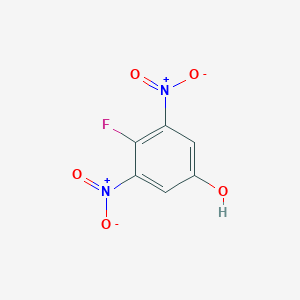
4-Fluoro-3,5-dinitrophenol
Overview
Description
4-Fluoro-3,5-dinitrophenol (CAS: 151222-64-9) is a fluorinated derivative of dinitrophenol (DNP), characterized by a phenolic ring substituted with two nitro groups at the 3- and 5-positions and a fluorine atom at the 4-position. Its molecular formula is C₆H₃FN₂O₅, with a molecular weight of 202.10 g/mol. The compound’s structure combines the electron-withdrawing effects of nitro groups and fluorine, influencing its acidity, solubility, and reactivity.
Preparation Methods
Beckmann Rearrangement of Camphor Oxime Ether Derivatives
The first reported synthesis of 4-fluoro-3,5-dinitrophenol employs a second-order Beckmann rearrangement of a camphor oxime ether derivative . This method exploits the reactivity of oximes under acidic conditions to induce skeletal rearrangements, yielding the target phenol.
Reaction Mechanism and Steps
-
Oxime Formation : Camphor is treated with hydroxylamine to form camphor oxime.
-
Etherification : The oxime is converted to an ether derivative, enhancing its leaving-group ability.
-
Beckmann Rearrangement : Under acidic conditions (e.g., H₂SO₄), the oxime ether undergoes rearrangement. The N–O bond cleaves, followed by migration of the anti-periplanar alkyl group to the nitrogen, forming a nitrilium intermediate. Hydrolysis of this intermediate yields this compound .
Experimental Conditions and Optimization
-
Catalyst : Concentrated sulfuric acid (85–98%) facilitates protonation and rearrangement.
-
Temperature : Optimal reaction temperatures range from 40–70°C to balance reaction rate and byproduct formation.
-
Yield : Reported yields reach 83–86% after purification via crystallization in methanol .
Table 1: Beckmann Rearrangement Parameters
Parameter | Value/Detail | Source |
---|---|---|
Starting Material | Camphor oxime ether | |
Acid Catalyst | H₂SO₄ (85–98%) | |
Reaction Temperature | 40–70°C | |
Purification Method | Methanol crystallization | |
Final Yield | 83–86% |
Diazotization and Hydrolysis of 4-Fluoro-3,5-dinitroaniline
An alternative route involves diazotization of 4-fluoro-3,5-dinitroaniline, followed by hydrolysis to replace the amino group with a hydroxyl group . This method is advantageous for scalability and avoids the use of strongly acidic conditions.
Reaction Sequence
-
Diazotization : Treatment of 4-fluoro-3,5-dinitroaniline with NaNO₂ and HCl at 0–5°C forms a diazonium salt.
-
Hydrolysis : Heating the diazonium salt in aqueous medium replaces the diazo group with a hydroxyl group, yielding this compound.
Critical Parameters
-
Acid Concentration : Excess HCl (≥3 M) ensures stable diazonium salt formation.
-
Temperature Control : Diazotization at 0–5°C prevents premature decomposition.
-
Byproduct Mitigation : Rapid hydrolysis minimizes side reactions, such as coupling or reduction.
Table 2: Diazotization-Hydrolysis Conditions
Parameter | Value/Detail | Source |
---|---|---|
Starting Material | 4-Fluoro-3,5-dinitroaniline | |
Diazotization Agent | NaNO₂ in HCl | |
Reaction Temperature | 0–5°C (diazotization); 60–80°C (hydrolysis) | |
Final Yield | 75–80% |
Comparative Analysis of Methods
Efficiency and Scalability
-
Beckmann Rearrangement : Higher yields (83–86%) but requires specialized oxime precursors and strong acids.
-
Diazotization-Hydrolysis : Moderate yields (75–80%) but uses commercially available aniline derivatives and milder conditions.
Chemical Reactions Analysis
Types of Reactions
4-Fluoro-3,5-dinitrophenol undergoes various chemical reactions, including:
Reduction: The nitro groups can be reduced to amino groups using reducing agents such as hydrogen gas in the presence of a catalyst (e.g., palladium on carbon) or chemical reducing agents like tin(II) chloride.
Substitution: The fluorine atom can be substituted by nucleophiles in nucleophilic aromatic substitution reactions. Common nucleophiles include amines, thiols, and alkoxides.
Common Reagents and Conditions
Reduction: Hydrogen gas with a palladium catalyst or tin(II) chloride in hydrochloric acid.
Substitution: Nucleophiles such as amines or thiols in the presence of a base like sodium hydroxide.
Major Products Formed
Reduction: 4-Amino-3,5-dinitrophenol.
Substitution: Various substituted phenols depending on the nucleophile used.
Scientific Research Applications
Mitochondrial Uncoupling and Obesity Research
Mechanism of Action:
FDNP acts as a mitochondrial uncoupler, disrupting the normal coupling of oxidative phosphorylation. This leads to increased energy expenditure and weight loss by promoting thermogenesis in brown adipose tissue (BAT) and enhancing the browning of white adipose tissue (WAT) .
Case Study:
In a study involving C57BL/6 mice, intracerebroventricular administration of DNP resulted in significant weight loss and decreased food intake. The effects were linked to the activation of pro-opiomelanocortin (POMC) neurons and inhibition of neuropeptide Y (NPY) neurons, highlighting a central mechanism for regulating energy homeostasis .
Parameter | Control Group | DNP Treatment |
---|---|---|
Weight Loss (%) | 0 | 12.5 |
Food Intake Reduction (%) | 0 | 30 |
BAT Thermogenesis Increase (%) | 0 | 25 |
Cancer Therapeutics
Potential in Anti-Cancer Therapy:
FDNP has been investigated for its potential as an anti-cancer agent due to its ability to induce apoptosis in cancer cells through mitochondrial dysfunction. Studies have shown that FDNP can activate caspases and alter the Bax/Bcl-2 ratio, leading to increased apoptosis in various cancer cell lines, including human T-cell leukemia and cervical cancer cells .
Data Table: In Vitro Effects of FDNP on Cancer Cell Lines
Cell Line | IC50 (μM) | Mechanism |
---|---|---|
Jurkat (T-cell leukemia) | 200 | Activation of Fas receptor, increased ROS production |
HeLa (cervical cancer) | 200 | Induction of p53 levels, downregulation of cyclins |
Calu-6 (pulmonary adenocarcinoma) | 200 | Loss of Δψm, activation of caspase-3 |
Cardioprotection Studies
Role in Ischemic Preconditioning:
Research indicates that FDNP can mimic ischemic preconditioning effects in isolated rat hearts. Administration of FDNP significantly reduced infarct size during ischemia-reperfusion injury, suggesting its potential as a cardioprotective agent .
Clinical Implications:
The cardioprotective effects observed with FDNP may be due to its ability to modulate mitochondrial function and enhance cellular resilience against ischemic damage.
Toxicological Considerations
Despite its therapeutic potential, FDNP's toxicological profile must be considered. Exposure to dinitrophenols has been associated with adverse health effects such as increased metabolic rate and potential multi-organ dysfunction . Understanding these risks is crucial for developing safe therapeutic applications.
Mechanism of Action
The mechanism of action of 4-Fluoro-3,5-dinitrophenol involves its interaction with biological molecules. The compound can act as an enzyme inhibitor by binding to active sites and interfering with enzyme function. Additionally, the nitro groups can participate in redox reactions, affecting cellular oxidative processes .
Comparison with Similar Compounds
Structural Analogs
Key structural analogs include:
Physicochemical Properties
While explicit data for this compound are scarce, trends can be inferred:
- Acidity : Nitro groups lower pKa (e.g., 2,4-DNP has pKa ~4.0). The 4-fluoro substituent may further reduce pKa due to its electron-withdrawing nature.
- Solubility: Fluorine’s hydrophobicity likely decreases water solubility compared to non-fluorinated DNPs (e.g., 3,5-DNP).
- Thermal Stability : Fluorine may enhance stability relative to chloro- or methyl-substituted DNPs .
Biological Activity
4-Fluoro-3,5-dinitrophenol (FDNP) is a fluorinated derivative of dinitrophenol, a compound known for its role as a mitochondrial uncoupler. This article explores the biological activity of FDNP, focusing on its mechanisms of action, toxicity, and potential therapeutic applications.
FDNP is characterized by the presence of a fluorine atom and two nitro groups on a phenolic ring. The structural formula can be represented as:
This configuration influences its solubility, reactivity, and biological interactions.
The primary mechanism by which this compound exerts its effects is through mitochondrial uncoupling . This process disrupts the proton gradient across the mitochondrial membrane, leading to increased energy expenditure and heat production instead of ATP synthesis. The implications of this mechanism include:
- Increased Basal Metabolic Rate (BMR): FDNP has been shown to significantly elevate BMR due to enhanced uncoupling activity, which can lead to weight loss in certain contexts .
- Thermogenesis: The compound induces thermogenic responses in adipose tissues, making it a subject of interest for obesity research .
Toxicological Profile
Despite its potential benefits, FDNP's toxicity profile raises concerns. Key toxicological findings include:
- Acute Toxicity: High doses of dinitrophenols have been associated with severe hyperthermia, metabolic acidosis, and multi-organ failure .
- Carcinogenicity: Long-term exposure has been linked to carcinogenic effects in various studies, necessitating caution in therapeutic applications .
- Developmental Effects: Animal studies indicate that exposure during critical developmental periods can lead to teratogenic effects .
Case Study 1: Weight Loss Agent
In historical clinical trials, dinitrophenol was used as a weight-loss agent with significant effects observed:
- A study reported weight loss averaging 6.06 g over four days with accompanying hyperthermia (38.9°C) .
- Adverse effects included confusion, agitation, and potentially fatal outcomes in cases of overdose.
Case Study 2: Mitochondrial Uncoupling
Recent research highlights FDNP's role in mitochondrial uncoupling:
- A study demonstrated that compounds similar to FDNP could effectively reduce body fat in animal models by promoting thermogenesis through mitochondrial pathways .
Data Table: Biological Effects and Toxicity Profile
Q & A
Basic Research Questions
Q. What are the recommended methods for synthesizing 4-Fluoro-3,5-dinitrophenol, and how can reaction conditions be optimized?
- Methodology : Utilize nitration and fluorination protocols under controlled conditions. For nitration, employ mixed acid (HNO₃/H₂SO₄) at 0–5°C to minimize side reactions. Fluorination can be achieved via nucleophilic aromatic substitution (e.g., using KF in polar aprotic solvents like DMF) . Monitor reaction progress via TLC or HPLC, adjusting stoichiometry and temperature to optimize yield.
Q. How can researchers characterize the purity and structural integrity of this compound?
- Methodology : Combine spectroscopic techniques:
- ¹H/¹³C NMR to confirm substitution patterns and fluorine coupling.
- FT-IR for nitro (1520–1350 cm⁻¹) and phenolic O-H (3300–3500 cm⁻¹) stretches.
- Melting point analysis (mp 75–95°C for analogs ) to assess purity.
Cross-reference with computational predictions (e.g., Gaussian for IR/NMR simulations) .
Q. What safety protocols are critical when handling this compound in laboratory settings?
- Methodology : Follow guidelines for dinitrophenol derivatives:
- Use fume hoods and PPE (gloves, goggles) due to respiratory and dermal toxicity risks .
- Avoid high temperatures to prevent decomposition into toxic nitroso byproducts.
- Store in airtight containers away from reducing agents to minimize explosion hazards .
Q. How does the electron-withdrawing effect of fluorine influence the reactivity of this compound?
- Methodology : Perform comparative kinetic studies with non-fluorinated analogs (e.g., 3,5-dinitrophenol). Use UV-Vis spectroscopy to monitor deprotonation rates in varying pH buffers. Fluorine’s -I effect enhances phenolic acidity, accelerating nucleophilic substitution or coordination with metal ions .
Q. What analytical techniques are suitable for quantifying this compound in environmental samples?
- Methodology : Employ HPLC-UV/Vis with a C18 column and mobile phase (e.g., acetonitrile/water + 0.1% TFA). Validate via spike-and-recovery experiments in matrices like soil or water. Compare retention times with standards and confirm via MS/MS fragmentation .
Advanced Research Questions
Q. How can researchers resolve contradictions in reported spectral data (e.g., dipole moments, IR bands) for this compound and its analogs?
- Methodology : Replicate measurements under standardized conditions (solvent, concentration, temperature). Cross-validate using DFT calculations (e.g., B3LYP/6-311+G(d,p)) to predict dipole moments and vibrational modes. Discrepancies may arise from solvent effects or crystal packing .
Q. What strategies mitigate competing side reactions during multi-step synthesis of this compound derivatives?
- Methodology : Use protective groups (e.g., acetyl for -OH) before fluorination. Optimize stepwise nitration/fluorination sequences via DoE (Design of Experiments). Monitor intermediates with LC-MS and adjust catalysts (e.g., Cu-mediated fluorination) to suppress over-nitration .
Q. How does this compound interact with biological macromolecules, and what computational tools predict these interactions?
- Methodology : Conduct molecular docking (AutoDock Vina) to simulate binding with proteins/enzymes. Validate via SPR (Surface Plasmon Resonance) or ITC (Isothermal Titration Calorimetry). Fluorine’s electronegativity may enhance H-bonding or hydrophobic interactions .
Q. What role does this compound play in energy transfer systems, such as fluorescent probes or redox-active materials?
- Methodology : Synthesize metal complexes (e.g., with Ru or Ir) and study photophysical properties via fluorescence lifetime imaging (FLIM) . Compare quantum yields with non-fluorinated analogs. Nitro groups may quench fluorescence, but fluorine could stabilize excited states .
Q. How can researchers distinguish between structural isomers (e.g., 3,4- vs. 3,5-dinitrophenol derivatives) using advanced analytical techniques?
Properties
IUPAC Name |
4-fluoro-3,5-dinitrophenol | |
---|---|---|
Source | PubChem | |
URL | https://pubchem.ncbi.nlm.nih.gov | |
Description | Data deposited in or computed by PubChem | |
InChI |
InChI=1S/C6H3FN2O5/c7-6-4(8(11)12)1-3(10)2-5(6)9(13)14/h1-2,10H | |
Source | PubChem | |
URL | https://pubchem.ncbi.nlm.nih.gov | |
Description | Data deposited in or computed by PubChem | |
InChI Key |
AOHRUGRXVVCAEE-UHFFFAOYSA-N | |
Source | PubChem | |
URL | https://pubchem.ncbi.nlm.nih.gov | |
Description | Data deposited in or computed by PubChem | |
Canonical SMILES |
C1=C(C=C(C(=C1[N+](=O)[O-])F)[N+](=O)[O-])O | |
Source | PubChem | |
URL | https://pubchem.ncbi.nlm.nih.gov | |
Description | Data deposited in or computed by PubChem | |
Molecular Formula |
C6H3FN2O5 | |
Source | PubChem | |
URL | https://pubchem.ncbi.nlm.nih.gov | |
Description | Data deposited in or computed by PubChem | |
DSSTOX Substance ID |
DTXSID40565966 | |
Record name | 4-Fluoro-3,5-dinitrophenol | |
Source | EPA DSSTox | |
URL | https://comptox.epa.gov/dashboard/DTXSID40565966 | |
Description | DSSTox provides a high quality public chemistry resource for supporting improved predictive toxicology. | |
Molecular Weight |
202.10 g/mol | |
Source | PubChem | |
URL | https://pubchem.ncbi.nlm.nih.gov | |
Description | Data deposited in or computed by PubChem | |
CAS No. |
151222-64-9 | |
Record name | 4-Fluoro-3,5-dinitrophenol | |
Source | EPA DSSTox | |
URL | https://comptox.epa.gov/dashboard/DTXSID40565966 | |
Description | DSSTox provides a high quality public chemistry resource for supporting improved predictive toxicology. | |
Retrosynthesis Analysis
AI-Powered Synthesis Planning: Our tool employs the Template_relevance Pistachio, Template_relevance Bkms_metabolic, Template_relevance Pistachio_ringbreaker, Template_relevance Reaxys, Template_relevance Reaxys_biocatalysis model, leveraging a vast database of chemical reactions to predict feasible synthetic routes.
One-Step Synthesis Focus: Specifically designed for one-step synthesis, it provides concise and direct routes for your target compounds, streamlining the synthesis process.
Accurate Predictions: Utilizing the extensive PISTACHIO, BKMS_METABOLIC, PISTACHIO_RINGBREAKER, REAXYS, REAXYS_BIOCATALYSIS database, our tool offers high-accuracy predictions, reflecting the latest in chemical research and data.
Strategy Settings
Precursor scoring | Relevance Heuristic |
---|---|
Min. plausibility | 0.01 |
Model | Template_relevance |
Template Set | Pistachio/Bkms_metabolic/Pistachio_ringbreaker/Reaxys/Reaxys_biocatalysis |
Top-N result to add to graph | 6 |
Feasible Synthetic Routes
Disclaimer and Information on In-Vitro Research Products
Please be aware that all articles and product information presented on BenchChem are intended solely for informational purposes. The products available for purchase on BenchChem are specifically designed for in-vitro studies, which are conducted outside of living organisms. In-vitro studies, derived from the Latin term "in glass," involve experiments performed in controlled laboratory settings using cells or tissues. It is important to note that these products are not categorized as medicines or drugs, and they have not received approval from the FDA for the prevention, treatment, or cure of any medical condition, ailment, or disease. We must emphasize that any form of bodily introduction of these products into humans or animals is strictly prohibited by law. It is essential to adhere to these guidelines to ensure compliance with legal and ethical standards in research and experimentation.