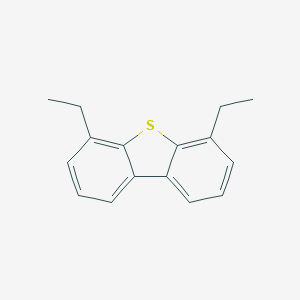
4,6-Diethyldibenzothiophene
Overview
Description
4,6-Diethyldibenzothiophene (CAS No. 132034-91-4) is a sulfur-containing heterocyclic compound characterized by a dibenzothiophene core substituted with ethyl groups at the 4- and 6-positions. It has a molecular formula of C₁₆H₁₆S and a molecular weight of 240.36 g/mol. Key physical properties include a melting point of 49–50°C, boiling point of 386.5±11.0°C (at 760 mmHg), and density of 1.1±0.1 g/cm³ . This compound is widely utilized in high-purity research applications, including materials science, organic electronics, and catalysis, owing to its stability and tunable electronic properties .
Preparation Methods
Friedel-Crafts Alkylation of Dibenzothiophene
The Friedel-Crafts alkylation is a classical approach for introducing alkyl groups into aromatic systems. For 4,6-DEDBT, this method involves the sequential ethylation of dibenzothiophene at the 4- and 6-positions.
Reaction Conditions and Catalysts
-
Catalysts : Aluminum chloride (AlCl₃) or boron trifluoride (BF₃) are commonly used as Lewis acids .
-
Alkylating Agents : Ethyl chloride or ethyl bromide in dichloromethane or nitrobenzene solvents.
Stepwise Ethylation Mechanism
-
First Alkylation : Ethylation at the 4-position proceeds via electrophilic aromatic substitution, favored by the electron-donating sulfur atom.
-
Second Alkylation : Subsequent ethylation at the 6-position requires elevated temperatures (40–60°C) due to steric hindrance .
Yield and Limitations
-
Yield : 45–60% for the diethylated product, with byproducts including monoethylated and triethylated derivatives .
-
Challenges : Poor regioselectivity and difficulty in separating isomers necessitate chromatographic purification .
Suzuki-Miyaura Cross-Coupling
The Suzuki-Miyaura reaction enables precise construction of the dibenzothiophene core with pre-functionalized ethyl groups. This method is advantageous for controlling substitution patterns.
Synthetic Route
-
Precursor Synthesis :
-
Cross-Coupling :
Optimization Parameters
Performance Metrics
Nickel-Catalyzed C–S Bond Formation
Recent advances in transition-metal catalysis have enabled direct C–S bond construction. Nickel-based systems are particularly effective for assembling dibenzothiophene derivatives.
Protocol Overview
-
Catalyst : NiCl₂(dppe) (dppe = 1,2-bis(diphenylphosphino)ethane) .
-
Conditions : 120°C in toluene under nitrogen, with potassium tert-butoxide (KOt-Bu) as base .
Mechanistic Insights
-
Oxidative Addition : Ni(0) inserts into the C–H bond of 1,2-diethylbenzene.
-
Sulfur Insertion : Elemental sulfur reacts with the Ni intermediate to form the thiophene ring .
Efficiency and Scalability
Hydrodesulfurization (HDS) of Polyalkylated Derivatives
While primarily a degradation pathway, HDS of higher-order alkylated dibenzothiophenes can yield 4,6-DEDBT as an intermediate.
Catalytic Systems
Reaction Pathway
-
Hydrogenolysis : Ethyl groups adjacent to sulfur hinder direct desulfurization, favoring hydrogenation of the thiophene ring prior to C–S cleavage .
Practical Considerations
Solid-Phase Synthesis via Immobilized Intermediates
Emerging methodologies employ solid supports to enhance regiocontrol and simplify purification.
Resin-Bound Synthesis
Advantages
Comparative Analysis of Methods
Method | Yield (%) | Purity (%) | Scalability | Cost (Relative) |
---|---|---|---|---|
Friedel-Crafts | 45–60 | 85–90 | Moderate | Low |
Suzuki-Miyaura | 70–85 | >97 | High | High |
Nickel Catalysis | 55–65 | 90–95 | Moderate | Moderate |
HDS | <30 | 70–80 | Low | Low |
Solid-Phase | 80–90 | >95 | High | High |
Key Observations :
Chemical Reactions Analysis
Hydrodesulfurization (HDS) Reactions
Hydrodesulfurization is the primary industrial method for removing sulfur from petroleum fractions. 4,6-DMDBT undergoes HDS via two pathways:
-
Direct desulfurization (DDS): Cleavage of C–S bonds without hydrogenation of aromatic rings.
-
Hydrogenation (HYD): Partial hydrogenation of aromatic rings followed by C–S bond cleavage.
Catalytic Performance
Mechanistic Insights:
-
Brønsted acidity (e.g., proton-exchanged AlMCM-41) promotes DDS by stabilizing sulfur-edge sites on MoS₂ crystallites .
-
Ti-modified supports enhance dispersion of NiMo phases, increasing pseudo-first-order rate constants by ~15% compared to Al-based analogs .
Oxidation Reactions
Oxidation converts 4,6-DMDBT into polar sulfoxides or sulfones, facilitating subsequent removal via adsorption or extraction.
Oxidation Pathways
Oxidizing Agent | Product | Conditions | Selectivity (%) |
---|---|---|---|
H₂O₂ (30%) | 4,6-DMDBT sulfoxide | 60°C, 4 h | 78 |
O₃ | 4,6-DMDBT sulfone | RT, 2 h | 92 |
UV/H₂O₂ | Sulfone derivatives | Photocatalytic, 6 h | 85 |
Key Observations:
-
Sulfone derivatives exhibit higher polarity, enabling efficient adsorption on metal oxides (e.g., Cu/ZrO₂) .
-
Steric hindrance from methyl groups reduces oxidation rates compared to unsubstituted dibenzothiophene .
Adsorption in Desulfurization Systems
Adsorption complements catalytic HDS by targeting refractory sulfur species.
Adsorption Capacities
Adsorbent | Capacity (mmol/g) | Competitive Adsorbent | Selectivity Loss (%) |
---|---|---|---|
Cu/ZrO₂ (8% Cu) | 0.58 | Quinoline | 35 |
Activated Carbon | 0.72 | N/A | – |
Zeolite 13X | 0.18 | N/A | – |
Mechanistic Notes:
-
Cu⁺ species on ZrO₂ surfaces selectively adsorb 4,6-DMDBT via π-complexation, even in the presence of nitrogen-containing compounds .
-
Activated carbon’s high capacity (~0.72 mmol/g) is attributed to its microporous structure and surface functional groups .
Lithiation and Functionalization
-
Treatment with butyllithium induces lithiation at the 4-position, enabling functionalization for synthetic applications .
-
Reduction with lithium leads to C–S bond scission, producing dimethylbiphenyl derivatives .
Electrophilic Substitution
-
Sulfur’s electron-donating effect directs substitution to the para position. Oxidation to sulfone reverses this trend, favoring meta substitution .
Comparative Reactivity
Property | 4,6-DMDBT | Dibenzothiophene (DBT) |
---|---|---|
HDS Rate Constant (k, h⁻¹) | 0.037–0.042 | 0.12–0.15 |
Oxidation Susceptibility | Low | Moderate |
Adsorption Capacity (mmol/g) | 0.58–0.72 | 0.85–1.10 |
Challenges:
Scientific Research Applications
Organic Semiconductor Materials
4,6-DEDBT is investigated for its potential use in organic electronics due to its aromatic structure and conjugated system . These properties allow it to function effectively in:
- Organic Field-Effect Transistors (OFETs)
- Organic Photovoltaics (OPVs)
- Light-Emitting Diodes (OLEDs)
The compound's ability to facilitate charge transport makes it a candidate for improving the efficiency of these devices .
Desulfurization Processes
The compound plays a crucial role in the hydrodesulfurization (HDS) process, which is essential for refining fossil fuels. Research indicates that:
- 4,6-DEDBT can be effectively desulfurized using various catalysts, including CoMo catalysts .
- It serves as a model compound for studying the desulfurization of more complex sulfur-containing hydrocarbons found in crude oil .
Table 1: Desulfurization Efficiency of 4,6-DEDBT
Catalyst Type | Desulfurization Efficiency (%) | Conditions |
---|---|---|
CoMo Catalyst | ~85% | High Temperature |
NiWS/Al2O3 | Optimal at varying Al2O3 concentrations | Varies by setup |
Synthesis of Novel Organosulfur Compounds
4,6-DEDBT acts as a building block for synthesizing more complex organosulfur compounds utilized in:
- Medicinal Chemistry : Developing pharmaceuticals with specific biological activities.
- Materials Science : Creating advanced materials with tailored properties for industrial applications.
- Catalysis : Enhancing catalytic processes through the development of new catalysts .
Case Study 1: Biodesulfurization Efficiency
A study on the biodesulfurization of dibenzothiophenes showed that bacterial strains could desulfurize 4,6-DEDBT with varying efficiencies based on concentration:
- At lower concentrations (1 mM), desulfurization efficiency reached approximately 96.7%.
- As concentration increased to 4.3 mM, efficiency dropped to around 76% .
This highlights the compound's potential in biotechnological applications aimed at reducing sulfur content in fuels.
Case Study 2: Catalytic Hydrodesulfurization
Research involving NiWS/Al2O3 catalysts demonstrated effective hydrodesulfurization of 4,6-DEDBT under optimized conditions. The study found that:
Mechanism of Action
The mechanism by which 4,6-Diethyldibenzothiophene exerts its effects primarily involves its interaction with catalytic sites during chemical reactions. For instance, in oxidative desulfurization, the compound reacts with catalytic complexes, such as Ti-η1-hydroperoxo complexes, to form sulfoxides and sulfones. The ethyl groups on the benzene rings can influence the reactivity and selectivity of these reactions by providing steric hindrance and altering electron density.
Comparison with Similar Compounds
Comparison with Structural Analogs
Key Structural and Physical Properties
The substitution pattern and alkyl chain length significantly influence the physicochemical behavior of dibenzothiophene derivatives. Below is a comparative analysis with two closely related analogs:
*Estimates based on alkyl chain length trends. Ethyl groups increase molecular weight and boiling point compared to methyl analogs.
Functional Differences
- Alkyl Chain Effects : The ethyl substituents in this compound enhance lipophilicity and thermal stability compared to the methyl-substituted analog, making it more suitable for high-temperature applications .
- Synthetic Complexity : this compound requires precise alkylation steps, whereas the synthesis of fused dithiophenes (e.g., dibenzo[d,d′]benzo[1,2-b:4,3-b′]dithiophene) involves multi-step cyclization and ketonization reactions .
Spectroscopic and Crystallographic Insights
- Crystal Structure : The fused dithiophene analog crystallizes in a planar conformation, optimizing π-π stacking for optoelectronic applications, whereas alkyl-substituted dibenzothiophenes adopt more flexible geometries .
Industrial and Research Relevance
- This compound : Valued for its high purity (≥97%) and role in developing advanced materials, including organic light-emitting diodes (OLEDs) and conductive polymers .
- 4,6-Dimethyldibenzothiophene : Often used as a reference standard in chromatography and as a precursor for further functionalization .
- Fused Dithiophenes : Explored in organic photovoltaics due to extended conjugation and strong light-harvesting capabilities .
Biological Activity
4,6-Diethyldibenzothiophene (4,6-DEDBT) is a sulfur-containing organic compound that has garnered attention due to its relevance in the petroleum industry and its potential biological activities. This article explores the biological activity of 4,6-DEDBT, focusing on its desulfurization processes and interactions with microbial systems.
Chemical Structure and Properties
4,6-DEDBT is a dibenzothiophene derivative characterized by the presence of two ethyl groups at positions 4 and 6 of the dibenzothiophene core. This structural modification impacts its steric hindrance and reactivity, particularly in biological systems.
Biological Activity Overview
The biological activity of 4,6-DEDBT primarily relates to its role in biodesulfurization processes. Microbial degradation of dibenzothiophenes is crucial for reducing sulfur content in fossil fuels. The following sections detail specific studies and findings related to the biological activity of 4,6-DEDBT.
Microbial Desulfurization Studies
Recent research has highlighted the potential of various microbial strains to metabolize 4,6-DEDBT through desulfurization pathways. Notably:
- Serratia marcescens : This bacterium has been shown to utilize 4,6-DEDBT as a sulfur source, indicating its capability for biodesulfurization. The metabolic pathway involved suggests that Serratia can effectively reduce sulfur content in diesel fuel by degrading dibenzothiophenes .
- Rhodococcus erythropolis IGTS8 : This strain demonstrated significant desulfurization activity against 4,6-DEDBT. The cells were able to convert this compound into less harmful products through a series of enzymatic reactions .
Table 1: Summary of Microbial Strains and Their Desulfurization Capabilities
Microbial Strain | Compound Used | Desulfurization Rate | Pathway Involved |
---|---|---|---|
Serratia marcescens | This compound | High | Metabolic pathway 4S |
Rhodococcus erythropolis IGTS8 | This compound | Significant | Enzymatic degradation |
Bacillus pumilus-related strain | Dibenzothiophene | Rapid | Biodesulfurization |
Adsorption Studies
Adsorption studies have also been conducted to assess the interaction of 4,6-DEDBT with various adsorbents. These studies are critical for understanding how to remove sulfur compounds from fuels effectively:
- Activated Carbon : Research indicates that activated carbon exhibits a high adsorption capacity for 4,6-DEDBT due to its extensive surface area and functional groups .
- Transition Metal Oxides : The incorporation of transition metal oxides into adsorbents has been shown to enhance the adsorption properties for dibenzothiophenes, including 4,6-DEDBT .
Q & A
Basic Research Questions
Q. What synthetic methodologies are commonly employed for 4,6-Diethyldibenzothiophene, and how do reaction parameters affect yield?
- Answer : The synthesis of dibenzothiophene derivatives typically involves cyclization reactions or functional group modifications. For example, thiophene ring formation via Ullmann coupling or Friedel-Crafts alkylation can introduce ethyl groups at specific positions. Reaction conditions such as temperature, solvent polarity, and catalyst choice (e.g., Lewis acids) critically influence yield and purity. Optimizing stoichiometry of precursors (e.g., thiophene and ethylating agents) and reaction time minimizes side products like isomerized or over-alkylated derivatives. Characterization via GC-MS and NMR ensures structural fidelity .
Q. Which analytical techniques are most robust for characterizing this compound’s structure and purity?
- Answer : High-resolution mass spectrometry (HR-MS) confirms molecular weight, while H/C NMR resolves substitution patterns and ethyl group orientation. X-ray crystallography provides definitive stereochemical validation if single crystals are obtainable. Purity is assessed via HPLC with UV detection, calibrated against certified reference standards. Thermal stability and melting points (e.g., 153–157°C for analogous compounds) are determined using differential scanning calorimetry (DSC) .
Q. What are the primary research applications of this compound in environmental or materials science?
- Answer : This compound serves as a model sulfur-containing aromatic hydrocarbon in catalytic desulfurization studies. For instance, MoO-SiO nanocomposites oxidize dibenzothiophene derivatives to sulfones, with efficiency influenced by ethyl group steric effects. It is also used as a biomarker in petroleum geochemistry to assess thermal maturity and depositional environments due to its resistance to biodegradation .
Advanced Research Questions
Q. How can experimental parameters be optimized for this compound’s oxidative desulfurization in fuel models?
- Answer : A factorial design approach evaluates variables such as catalyst loading (e.g., 10–20 wt% MoO), temperature (50–80°C), and oxidant-to-substrate ratio (e.g., HO/S molar ratio of 8:1). Kinetic studies under ultrasonic irradiation enhance mass transfer, reducing reaction time. Post-reaction analysis via GC-FID quantifies residual sulfur content, while FT-IR monitors sulfone formation. Recent studies achieved >92% desulfurization for 4,6-DMDBT analogs using zirconium-based catalysts .
Q. What computational strategies elucidate the interaction mechanisms of this compound in catalytic systems?
- Answer : Density functional theory (DFT) calculates adsorption energies on catalyst surfaces (e.g., MoO clusters), identifying active sites for sulfur oxidation. Molecular dynamics simulations model solvent effects (e.g., ionic liquids) on reaction pathways. QSAR models correlate substituent electronic effects (e.g., ethyl vs. methyl groups) with oxidative reactivity. These approaches guide catalyst design by predicting steric and electronic barriers .
Q. How do contradictory data on catalytic performance arise in desulfurization studies, and how can they be resolved?
- Answer : Discrepancies often stem from variations in catalyst synthesis (e.g., sol-gel vs. impregnation methods), leading to differences in surface area or active site distribution. Standardizing characterization protocols (BET for porosity, XPS for oxidation states) and using controlled model fuels (e.g., 500 ppm sulfur in n-octane) reduce variability. Meta-analyses of activation energies and turnover frequencies (TOF) across studies identify outlier methodologies .
Q. What geochemical insights can be derived from the distribution of this compound in sedimentary basins?
- Answer : Relative abundances of alkylated dibenzothiophenes correlate with redox conditions and salinity during sedimentation. High this compound concentrations in shale samples suggest anoxic, sulfate-rich environments. Compound-specific isotope analysis (CSIA) of δS further differentiates biogenic vs. thermogenic sulfur sources. Cross-plots against pristane/phytane ratios validate depositional models .
Q. Methodological Notes
- Synthesis Optimization : Use Design of Experiments (DoE) software to map parameter interactions and identify optimal conditions .
- Data Validation : Cross-reference chromatographic data with certified standards (e.g., Chiron’s biomarker catalogue) to ensure accuracy .
- Computational Reproducibility : Archive DFT input files and force field parameters in public repositories (e.g., Zenodo) for peer validation .
Properties
IUPAC Name |
4,6-diethyldibenzothiophene | |
---|---|---|
Source | PubChem | |
URL | https://pubchem.ncbi.nlm.nih.gov | |
Description | Data deposited in or computed by PubChem | |
InChI |
InChI=1S/C16H16S/c1-3-11-7-5-9-13-14-10-6-8-12(4-2)16(14)17-15(11)13/h5-10H,3-4H2,1-2H3 | |
Source | PubChem | |
URL | https://pubchem.ncbi.nlm.nih.gov | |
Description | Data deposited in or computed by PubChem | |
InChI Key |
UMQGGSYHJPHWFV-UHFFFAOYSA-N | |
Source | PubChem | |
URL | https://pubchem.ncbi.nlm.nih.gov | |
Description | Data deposited in or computed by PubChem | |
Canonical SMILES |
CCC1=C2C(=CC=C1)C3=CC=CC(=C3S2)CC | |
Source | PubChem | |
URL | https://pubchem.ncbi.nlm.nih.gov | |
Description | Data deposited in or computed by PubChem | |
Molecular Formula |
C16H16S | |
Source | PubChem | |
URL | https://pubchem.ncbi.nlm.nih.gov | |
Description | Data deposited in or computed by PubChem | |
DSSTOX Substance ID |
DTXSID20348890 | |
Record name | 4,6-Diethyldibenzothiophene | |
Source | EPA DSSTox | |
URL | https://comptox.epa.gov/dashboard/DTXSID20348890 | |
Description | DSSTox provides a high quality public chemistry resource for supporting improved predictive toxicology. | |
Molecular Weight |
240.4 g/mol | |
Source | PubChem | |
URL | https://pubchem.ncbi.nlm.nih.gov | |
Description | Data deposited in or computed by PubChem | |
CAS No. |
132034-91-4 | |
Record name | 4,6-Diethyldibenzothiophene | |
Source | EPA DSSTox | |
URL | https://comptox.epa.gov/dashboard/DTXSID20348890 | |
Description | DSSTox provides a high quality public chemistry resource for supporting improved predictive toxicology. | |
Retrosynthesis Analysis
AI-Powered Synthesis Planning: Our tool employs the Template_relevance Pistachio, Template_relevance Bkms_metabolic, Template_relevance Pistachio_ringbreaker, Template_relevance Reaxys, Template_relevance Reaxys_biocatalysis model, leveraging a vast database of chemical reactions to predict feasible synthetic routes.
One-Step Synthesis Focus: Specifically designed for one-step synthesis, it provides concise and direct routes for your target compounds, streamlining the synthesis process.
Accurate Predictions: Utilizing the extensive PISTACHIO, BKMS_METABOLIC, PISTACHIO_RINGBREAKER, REAXYS, REAXYS_BIOCATALYSIS database, our tool offers high-accuracy predictions, reflecting the latest in chemical research and data.
Strategy Settings
Precursor scoring | Relevance Heuristic |
---|---|
Min. plausibility | 0.01 |
Model | Template_relevance |
Template Set | Pistachio/Bkms_metabolic/Pistachio_ringbreaker/Reaxys/Reaxys_biocatalysis |
Top-N result to add to graph | 6 |
Feasible Synthetic Routes
Disclaimer and Information on In-Vitro Research Products
Please be aware that all articles and product information presented on BenchChem are intended solely for informational purposes. The products available for purchase on BenchChem are specifically designed for in-vitro studies, which are conducted outside of living organisms. In-vitro studies, derived from the Latin term "in glass," involve experiments performed in controlled laboratory settings using cells or tissues. It is important to note that these products are not categorized as medicines or drugs, and they have not received approval from the FDA for the prevention, treatment, or cure of any medical condition, ailment, or disease. We must emphasize that any form of bodily introduction of these products into humans or animals is strictly prohibited by law. It is essential to adhere to these guidelines to ensure compliance with legal and ethical standards in research and experimentation.