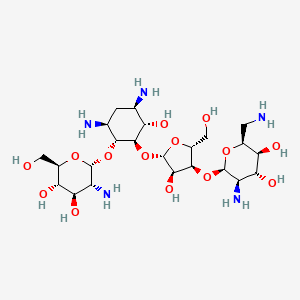
Paromomycin
Overview
Description
Paromomycin is an aminoglycoside antibiotic produced by Streptomyces rimosus var. paromomycinus . It is used to treat acute and chronic intestinal amebiasis, a bowel infection caused by a parasite in your stomach or bowels . It is also used with other medicines to help lessen the symptoms of hepatic coma, a complication of liver disease . Paromomycin is also used as an antimicrobial to treat a number of parasitic infections including amebiasis, giardiasis, leishmaniasis, and tapeworm infection .
Synthesis Analysis
Paromomycin is produced through solid-state fermentation using Streptomyces rimosus subsp. paromomycinus NRRL 2455 . Upon screening of 6 different substrates, maximum paromomycin concentration was obtained with the cost-effective agro-industrial byproduct, corn bran, impregnated with aminoglycoside production media .Molecular Structure Analysis
The IUPAC name of Paromomycin is (2S,3S,4R,5R,6R)-5-amino-2-(aminomethyl)-6-[(2R,3S,4R,5S)-5-[(1R,2R,3S,5R,6S)-3,5-diamino-2-[(2S,3R,4R,5S,6R)-3-amino-4,5-dihydroxy-6-(hydroxymethyl)oxan-2-yl]oxy-6-hydroxycyclohexyl]oxy-4-hydroxy-2-(hydroxymethyl)oxolan-3-yl]oxyoxane-3,4-diol . The molecular formula is C23H45N5O14 .Chemical Reactions Analysis
Paromomycin inhibits protein synthesis by binding to 16S ribosomal RNA . Bacterial proteins are synthesized by ribosomal RNA complexes which are composed of 2 subunits, a large subunit (50s) and small (30s) subunit, which forms a 70s ribosomal subunit .Physical And Chemical Properties Analysis
Paromomycin has 19 hydrogen bond acceptors, 13 hydrogen bond donors, 9 rotatable bonds, a topological polar surface area of 347.32, and a molecular weight of 615.3 .Scientific Research Applications
Mechanism of Action in Treating Leishmaniasis
Paromomycin is known for its efficacy in treating leishmaniasis. Research has demonstrated its impact on the cellular and molecular mechanisms of the Leishmania parasite. For instance, Maarouf et al. (1997) discovered that Paromomycin inhibits RNA synthesis in Leishmania donovani promastigotes, affecting membranous polar lipids and membrane fluidity, which leads to altered membrane permeability (Maarouf et al., 1997). Furthermore, the same study noted that Paromomycin disrupts mitochondrial activity in Leishmania donovani, affecting cellular energetics and metabolism (Maarouf et al., 1997).
Differential Effects on Ribosomes
A study by Fernández et al. (2010) demonstrated that Paromomycin differentially affects the translation processes in Leishmania mexicana and mammalian cells. This is due to its stronger binding to the parasite's ribosomal decoding site compared to its mammalian counterpart, explaining its therapeutic efficiency against leishmaniasis (Fernández et al., 2010).
Development of Paromomycin Resistance
Research by Maarouf et al. (1998) on the development of Paromomycin-resistant Leishmania donovani promastigotes revealed that resistance might be due to decreased drug uptake, likely resulting from altered membrane composition. This insight is crucial for understanding and addressing drug resistance in Leishmania (Maarouf et al., 1998).
Role in Other Parasitic Infections
Paromomycin's effectiveness extends beyond leishmaniasis. For example, Mancassola et al. (1995) explored its anticryptosporidial activity, finding that prophylactic use of Paromomycin in goat kids prevented Cryptosporidium parvum infection, suggesting its potential as an anticryptosporidial agent (Mancassola et al., 1995).
Immunological Impact
Das et al. (2014) investigated the immunomodulatory effects of Paromomycin, particularly in combination with Miltefosine for treating visceral leishmaniasis. Their findings highlighted the crucial role of Paromomycin in the maturation and activation of dendritic cells, which is essential for anti-leishmanial activity. This study emphasizes the importance of immunological aspects in the effectiveness of Paromomycin against leishmaniasis (Das et al., 2014).
Molecular Dynamics and Resistance Mechanisms
A molecular dynamics study by Romanowska et al. (2011) provided insights into bacterial resistance to aminoglycosides, including Paromomycin. By analyzing mutations in the bacterial ribosomal A-site, the study contributed to a better understanding of the physicochemical mechanisms behind bacterial resistance to aminoglycosides. This is crucial for developing strategies to overcome drug resistance (Romanowska et al., 2011).
Oral Bioavailability Enhancement
Pinjari et al. (2017) focused on improving the oral bioavailability of Paromomycin. The study explored various formulation approaches to enhance its absorption when taken orally. This research is significant in potentially making Paromomycin more accessible and convenient for patients, especially in areas where intramuscular injection is not feasible (Pinjari et al., 2017).
Phenotypic Suppression in Yeast
Paromomycin's ability to phenotypically suppress nonsense mutations in yeast was studied by Palmer et al. (1979). This research contributes to our understanding of how Paromomycin interacts with the genetic machinery of cells, providing a foundation for further exploration in genetic studies and potential applications in gene therapy (Palmer et al., 1979).
Inhibition of Cryptosporidium Infection
Marshall and Flanigan (1992) explored Paromomycin's efficacy in inhibiting Cryptosporidium parvum infection in a human enterocyte cell line. This research adds to the body of knowledge on the potential use of Paromomycin in treating enteritis, especially in immunocompromised patients, and highlights its broad-spectrum activity against various protozoan infections (Marshall & Flanigan, 1992).
Safety And Hazards
Future Directions
Topical Paromomycin with methylbenzethonium chloride (MBCL) could be a therapeutic alternative to pentavalent antimony compounds (SbV) in selected cases of the old world Cutaneous Leishmaniasis (CL) . Development of new formulations with better efficacy and tolerability remains to be an area of future research .
properties
IUPAC Name |
(2S,3S,4R,5R,6R)-5-amino-2-(aminomethyl)-6-[(2R,3S,4R,5S)-5-[(1R,2R,3S,5R,6S)-3,5-diamino-2-[(2S,3R,4R,5S,6R)-3-amino-4,5-dihydroxy-6-(hydroxymethyl)oxan-2-yl]oxy-6-hydroxycyclohexyl]oxy-4-hydroxy-2-(hydroxymethyl)oxolan-3-yl]oxyoxane-3,4-diol | |
---|---|---|
Source | PubChem | |
URL | https://pubchem.ncbi.nlm.nih.gov | |
Description | Data deposited in or computed by PubChem | |
InChI |
InChI=1S/C23H45N5O14/c24-2-7-13(32)15(34)10(27)21(37-7)41-19-9(4-30)39-23(17(19)36)42-20-12(31)5(25)1-6(26)18(20)40-22-11(28)16(35)14(33)8(3-29)38-22/h5-23,29-36H,1-4,24-28H2/t5-,6+,7+,8-,9-,10-,11-,12+,13-,14-,15-,16-,17-,18-,19-,20-,21-,22-,23+/m1/s1 | |
Source | PubChem | |
URL | https://pubchem.ncbi.nlm.nih.gov | |
Description | Data deposited in or computed by PubChem | |
InChI Key |
UOZODPSAJZTQNH-LSWIJEOBSA-N | |
Source | PubChem | |
URL | https://pubchem.ncbi.nlm.nih.gov | |
Description | Data deposited in or computed by PubChem | |
Canonical SMILES |
C1C(C(C(C(C1N)OC2C(C(C(C(O2)CO)O)O)N)OC3C(C(C(O3)CO)OC4C(C(C(C(O4)CN)O)O)N)O)O)N | |
Source | PubChem | |
URL | https://pubchem.ncbi.nlm.nih.gov | |
Description | Data deposited in or computed by PubChem | |
Isomeric SMILES |
C1[C@H]([C@@H]([C@H]([C@@H]([C@H]1N)O[C@@H]2[C@@H]([C@H]([C@@H]([C@H](O2)CO)O)O)N)O[C@H]3[C@@H]([C@@H]([C@H](O3)CO)O[C@@H]4[C@@H]([C@H]([C@@H]([C@@H](O4)CN)O)O)N)O)O)N | |
Source | PubChem | |
URL | https://pubchem.ncbi.nlm.nih.gov | |
Description | Data deposited in or computed by PubChem | |
Molecular Formula |
C23H45N5O14 | |
Source | PubChem | |
URL | https://pubchem.ncbi.nlm.nih.gov | |
Description | Data deposited in or computed by PubChem | |
Related CAS |
1263-89-4 (sulfate), 35665-49-7 (sulfate (2:5)), 7205-49-4 (sulfate (1:1)) | |
Record name | Paromomycin [INN:BAN] | |
Source | ChemIDplus | |
URL | https://pubchem.ncbi.nlm.nih.gov/substance/?source=chemidplus&sourceid=0007542372 | |
Description | ChemIDplus is a free, web search system that provides access to the structure and nomenclature authority files used for the identification of chemical substances cited in National Library of Medicine (NLM) databases, including the TOXNET system. | |
DSSTOX Substance ID |
DTXSID8023424 | |
Record name | Paromomycin | |
Source | EPA DSSTox | |
URL | https://comptox.epa.gov/dashboard/DTXSID8023424 | |
Description | DSSTox provides a high quality public chemistry resource for supporting improved predictive toxicology. | |
Molecular Weight |
615.6 g/mol | |
Source | PubChem | |
URL | https://pubchem.ncbi.nlm.nih.gov | |
Description | Data deposited in or computed by PubChem | |
Physical Description |
Solid | |
Record name | Paromomycin | |
Source | Human Metabolome Database (HMDB) | |
URL | http://www.hmdb.ca/metabolites/HMDB0015490 | |
Description | The Human Metabolome Database (HMDB) is a freely available electronic database containing detailed information about small molecule metabolites found in the human body. | |
Explanation | HMDB is offered to the public as a freely available resource. Use and re-distribution of the data, in whole or in part, for commercial purposes requires explicit permission of the authors and explicit acknowledgment of the source material (HMDB) and the original publication (see the HMDB citing page). We ask that users who download significant portions of the database cite the HMDB paper in any resulting publications. | |
Solubility |
7.97e+01 g/L | |
Record name | Paromomycin | |
Source | Human Metabolome Database (HMDB) | |
URL | http://www.hmdb.ca/metabolites/HMDB0015490 | |
Description | The Human Metabolome Database (HMDB) is a freely available electronic database containing detailed information about small molecule metabolites found in the human body. | |
Explanation | HMDB is offered to the public as a freely available resource. Use and re-distribution of the data, in whole or in part, for commercial purposes requires explicit permission of the authors and explicit acknowledgment of the source material (HMDB) and the original publication (see the HMDB citing page). We ask that users who download significant portions of the database cite the HMDB paper in any resulting publications. | |
Mechanism of Action |
Paromomycin inhibits protein synthesis by binding to 16S ribosomal RNA. Bacterial proteins are synthesized by ribosomal RNA complexes which are composed of 2 subunits, a large subunit (50s) and small (30s) subunit, which forms a 70s ribosomal subunit. tRNA binds to the top of this ribosomal structure. Paramomycin binds to the A site, which causes defective polypeptide chains to be produced. Continuous production of defective proteins eventually leads to bacterial death. | |
Record name | Paromomycin | |
Source | DrugBank | |
URL | https://www.drugbank.ca/drugs/DB01421 | |
Description | The DrugBank database is a unique bioinformatics and cheminformatics resource that combines detailed drug (i.e. chemical, pharmacological and pharmaceutical) data with comprehensive drug target (i.e. sequence, structure, and pathway) information. | |
Explanation | Creative Common's Attribution-NonCommercial 4.0 International License (http://creativecommons.org/licenses/by-nc/4.0/legalcode) | |
Product Name |
Paromomycin | |
CAS RN |
7542-37-2, 1263-89-4 | |
Record name | Paromomycin | |
Source | CAS Common Chemistry | |
URL | https://commonchemistry.cas.org/detail?cas_rn=7542-37-2 | |
Description | CAS Common Chemistry is an open community resource for accessing chemical information. Nearly 500,000 chemical substances from CAS REGISTRY cover areas of community interest, including common and frequently regulated chemicals, and those relevant to high school and undergraduate chemistry classes. This chemical information, curated by our expert scientists, is provided in alignment with our mission as a division of the American Chemical Society. | |
Explanation | The data from CAS Common Chemistry is provided under a CC-BY-NC 4.0 license, unless otherwise stated. | |
Record name | Paromomycin [INN:BAN] | |
Source | ChemIDplus | |
URL | https://pubchem.ncbi.nlm.nih.gov/substance/?source=chemidplus&sourceid=0007542372 | |
Description | ChemIDplus is a free, web search system that provides access to the structure and nomenclature authority files used for the identification of chemical substances cited in National Library of Medicine (NLM) databases, including the TOXNET system. | |
Record name | Paromomycin | |
Source | DrugBank | |
URL | https://www.drugbank.ca/drugs/DB01421 | |
Description | The DrugBank database is a unique bioinformatics and cheminformatics resource that combines detailed drug (i.e. chemical, pharmacological and pharmaceutical) data with comprehensive drug target (i.e. sequence, structure, and pathway) information. | |
Explanation | Creative Common's Attribution-NonCommercial 4.0 International License (http://creativecommons.org/licenses/by-nc/4.0/legalcode) | |
Record name | Paromomycin | |
Source | EPA DSSTox | |
URL | https://comptox.epa.gov/dashboard/DTXSID8023424 | |
Description | DSSTox provides a high quality public chemistry resource for supporting improved predictive toxicology. | |
Record name | Paromomycin | |
Source | European Chemicals Agency (ECHA) | |
URL | https://echa.europa.eu/substance-information/-/substanceinfo/100.028.567 | |
Description | The European Chemicals Agency (ECHA) is an agency of the European Union which is the driving force among regulatory authorities in implementing the EU's groundbreaking chemicals legislation for the benefit of human health and the environment as well as for innovation and competitiveness. | |
Explanation | Use of the information, documents and data from the ECHA website is subject to the terms and conditions of this Legal Notice, and subject to other binding limitations provided for under applicable law, the information, documents and data made available on the ECHA website may be reproduced, distributed and/or used, totally or in part, for non-commercial purposes provided that ECHA is acknowledged as the source: "Source: European Chemicals Agency, http://echa.europa.eu/". Such acknowledgement must be included in each copy of the material. ECHA permits and encourages organisations and individuals to create links to the ECHA website under the following cumulative conditions: Links can only be made to webpages that provide a link to the Legal Notice page. | |
Record name | PAROMOMYCIN | |
Source | FDA Global Substance Registration System (GSRS) | |
URL | https://gsrs.ncats.nih.gov/ginas/app/beta/substances/61JJC8N5ZK | |
Description | The FDA Global Substance Registration System (GSRS) enables the efficient and accurate exchange of information on what substances are in regulated products. Instead of relying on names, which vary across regulatory domains, countries, and regions, the GSRS knowledge base makes it possible for substances to be defined by standardized, scientific descriptions. | |
Explanation | Unless otherwise noted, the contents of the FDA website (www.fda.gov), both text and graphics, are not copyrighted. They are in the public domain and may be republished, reprinted and otherwise used freely by anyone without the need to obtain permission from FDA. Credit to the U.S. Food and Drug Administration as the source is appreciated but not required. | |
Record name | Paromomycin | |
Source | Human Metabolome Database (HMDB) | |
URL | http://www.hmdb.ca/metabolites/HMDB0015490 | |
Description | The Human Metabolome Database (HMDB) is a freely available electronic database containing detailed information about small molecule metabolites found in the human body. | |
Explanation | HMDB is offered to the public as a freely available resource. Use and re-distribution of the data, in whole or in part, for commercial purposes requires explicit permission of the authors and explicit acknowledgment of the source material (HMDB) and the original publication (see the HMDB citing page). We ask that users who download significant portions of the database cite the HMDB paper in any resulting publications. | |
Citations
Disclaimer and Information on In-Vitro Research Products
Please be aware that all articles and product information presented on BenchChem are intended solely for informational purposes. The products available for purchase on BenchChem are specifically designed for in-vitro studies, which are conducted outside of living organisms. In-vitro studies, derived from the Latin term "in glass," involve experiments performed in controlled laboratory settings using cells or tissues. It is important to note that these products are not categorized as medicines or drugs, and they have not received approval from the FDA for the prevention, treatment, or cure of any medical condition, ailment, or disease. We must emphasize that any form of bodily introduction of these products into humans or animals is strictly prohibited by law. It is essential to adhere to these guidelines to ensure compliance with legal and ethical standards in research and experimentation.