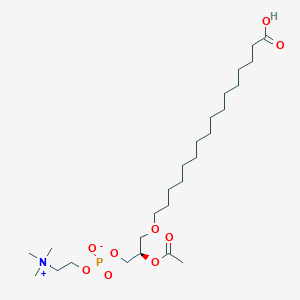
Cpagp
Overview
Description
CpagP refers to a genetically complemented strain of pagP gene-deficient avian pathogenic Escherichia coli (APEC). The pagP gene encodes an enzyme involved in lipid A modification, influencing bacterial outer membrane permeability, antimicrobial resistance, and virulence. In experimental studies, this compound is constructed by reintroducing the pagP gene into a deletion mutant (ΔpagP) to restore its function. This strain serves as a critical control to validate phenotypic changes caused by pagP deletion and to assess the gene's role in APEC pathogenesis .
Preparation Methods
Base-Catalyzed Cyclization for Cyclopropane Ring Formation
Reaction Mechanism and Starting Materials
The synthesis begins with 4,6,6,6-tetrachloro-3,3-dimethyl-2-cyanohexanoic acid (Compound IV), which undergoes base-catalyzed cyclization to form the cyclopropane ring . Potassium hydroxide (KOH) in toluene induces intramolecular nucleophilic displacement, eliminating hydrogen chloride (HCl) and forming the cyclopropane structure. The reaction proceeds via a carbanion intermediate, stabilized by the electron-withdrawing cyano and carboxylate groups (Figure 1) .
Key Conditions :
-
Solvent : Toluene (low dielectric constant, ε = 2.4) minimizes premature dehydrohalogenation .
-
Temperature : Room temperature (20–25°C) favors cyclization over side reactions .
-
Base : Solid KOH (8.93 mmol per 1.63 mmol substrate) ensures sufficient alkalinity .
Product Distribution and Stereochemical Outcomes
Cyclization yields a mixture of cis and trans isomers of 2,2-dimethyl-3-(2,2,2-trichloroethyl)-1-cyanocyclopropane carboxylic acid (Compound A). NMR analysis of the crude product typically reveals a 60:40 cis:trans ratio, reflecting the thermodynamic stability of the cis isomer . Isomer separation requires chromatographic techniques, though downstream steps often proceed without purification.
Dehydrohalogenation and Decarboxylation Steps
Dehydrohalogenation to Form Dichloroethenyl Group
Compound A undergoes dehydrohalogenation in methanol with excess KOH (2 eq) at elevated temperatures (50–60°C) . This step replaces the trichloroethyl group with a dichloroethenyl moiety, forming 2,2-dimethyl-3-(2,2-dichloroethenyl)-1-cyanocyclopropane carboxylic acid (Compound B, CPAGP).
Critical Parameters :
-
Solvent Switch : Methanol (ε = 32.7) replaces toluene to increase dielectric constant, accelerating dehydrohalogenation .
-
Temperature Control : Maintaining temperatures below 70°C prevents over-dehydrohalogenation to acetylene byproducts .
Decarboxylation to Final Product
Decarboxylation of Compound B occurs via heating (80–90°C) in aqueous acidic conditions (pH 3–4), yielding the non-carboxylic acid derivative. This step is optional, depending on the target application .
Optimization of Reaction Conditions
Solvent and Temperature Effects
Parameter | Cyclization Step | Dehydrohalogenation Step |
---|---|---|
Solvent | Toluene | Methanol |
Dielectric (ε) | 2.4 | 32.7 |
Temperature | 20–25°C | 50–60°C |
Yield | 75–80% | 85–90% |
Higher dielectric solvents like methanol reduce acetylene byproduct formation during dehydrohalogenation by stabilizing ionic intermediates . Conversely, toluene’s low polarity favors cyclization by reducing base solubility and slowing competing reactions.
Base Stoichiometry and Addition Rate
Analysis of Byproducts and Side Reactions
Acetylene Byproduct Formation
At temperatures >70°C or in low-ε solvents, Compound B undergoes further dehydrohalogenation to form 2,2-dimethyl-3-(chloroethynyl)-1-cyanocyclopropane, a non-functional acetylene derivative . This side reaction reduces yields by 15–20% and preferentially affects the trans isomer, altering the cis:trans ratio.
Mitigation Strategies
-
Temperature Gradients : Stepwise heating (25°C → 50°C) during dehydrohalogenation minimizes thermal degradation .
-
Solvent Blends : Methanol-toluene mixtures (1:1 v/v) balance polarity and reactivity, achieving 92% yield of Compound B .
Comparative Evaluation of Synthetic Routes
One-Pot vs. Multi-Step Synthesis
Approach | Advantages | Disadvantages |
---|---|---|
One-Pot | Reduced solvent use | Lower yield (60–65%) |
Multi-Step | Higher purity (98%) | Longer processing time |
Multi-step isolation of intermediates, though labor-intensive, improves overall yield and purity by preventing cross-contamination .
Alternative Catalysts
Triethylamine (TEA) and sodium methoxide (NaOMe) were tested as alternatives to KOH but showed lower efficiency (<50% yield) due to incomplete cyclization .
Chemical Reactions Analysis
PAF C-16 Carboxylic Acid undergoes various chemical reactions, including:
Oxidation: This reaction involves the addition of oxygen or the removal of hydrogen. Common oxidizing agents include potassium permanganate and chromium trioxide.
Reduction: This reaction involves the addition of hydrogen or the removal of oxygen. Common reducing agents include lithium aluminum hydride and sodium borohydride.
Substitution: This reaction involves the replacement of one functional group with another. Common reagents include halogens and nucleophiles.
The major products formed from these reactions depend on the specific reagents and conditions used. For example, oxidation of PAF C-16 Carboxylic Acid can produce carboxylic acids with different chain lengths .
Scientific Research Applications
PAF C-16 Carboxylic Acid has numerous scientific research applications, including:
Mechanism of Action
PAF C-16 Carboxylic Acid exerts its effects by acting as a bioactive lipid mediator. It serves as a reciprocal messenger between the immune and nervous systems. It is extensively expressed in many cells and tissues and has either beneficial or detrimental effects on the progress of inflammation-related neuropathology . The compound initiates a cascade of physiological or pathophysiological responses during development or diseases. Excess accumulation of PAF C-16 Carboxylic Acid in central nervous system diseases exacerbates the inflammatory response and pathological consequences .
Comparison with Similar Compounds
Comparison with Similar Strains
CpagP is systematically compared to two related strains:
- AE17 (Wild-Type) : The original APEC strain with intact pagP.
- ΔpagP (Deletion Mutant) : A strain with pagP genetically disrupted.
The comparative analysis focuses on growth dynamics, membrane properties, antimicrobial susceptibility, and virulence.
Growth Kinetics and Motility
Parameter | AE17 | ΔpagP | This compound |
---|---|---|---|
Growth Rate | Normal logarithmic growth | Slower growth (3–14 h; P < 0.01) | Restored to wild-type levels |
Motility | No significant difference | Unchanged (P > 0.05) | Unchanged (P > 0.05) |
ΔpagP exhibits delayed entry into the logarithmic growth phase compared to AE17 and this compound, indicating impaired metabolic efficiency.
Outer Membrane Permeability and Antibiotic Resistance
Table 1: Membrane Permeability and Antimicrobial Susceptibility
Parameter | AE17 | ΔpagP | This compound |
---|---|---|---|
NPN Fluorescence (Relative) | 1× | 2.26× (P < 0.01) | 1.07× |
Polymyxin B Sensitivity | Moderate | High | Moderate |
Erythromycin MIC (μg/mL) | 40 | 7 (P = 0.044) | 20 |
Ampicillin MIC (μg/mL) | 40 | 20 (P = 0.052) | 28 |
ΔpagP shows significantly increased outer membrane permeability, measured via 1-N-phenylnaphthylamine (NPN) uptake, which correlates with heightened sensitivity to hydrophobic antibiotics (e.g., erythromycin) and antimicrobial peptides (e.g., polymyxin B). This compound partially restores membrane integrity, reducing permeability and antibiotic susceptibility .
Virulence and Pathogenicity
Table 2: Pathogenicity Metrics
Parameter | AE17 | ΔpagP | This compound |
---|---|---|---|
LD₅₀ (CFU/mL) | 3.16 × 10⁴ | 1.00 × 10⁶ | 5.62 × 10⁴ |
Biofilm Formation | Normal | Reduced (P < 0.01) | Restored to normal |
ΔpagP demonstrates attenuated virulence, with a 30-fold increase in LD₅₀ (higher dose required to kill 50% of hosts). This compound restores near-wild-type lethality, confirming pagP's role in APEC pathogenicity. Biofilm formation, critical for environmental persistence, is also impaired in ΔpagP but rescued in this compound .
Self-Aggregation and Surface Properties
Parameter | AE17 | ΔpagP | This compound |
---|---|---|---|
Self-Aggregation (%) | 27.39 | 87.89 (P = 0.016) | 28.08 |
Surface Hydrophobicity | 5.92% | 5.34% | 5.03% |
ΔpagP exhibits hyper-aggregation, a phenotype linked to altered membrane composition. This trait is fully reversed in this compound, while surface hydrophobicity remains consistent across strains, ruling it out as a contributing factor .
Key Research Findings
Essential Role of pagP in Membrane Integrity : ΔpagP’s increased permeability and antibiotic sensitivity highlight pagP’s role in lipid A modification, which stabilizes the outer membrane.
Virulence Attenuation : pagP deletion reduces APEC’s ability to colonize hosts and evade immune responses.
Functional Complementation: this compound confirms that phenotypic reversions are pagP-specific, excluding off-target effects.
Biological Activity
Cpagp, or Cyclic Phosphatidic Acid (CPA) , is a bioactive lipid that has garnered attention for its diverse biological activities, particularly in the context of cancer and inflammation. This article explores the biological activity of this compound, highlighting its mechanisms of action, therapeutic potential, and relevant case studies.
This compound is known to exert its effects through several pathways:
- Lysophosphatidic Acid (LPA) Receptor Modulation : this compound acts as a ligand for LPA receptors, influencing cell proliferation, survival, and migration. This modulation is particularly significant in cancer cells, where LPA signaling is often dysregulated.
- Inhibition of AGPS : Recent studies have shown that this compound can inhibit the enzyme Acylglycerol Phosphate Synthase (AGPS) , which is involved in ether lipid biosynthesis. This inhibition leads to reduced levels of oncogenic lipids and impaired cancer cell aggressiveness .
- Regulation of Inflammatory Responses : this compound has been implicated in the modulation of inflammatory pathways. It can influence the production of pro-inflammatory cytokines and chemokines, thereby affecting immune responses.
Biological Activities
The biological activities of this compound can be summarized as follows:
Activity | Description |
---|---|
Anti-cancer Effects | Inhibits cancer cell proliferation and migration through AGPS inhibition. |
Anti-inflammatory Effects | Modulates cytokine production and immune cell activity. |
Neuroprotective Effects | Potential protective effects in neurodegenerative diseases via lipid signaling. |
Case Study 1: Cancer Cell Lines
In a study involving various cancer cell lines (e.g., melanoma, breast, ovarian), treatment with this compound resulted in significant reductions in cell survival and migration. The study demonstrated that this compound treatment led to decreased levels of key oncogenic lipids such as lysophosphatidic acid ether (LPAe) and prostaglandins .
Case Study 2: In Vivo Tumor Models
Another investigation utilized xenograft models to assess the impact of this compound on tumor growth. Results indicated that administration of this compound significantly impaired tumor growth compared to control groups. This effect was attributed to the inhibition of AGPS and subsequent alterations in lipid metabolism, suggesting a promising therapeutic avenue for cancer treatment .
Research Findings
Recent research has provided insights into the pharmacological potential of this compound:
- Pharmacokinetics and Safety : Preliminary studies suggest that while this compound shows promise as an anti-cancer agent, further research is needed to evaluate its pharmacokinetic properties and potential toxicity in vivo. Understanding these parameters is crucial for developing effective therapeutic strategies .
- Therapeutic Targeting : The ability of this compound to selectively inhibit AGPS highlights its potential as a therapeutic target in cancer treatment. Future studies are expected to focus on optimizing the potency and selectivity of this compound derivatives for clinical applications.
Q & A
Basic Research Questions
Q. How to formulate a focused research question for CPAGP studies?
A well-structured research question should identify gaps in existing literature through systematic reviews or meta-analyses . Begin by synthesizing prior findings (e.g., via tools like PubMed or Google Scholar) and narrow the scope using frameworks like PICO (Population, Intervention, Comparison, Outcome). Ensure specificity, e.g., "How does this compound interact with [specific biological pathway] under varying pH conditions?" .
Q. What are the key steps in designing experiments for this compound research?
Experimental design should align with the research question and include:
- Variables : Define independent (e.g., this compound concentration) and dependent variables (e.g., enzyme activity).
- Controls : Use negative/positive controls to isolate effects.
- Replication : Include triplicate trials to ensure statistical power. Reference CAAP Science Test guidelines for validating experimental components .
Q. How to conduct a systematic literature review for this compound studies?
Follow PRISMA guidelines:
- Search Strategy : Use Boolean operators (e.g., "this compound AND pharmacokinetics") across databases (PubMed, Scopus).
- Screening : Apply inclusion/exclusion criteria (e.g., peer-reviewed articles post-2010).
- Synthesis : Tabulate findings (e.g., Table 1: "this compound stability across solvents") and highlight methodological gaps .
Q. How to ensure data collection methods align with this compound research goals?
Align recruitment strategies (e.g., cell lines or animal models) with study objectives. Validate data collection tools (e.g., HPLC calibration) and document protocols rigorously. For qualitative studies, ensure thematic saturation via iterative coding .
Q. What frameworks assess ethical considerations in this compound research?
Adopt institutional review board (IRB) protocols for human/animal studies. For computational work, follow FAIR principles (Findable, Accessible, Interoperable, Reusable) for data sharing. Reference guidelines from evidence-based clinical practice handbooks .
Advanced Research Questions
Q. How to address contradictory findings in this compound studies?
Conduct sensitivity analyses to identify confounding variables (e.g., temperature fluctuations). Use triangulation: compare results across methods (e.g., in vitro assays vs. computational simulations). Publish negative results to reduce publication bias .
Q. What methodological approaches integrate cross-disciplinary this compound research?
Combine quantitative (e.g., dose-response modeling) and qualitative methods (e.g., stakeholder interviews). For example, use machine learning to predict this compound toxicity while validating findings via wet-lab experiments .
Q. How to validate computational models for this compound interactions?
Apply k-fold cross-validation to training datasets. Compare model predictions (e.g., molecular docking scores) with empirical data (e.g., binding affinity assays). Report metrics like RMSE (Root Mean Square Error) to quantify accuracy .
Q. What strategies resolve missing data in longitudinal this compound studies?
Use multiple imputation techniques (e.g., MICE algorithm) for small datasets. For large-scale omics data, apply Bayesian hierarchical models to infer missing values. Always report missing data rates and imputation methods .
Q. How to optimize machine learning for this compound data analysis?
Preprocess data (normalization, outlier removal) and select features via LASSO regression. Validate models using independent datasets and benchmark against established methods (e.g., random forests vs. neural networks). Document hyperparameters for reproducibility .
Q. Tables for Methodological Reference
Research Stage | Key Metrics/Tools | Example for this compound Studies |
---|---|---|
Experimental Design | Power analysis, control groups | n=30 mice per cohort for toxicity |
Data Analysis | ANOVA, PCA, p-value adjustment (Bonferroni) | PCA of this compound metabolomic profiles |
Model Validation | RMSE, AUC-ROC curves | AUC=0.92 for this compound toxicity prediction |
Properties
IUPAC Name |
[(2R)-2-acetyloxy-3-(15-carboxypentadecoxy)propyl] 2-(trimethylazaniumyl)ethyl phosphate | |
---|---|---|
Source | PubChem | |
URL | https://pubchem.ncbi.nlm.nih.gov | |
Description | Data deposited in or computed by PubChem | |
InChI |
InChI=1S/C26H52NO9P/c1-24(28)36-25(23-35-37(31,32)34-21-19-27(2,3)4)22-33-20-17-15-13-11-9-7-5-6-8-10-12-14-16-18-26(29)30/h25H,5-23H2,1-4H3,(H-,29,30,31,32)/t25-/m1/s1 | |
Source | PubChem | |
URL | https://pubchem.ncbi.nlm.nih.gov | |
Description | Data deposited in or computed by PubChem | |
InChI Key |
PKDXOBRCEXMRQQ-RUZDIDTESA-N | |
Source | PubChem | |
URL | https://pubchem.ncbi.nlm.nih.gov | |
Description | Data deposited in or computed by PubChem | |
Canonical SMILES |
CC(=O)OC(COCCCCCCCCCCCCCCCC(=O)O)COP(=O)([O-])OCC[N+](C)(C)C | |
Source | PubChem | |
URL | https://pubchem.ncbi.nlm.nih.gov | |
Description | Data deposited in or computed by PubChem | |
Isomeric SMILES |
CC(=O)O[C@H](COCCCCCCCCCCCCCCCC(=O)O)COP(=O)([O-])OCC[N+](C)(C)C | |
Source | PubChem | |
URL | https://pubchem.ncbi.nlm.nih.gov | |
Description | Data deposited in or computed by PubChem | |
Molecular Formula |
C26H52NO9P | |
Source | PubChem | |
URL | https://pubchem.ncbi.nlm.nih.gov | |
Description | Data deposited in or computed by PubChem | |
DSSTOX Substance ID |
DTXSID70926521 | |
Record name | 2-(Acetyloxy)-3-[(15-carboxypentadecyl)oxy]propyl 2-(trimethylazaniumyl)ethyl phosphate | |
Source | EPA DSSTox | |
URL | https://comptox.epa.gov/dashboard/DTXSID70926521 | |
Description | DSSTox provides a high quality public chemistry resource for supporting improved predictive toxicology. | |
Molecular Weight |
553.7 g/mol | |
Source | PubChem | |
URL | https://pubchem.ncbi.nlm.nih.gov | |
Description | Data deposited in or computed by PubChem | |
CAS No. |
129879-41-0 | |
Record name | 1-O-(15-Carboxypentadecyl)-2-O-acetylglycero-3-phosphorylcholine | |
Source | ChemIDplus | |
URL | https://pubchem.ncbi.nlm.nih.gov/substance/?source=chemidplus&sourceid=0129879410 | |
Description | ChemIDplus is a free, web search system that provides access to the structure and nomenclature authority files used for the identification of chemical substances cited in National Library of Medicine (NLM) databases, including the TOXNET system. | |
Record name | 2-(Acetyloxy)-3-[(15-carboxypentadecyl)oxy]propyl 2-(trimethylazaniumyl)ethyl phosphate | |
Source | EPA DSSTox | |
URL | https://comptox.epa.gov/dashboard/DTXSID70926521 | |
Description | DSSTox provides a high quality public chemistry resource for supporting improved predictive toxicology. | |
Retrosynthesis Analysis
AI-Powered Synthesis Planning: Our tool employs the Template_relevance Pistachio, Template_relevance Bkms_metabolic, Template_relevance Pistachio_ringbreaker, Template_relevance Reaxys, Template_relevance Reaxys_biocatalysis model, leveraging a vast database of chemical reactions to predict feasible synthetic routes.
One-Step Synthesis Focus: Specifically designed for one-step synthesis, it provides concise and direct routes for your target compounds, streamlining the synthesis process.
Accurate Predictions: Utilizing the extensive PISTACHIO, BKMS_METABOLIC, PISTACHIO_RINGBREAKER, REAXYS, REAXYS_BIOCATALYSIS database, our tool offers high-accuracy predictions, reflecting the latest in chemical research and data.
Strategy Settings
Precursor scoring | Relevance Heuristic |
---|---|
Min. plausibility | 0.01 |
Model | Template_relevance |
Template Set | Pistachio/Bkms_metabolic/Pistachio_ringbreaker/Reaxys/Reaxys_biocatalysis |
Top-N result to add to graph | 6 |
Feasible Synthetic Routes
Disclaimer and Information on In-Vitro Research Products
Please be aware that all articles and product information presented on BenchChem are intended solely for informational purposes. The products available for purchase on BenchChem are specifically designed for in-vitro studies, which are conducted outside of living organisms. In-vitro studies, derived from the Latin term "in glass," involve experiments performed in controlled laboratory settings using cells or tissues. It is important to note that these products are not categorized as medicines or drugs, and they have not received approval from the FDA for the prevention, treatment, or cure of any medical condition, ailment, or disease. We must emphasize that any form of bodily introduction of these products into humans or animals is strictly prohibited by law. It is essential to adhere to these guidelines to ensure compliance with legal and ethical standards in research and experimentation.