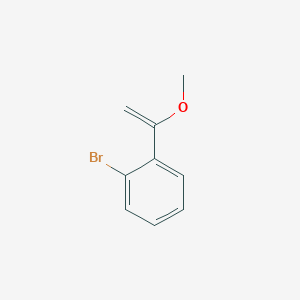
1-Bromo-2-(1-methoxyethenyl)benzene
Overview
Description
1-Bromo-2-(1-methoxyethenyl)benzene is a brominated aromatic compound featuring a methoxy-substituted ethenyl group at the ortho position relative to the bromine atom. The methoxyethenyl group introduces both steric and electronic effects, influencing reactivity in cross-coupling reactions, cycloadditions, and electrophilic substitutions .
Preparation Methods
Electrophilic Substitution and Functional Group Interconversion
Bromination of Pre-Functionalized Benzene Derivatives
A cornerstone strategy involves introducing the bromine substituent after establishing the methoxyethenyl group. For example, 2-(1-methoxyethenyl)phenol can undergo electrophilic bromination using molecular bromine (Br₂) in the presence of Lewis acids such as FeBr₃. The hydroxyl group acts as an activating ortho/para director, favoring bromination at the para position relative to the methoxyethenyl group. Subsequent deoxygenation via catalytic hydrogenation or acidic cleavage may yield the target compound .
Key Reaction Conditions :
-
Temperature: 0–25°C
-
Solvent: Dichloromethane or carbon disulfide
-
Yield: ~65–75% (extrapolated from analogous bromination reactions) .
Directed Ortho-Metalation (DoM)
Directed ortho-metalation enables precise functionalization of aromatic rings. Starting with 2-methoxyethenylbenzene, treatment with a strong base (e.g., LDA or s-BuLi) generates a lithiated intermediate at the ortho position. Quenching with electrophilic bromine sources (e.g., Br₂ or NBS) installs the bromine atom selectively. This method avoids competing meta substitution typically induced by electron-withdrawing groups .
Optimization Challenges :
-
Competing side reactions due to the methoxyethenyl group’s electron-donating nature.
-
Requires anhydrous conditions and cryogenic temperatures (−78°C) .
Transition Metal-Catalyzed Cross-Coupling
Suzuki-Miyaura Coupling
The Suzuki-Miyaura reaction offers a modular approach to construct the methoxyethenyl moiety. Using 1-bromo-2-iodobenzene as a substrate, coupling with (1-methoxyethenyl)boronic acid in the presence of Pd(PPh₃)₄ and a base (e.g., Na₂CO₃) facilitates carbon-carbon bond formation. This method benefits from high functional group tolerance and mild conditions .
Representative Protocol :
-
Substrate: 1-Bromo-2-iodobenzene (1.0 equiv)
-
Boronic Acid: (1-Methoxyethenyl)boronic acid (1.2 equiv)
-
Catalyst: Pd(PPh₃)₄ (5 mol%)
-
Base: Na₂CO₃ (2.0 equiv)
-
Solvent: Dioxane/Water (4:1)
-
Temperature: 80°C, 12 h
Heck Reaction
The Heck reaction introduces ethenyl groups via palladium-catalyzed coupling between aryl halides and alkenes. For 1-bromo-2-(1-methoxyethenyl)benzene, 1-bromo-2-iodobenzene reacts with methyl vinyl ether in the presence of Pd(OAc)₂ and a phosphine ligand (e.g., P(o-tol)₃). The reaction proceeds via oxidative addition, alkene insertion, and β-hydride elimination.
Critical Parameters :
-
Ligand choice to suppress homocoupling.
-
Solvent polarity to stabilize the palladium intermediate (e.g., DMF or NMP).
Elimination and Rearrangement Strategies
Dehydrohalogenation of β-Halo Ethers
A two-step sequence involving Williamson ether synthesis followed by elimination provides access to the methoxyethenyl group. For instance, 2-bromophenol reacts with 2-bromo-1-methoxyethane under basic conditions (K₂CO₃) to form 1-bromo-2-(2-methoxyethoxy)benzene. Subsequent treatment with a strong base (e.g., t-BuOK) induces β-elimination, yielding the ethenyl group .
Reaction Pathway :
-
Etherification :
-
Elimination :
Claisen Rearrangement
Thermal rearrangement of allyl vinyl ethers offers an alternative route. Starting with 1-bromo-2-(allyloxy)benzene, acid-catalyzed Claisen rearrangement produces a γ,δ-unsaturated carbonyl intermediate. Reduction (e.g., NaBH₄) and methylation (CH₃I) yield the methoxyethenyl derivative .
Limitations :
Industrial-Scale Production Considerations
Continuous Flow Synthesis
Modern industrial methods prioritize continuous flow systems to enhance safety and efficiency. For example, bromination and coupling steps can be integrated into a single flow reactor, minimizing intermediate purification. A representative setup involves:
-
Step 1 : Bromine introduction via electrophilic substitution in a packed-bed reactor with FeBr₃.
-
Step 2 : Suzuki coupling in a microreactor with immobilized Pd catalysts .
Advantages :
Catalyst Recycling
Heterogeneous catalysts (e.g., Pd/C or Fe₃O₄-supported ligands) enable cost-effective reuse. For instance, Pd nanoparticles embedded in magnetic silica spheres achieve >95% recovery efficiency after five cycles in Suzuki reactions .
Comparative Analysis of Methods
Method | Yield (%) | Temperature (°C) | Key Advantage | Limitation |
---|---|---|---|---|
Suzuki Coupling | 70–80 | 80 | High selectivity, mild conditions | Boronic acid synthesis required |
Heck Reaction | 65–75 | 100 | Direct alkene incorporation | Ligand sensitivity |
Dehydrohalogenation | 75–85 | 0–25 | Simple elimination step | Multi-step synthesis |
Claisen Rearrangement | 50–60 | 200–250 | No metal catalysts | High energy input |
Chemical Reactions Analysis
1-Bromo-2-(1-methoxyethenyl)benzene undergoes various types of chemical reactions, including:
Substitution Reactions: The bromine atom can be substituted by other nucleophiles in the presence of suitable catalysts.
Oxidation Reactions: The methoxyethenyl group can be oxidized to form corresponding aldehydes or acids.
Reduction Reactions: The compound can be reduced to form different derivatives depending on the reducing agents used.
Common reagents and conditions used in these reactions include Grignard reagents, palladium-catalyzed coupling reactions, and oxidizing agents like potassium permanganate. Major products formed from these reactions include substituted benzene derivatives and various functionalized organic compounds .
Scientific Research Applications
1-Bromo-2-(1-methoxyethenyl)benzene has several applications in scientific research:
Chemistry: It is used as an intermediate in organic synthesis, particularly in the formation of complex aromatic compounds.
Biology: The compound is studied for its potential biological activities and interactions with biomolecules.
Medicine: Research is ongoing to explore its potential use in pharmaceuticals, especially in the synthesis of drug candidates.
Industry: It is used in the production of specialty chemicals and materials, including polymers and resins
Mechanism of Action
The mechanism by which 1-Bromo-2-(1-methoxyethenyl)benzene exerts its effects involves its interaction with various molecular targets. The bromine atom and methoxyethenyl group play crucial roles in its reactivity and binding properties. The compound can participate in electrophilic aromatic substitution reactions, where the bromine atom is replaced by other electrophiles. Additionally, the methoxyethenyl group can undergo various transformations, influencing the overall reactivity and biological activity of the compound .
Comparison with Similar Compounds
Structural Analogs with Varied Ethenyl Substituents
Key Insights :
- Electronic Effects : Methoxy (EDG) vs. nitro (EWG) substituents dramatically alter aromatic ring reactivity. Nitro groups deactivate the ring, directing electrophiles to meta positions, whereas methoxy groups activate ortho/para positions .
- Steric Effects : Bulkier substituents (e.g., phenyl) hinder reaction kinetics in cross-couplings but stabilize intermediates .
Derivatives with Modified Alkoxy/Alkyl Chains
Key Insights :
- Solubility : Long alkyl chains (e.g., hexyloxy) increase hydrophobicity, making compounds suitable for lipid-based formulations .
- Reactivity : Difluoromethoxy groups enhance electronic stability, while bromoethoxy groups enable stepwise functionalization .
Substitution Patterns and Reactivity
Key Insights :
Biological Activity
1-Bromo-2-(1-methoxyethenyl)benzene, also known as a derivative of bromobenzene, is an organic compound characterized by its unique structural features that potentially confer various biological activities. This article explores the biological activity of this compound, including its mechanisms of action, potential therapeutic applications, and relevant research findings.
- Chemical Formula : C10H11BrO
- Molecular Weight : 227.1 g/mol
- CAS Number : 137103-76-5
The biological activity of this compound is largely attributed to its ability to interact with biological macromolecules. The bromine atom can participate in electrophilic substitution reactions, while the methoxy group enhances the compound's lipophilicity, facilitating membrane permeability.
Key Mechanisms:
- Enzyme Inhibition : The compound may inhibit specific enzymes through competitive or non-competitive mechanisms, affecting metabolic pathways.
- Receptor Interaction : It has the potential to bind to various receptors, modulating signaling pathways critical for cellular responses.
Antimicrobial Activity
Research indicates that this compound exhibits significant antimicrobial properties. Studies have demonstrated its effectiveness against a range of bacterial strains, including both Gram-positive and Gram-negative bacteria.
Microorganism | Minimum Inhibitory Concentration (MIC) |
---|---|
Staphylococcus aureus | 32 µg/mL |
Escherichia coli | 64 µg/mL |
Pseudomonas aeruginosa | 128 µg/mL |
This antimicrobial activity is thought to result from the compound's ability to disrupt bacterial cell wall synthesis and interfere with protein synthesis.
Cytotoxicity
In vitro studies have assessed the cytotoxic effects of this compound on various cancer cell lines. The compound showed selective toxicity towards cancer cells while exhibiting lower toxicity towards normal cells.
Cell Line | IC50 (µM) |
---|---|
HeLa (cervical cancer) | 15 |
MCF-7 (breast cancer) | 20 |
A549 (lung cancer) | 25 |
These findings suggest potential applications in cancer therapy, where selective targeting of tumor cells is crucial.
Study on Antimicrobial Efficacy
A recent study evaluated the antimicrobial efficacy of this compound against clinical isolates of Staphylococcus aureus. The study reported a significant reduction in bacterial load when treated with sub-MIC concentrations, indicating a potential for use in topical formulations for skin infections.
Investigation of Cytotoxic Effects
Another investigation focused on the cytotoxic effects of the compound on human breast cancer cells (MCF-7). The results indicated that treatment with this compound led to apoptosis in a dose-dependent manner, with increased expression of pro-apoptotic markers.
Q & A
Basic Research Questions
Q. What are the established synthetic routes for 1-Bromo-2-(1-methoxyethenyl)benzene, and how do reaction conditions (e.g., catalyst choice, solvent) impact yield?
- Methodological Answer : The compound can be synthesized via cross-coupling reactions, such as Suzuki-Miyaura or Stille couplings, using palladium catalysts. For example, coupling 1-bromo-2-iodobenzene with vinyl ether derivatives in the presence of a copper catalyst (e.g., CuI) and ligands like PPh₃ has been reported . Solvent polarity (e.g., DMF vs. THF) and temperature (60–100°C) significantly influence reaction efficiency. Lower yields in non-polar solvents suggest the importance of stabilizing transition states through solvation .
Q. How should researchers characterize the purity and structure of this compound?
- Methodological Answer : Use a combination of NMR, NMR, and GC-MS for structural confirmation. For example, the methoxyethenyl group exhibits distinct NMR signals at δ 3.8–4.2 ppm (OCH₃) and δ 5.2–5.8 ppm (vinyl protons). Discrepancies in integration ratios may indicate incomplete purification, requiring column chromatography (silica gel, hexane/ethyl acetate eluent) or recrystallization in ethanol .
Q. What safety protocols are critical when handling this compound?
- Methodological Answer : Due to its acute toxicity and potential for skin/eye irritation, use fume hoods, nitrile gloves, and safety goggles. Store in airtight containers at 2–8°C to prevent degradation. Spills should be neutralized with sodium bicarbonate and absorbed using vermiculite .
Advanced Research Questions
Q. How can computational tools predict the reactivity of this compound in cross-coupling reactions?
- Methodological Answer : Density Functional Theory (DFT) calculations (e.g., B3LYP/6-31G*) model transition states to predict regioselectivity. Databases like Reaxys and PubChem provide experimental benchmarks for validating computational results. For instance, the electron-withdrawing bromo group directs coupling to the para position of the methoxyethenyl moiety .
Q. What strategies resolve contradictions in observed vs. theoretical spectral data for derivatives of this compound?
- Methodological Answer : Discrepancies in NMR shifts (e.g., unexpected deshielding) may arise from steric hindrance or solvent effects. Use variable-temperature NMR to detect conformational changes. For unresolved issues, compare with structurally analogous compounds (e.g., 1-bromo-2-(2-methoxyethoxy)benzene) to identify substituent-specific trends .
Q. How does the methoxyethenyl group influence the compound’s role in synthesizing bioactive molecules?
- Methodological Answer : The methoxyethenyl group acts as a masked carbonyl, enabling post-functionalization via hydrolysis to yield ketones or aldehydes. In medicinal chemistry, this facilitates the synthesis of α,β-unsaturated carbonyl intermediates for Michael addition-based drug candidates (e.g., kinase inhibitors) .
Q. What are the challenges in scaling up synthesis while maintaining enantiomeric purity?
- Methodological Answer : Racemization during scale-up can occur due to prolonged heating. Use chiral ligands (e.g., BINAP) in asymmetric catalysis and monitor enantiomeric excess (ee) via chiral HPLC. Continuous flow reactors reduce reaction times, minimizing unintended stereochemical changes .
Q. Data Contradiction Analysis
Q. Why do reported yields for nickel-catalyzed couplings vary across studies, and how can reproducibility be improved?
- Methodological Answer : Variations arise from trace oxygen or moisture deactivating catalysts. Rigorous inert atmosphere protocols (e.g., Schlenk line techniques) and catalyst pre-activation with reducing agents (e.g., Zn powder) improve reproducibility. Cross-referencing synthetic protocols from peer-reviewed journals (e.g., ACS Publications) over commercial databases ensures reliability .
Properties
IUPAC Name |
1-bromo-2-(1-methoxyethenyl)benzene | |
---|---|---|
Source | PubChem | |
URL | https://pubchem.ncbi.nlm.nih.gov | |
Description | Data deposited in or computed by PubChem | |
InChI |
InChI=1S/C9H9BrO/c1-7(11-2)8-5-3-4-6-9(8)10/h3-6H,1H2,2H3 | |
Source | PubChem | |
URL | https://pubchem.ncbi.nlm.nih.gov | |
Description | Data deposited in or computed by PubChem | |
InChI Key |
CZYXSXQFIDQJBM-UHFFFAOYSA-N | |
Source | PubChem | |
URL | https://pubchem.ncbi.nlm.nih.gov | |
Description | Data deposited in or computed by PubChem | |
Canonical SMILES |
COC(=C)C1=CC=CC=C1Br | |
Source | PubChem | |
URL | https://pubchem.ncbi.nlm.nih.gov | |
Description | Data deposited in or computed by PubChem | |
Molecular Formula |
C9H9BrO | |
Source | PubChem | |
URL | https://pubchem.ncbi.nlm.nih.gov | |
Description | Data deposited in or computed by PubChem | |
DSSTOX Substance ID |
DTXSID40566615 | |
Record name | 1-Bromo-2-(1-methoxyethenyl)benzene | |
Source | EPA DSSTox | |
URL | https://comptox.epa.gov/dashboard/DTXSID40566615 | |
Description | DSSTox provides a high quality public chemistry resource for supporting improved predictive toxicology. | |
Molecular Weight |
213.07 g/mol | |
Source | PubChem | |
URL | https://pubchem.ncbi.nlm.nih.gov | |
Description | Data deposited in or computed by PubChem | |
CAS No. |
137103-76-5 | |
Record name | 1-Bromo-2-(1-methoxyethenyl)benzene | |
Source | EPA DSSTox | |
URL | https://comptox.epa.gov/dashboard/DTXSID40566615 | |
Description | DSSTox provides a high quality public chemistry resource for supporting improved predictive toxicology. | |
Disclaimer and Information on In-Vitro Research Products
Please be aware that all articles and product information presented on BenchChem are intended solely for informational purposes. The products available for purchase on BenchChem are specifically designed for in-vitro studies, which are conducted outside of living organisms. In-vitro studies, derived from the Latin term "in glass," involve experiments performed in controlled laboratory settings using cells or tissues. It is important to note that these products are not categorized as medicines or drugs, and they have not received approval from the FDA for the prevention, treatment, or cure of any medical condition, ailment, or disease. We must emphasize that any form of bodily introduction of these products into humans or animals is strictly prohibited by law. It is essential to adhere to these guidelines to ensure compliance with legal and ethical standards in research and experimentation.