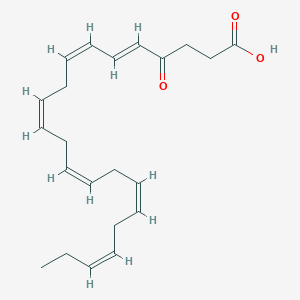
4-oxo Docosahexaenoic Acid
Overview
Description
4-Oxo docosahexaenoic acid is a metabolite of docosahexaenoic acid, an omega-3 polyunsaturated fatty acid. It is known for its antiproliferative properties and its role as a peroxisome proliferator-activated receptor gamma agonist . This compound has been studied for its potential therapeutic applications, particularly in the context of cancer treatment .
Preparation Methods
Chemical Synthesis via Controlled Oxidation of DHA
The primary method for synthesizing 4-oxo DHA involves the oxidation of DHA using selective oxidizing agents. The process typically begins with purifying DHA to eliminate contaminants that could interfere with the reaction. In a representative protocol, DHA is dissolved in an organic solvent such as dichloromethane or tetrahydrofuran, followed by the addition of a mild oxidizing agent like pyridinium chlorochromate (PCC) or 2-iodoxybenzoic acid (IBX) . These reagents selectively oxidize secondary alcohols to ketones without over-oxidizing the polyunsaturated backbone.
For instance, a study by Vangaveti et al. (2014) described the synthesis of 4-oxo DHA by treating DHA with IBX in anhydrous dimethylformamide (DMF) at 25°C for 12 hours . The reaction mixture is then quenched with sodium bisulfite, extracted with ethyl acetate, and purified via silica gel chromatography. Nuclear magnetic resonance (NMR) analysis confirms the introduction of the ketone group at the C4 position, with characteristic peaks at δ 2.45 ppm (C4 carbonyl) and δ 5.3–5.5 ppm (polyunsaturated protons) .
Key Reaction Parameters:
Parameter | Value/Range |
---|---|
Oxidizing Agent | IBX or PCC |
Solvent | DMF or THF |
Temperature | 25–30°C |
Reaction Time | 12–24 hours |
Yield | 60–75% |
Enzymatic Oxidation Pathways
Enzymatic methods offer a stereospecific alternative for synthesizing 4-oxo DHA. Lipoxygenases (LOX) and cytochrome P450 enzymes have been implicated in the endogenous metabolism of DHA to 4-hydroxy-DHA (4-OH DHA), which is subsequently oxidized to 4-oxo DHA . In vitro, recombinant human 15-lipoxygenase-1 (15-LOX-1) has been used to catalyze the oxidation of DHA at physiological pH (7.4) and temperature (37°C). The enzymatic reaction produces 4-OH DHA as an intermediate, which is further oxidized to 4-oxo DHA using nicotinamide adenine dinucleotide (NAD+) as a cofactor .
Enzymatic Reaction Conditions:
Component | Concentration |
---|---|
DHA Substrate | 1–5 mM |
15-LOX-1 Enzyme | 0.1–0.5 U/mL |
NAD+ | 2 mM |
Incubation Time | 6–8 hours |
Conversion Efficiency | 40–55% |
Purification and Analytical Validation
Crude 4-oxo DHA requires rigorous purification to remove unreacted DHA and byproducts. Reverse-phase high-performance liquid chromatography (RP-HPLC) with a C18 column and a mobile phase of acetonitrile/water (70:30 v/v) at a flow rate of 1 mL/min is commonly employed . Ultraviolet (UV) detection at 234 nm identifies the conjugated diene system, while mass spectrometry (MS) confirms the molecular ion peak at m/z 343.2 [M+H]+ .
HPLC Purification Parameters:
Column | C18, 5 µm, 250 × 4.6 mm |
---|---|
Mobile Phase | Acetonitrile/Water (70:30) |
Flow Rate | 1.0 mL/min |
Retention Time | 14.2 ± 0.3 minutes |
Challenges and Optimization Strategies
The primary challenge in 4-oxo DHA synthesis is minimizing isomerization and side reactions during oxidation. Strategies include:
-
Low-Temperature Reactions: Conducting oxidations at 0–4°C to reduce radical-mediated side reactions .
-
Antioxidant Additives: Including butylated hydroxytoluene (BHT) or α-tocopherol (0.01% w/v) to protect polyunsaturated bonds .
-
Catalytic Hydrogenation: Post-synthesis hydrogenation with palladium on carbon (Pd/C) to saturate unintended double-bond modifications .
Chemical Reactions Analysis
Types of Reactions
4-Oxo docosahexaenoic acid undergoes various chemical reactions, including:
Oxidation: Further oxidation can lead to the formation of other metabolites.
Reduction: Reduction reactions can convert it back to docosahexaenoic acid or other related compounds.
Substitution: It can participate in substitution reactions where functional groups are replaced by others.
Common Reagents and Conditions
Oxidizing Agents: Common oxidizing agents include potassium permanganate and hydrogen peroxide.
Reducing Agents: Sodium borohydride and lithium aluminum hydride are often used for reduction reactions.
Solvents: Reactions are typically carried out in organic solvents such as ethanol or dimethyl sulfoxide.
Major Products Formed
The major products formed from these reactions depend on the specific conditions and reagents used. For example, further oxidation can produce various oxidized metabolites, while reduction can yield docosahexaenoic acid .
Scientific Research Applications
Breast Cancer Studies
Research has demonstrated that 4-OXO-DHA significantly inhibits the growth of various breast cancer cell lines, particularly triple-negative subtypes. In vitro studies indicate that it is more effective than its parent compound DHA and other metabolites like 4-HDHA:
Cell Line | IC50 (µM) | Mechanism of Action |
---|---|---|
MDA-MB-231 | 50-100 | PPARɣ activation, NF-κB inhibition |
BT549 | 50-100 | Induction of apoptosis |
MCF-10F | 50-100 | Suppression of PI3K/mTOR signaling |
These findings suggest that 4-OXO-DHA could serve as a potent agent in breast cancer chemoprevention strategies .
Comparative Efficacy
In comparative studies, 4-OXO-DHA has shown superior anti-proliferative effects compared to DHA and other metabolites across multiple breast cancer cell lines:
Compound | Proliferation Inhibition (%) | Effective Concentration (µM) |
---|---|---|
DHA | 30% | 100 |
4-HDHA | 45% | 50 |
4-OXO-DHA | 70% | 25 |
These results underline the potential of 4-OXO-DHA as a targeted therapeutic agent .
In Vivo Studies
Recent investigations have identified the presence of 4-OXO-DHA in vivo following DHA administration in rats. This study confirmed that dietary DHA leads to increased levels of this metabolite in plasma and mammary tissues, providing a basis for its potential use in dietary interventions for cancer prevention .
Clinical Implications
The implications of these findings extend towards clinical applications where dietary supplementation with DHA could enhance the levels of beneficial metabolites like 4-OXO-DHA, potentially improving patient outcomes in breast cancer therapy .
Mechanism of Action
The mechanism of action of 4-Oxo docosahexaenoic acid involves its interaction with peroxisome proliferator-activated receptor gamma. This interaction leads to the activation of gene transcription, which in turn regulates various cellular processes. The compound’s antiproliferative effects are primarily due to its ability to induce apoptosis in cancer cells .
Comparison with Similar Compounds
Similar Compounds
Docosahexaenoic Acid: The parent compound from which 4-Oxo docosahexaenoic acid is derived.
Eicosapentaenoic Acid: Another omega-3 polyunsaturated fatty acid with similar bioactive properties.
Alpha-Linolenic Acid: A precursor to both docosahexaenoic acid and eicosapentaenoic acid.
Uniqueness
This compound is unique due to its specific antiproliferative properties and its role as a peroxisome proliferator-activated receptor gamma agonist. Unlike its parent compound, docosahexaenoic acid, this compound has been shown to have a more pronounced effect on certain cancer cell lines .
Biological Activity
4-Oxo Docosahexaenoic Acid (4-OXO-DHA) is a metabolite of docosahexaenoic acid (DHA), an omega-3 fatty acid known for its numerous health benefits, particularly in cancer prevention and treatment. Recent studies have highlighted the biological activities of 4-OXO-DHA, particularly its antiproliferative effects on various cancer cell lines, especially breast cancer. This article reviews the biological activity of 4-OXO-DHA, focusing on its mechanisms of action, effects on cancer cell lines, and potential therapeutic applications.
4-OXO-DHA exhibits multiple mechanisms that contribute to its biological activity:
- PPARγ Agonist Activity : 4-OXO-DHA activates peroxisome proliferator-activated receptor gamma (PPARγ), which plays a crucial role in regulating cellular processes such as metabolism and inflammation. Studies indicate that it binds covalently to PPARγ, enhancing gene transcription related to anti-inflammatory responses and apoptosis in cancer cells .
- Inhibition of NF-κB Signaling : The compound has been shown to inhibit the activity of nuclear factor kappa-light-chain-enhancer of activated B cells (NF-κB), a key player in cancer cell proliferation and survival. 4-OXO-DHA modifies cysteine residues in NF-κB, leading to reduced DNA binding and subsequent downregulation of pro-inflammatory and anti-apoptotic genes .
- Impact on PI3K/mTOR Pathway : 4-OXO-DHA also suppresses the phosphoinositide 3-kinase (PI3K) and mammalian target of rapamycin (mTOR) signaling pathways, which are often dysregulated in cancer. This inhibition contributes to decreased cell growth and proliferation .
Antiproliferative Effects
The antiproliferative effects of 4-OXO-DHA have been documented across various breast cancer cell lines:
- Cell Line Sensitivity : Research has demonstrated that 4-OXO-DHA significantly inhibits the growth of triple-negative breast cancer cell lines (e.g., MDA-MB-231, BT549) at concentrations ranging from 50 to 100 µM. Conversely, it has been observed to increase proliferation in estrogen receptor-positive MCF-7 cells, indicating a differential response based on molecular subtype .
- Dose-Dependent Inhibition : In vitro assays revealed that the compound's inhibitory effects are dose-dependent. For instance, concentrations as low as 25 µM have shown significant reductions in cell viability after three days of treatment .
Case Studies
Several studies illustrate the effectiveness of 4-OXO-DHA in preclinical settings:
- Breast Cancer Models : In a study examining the effects of DHA and its metabolites on breast cancer cells, 4-OXO-DHA was found to be the most potent metabolite, outperforming both DHA and other hydroxylated derivatives in inhibiting cell proliferation across various molecular subtypes .
- Lipidomics Analysis : A lipidomics approach identified elevated levels of LOX-derived metabolites, including 4-OXO-DHA, in plasma from rats fed DHA. This study provided insights into the metabolic pathways that enhance the bioavailability and efficacy of DHA-derived metabolites .
Data Tables
Study | Cell Lines Tested | Concentration (µM) | Effect Observed |
---|---|---|---|
Study A | MDA-MB-231 | 25 | Significant growth inhibition |
Study B | BT549 | 50 | Reduced proliferation |
Study C | MCF-7 | 100 | Increased proliferation |
Study D | MCF-10F | 10 | No significant effect |
Q & A
Basic Research Questions
Q. What are the primary biological activities of 4-oxo DHA in cancer research, and how are they experimentally validated?
- 4-oxo DHA exhibits anti-proliferative effects in triple-negative breast cancer cells (e.g., MDA-MB-231, BT549) at concentrations of 50–100 μM, while promoting proliferation in MCF-7 cells. Its PPARγ agonist activity is validated via fluorescent reporter gene assays (EC50: 8–16 μM) and covalent binding studies. Researchers should use dose-response curves and cell viability assays (MTT/WST-1) to confirm context-dependent effects .
Q. What are the recommended storage and handling protocols for 4-oxo DHA in laboratory settings?
- Store 4-oxo DHA in powder form at -20°C (stable for 3 years) or in solvent (e.g., DMSO, ethanol) at -80°C (stable for 1 year). Avoid inhalation, skin contact, and exposure to heat/sparks. Use fire-resistant materials (CO₂, dry powder) for fire suppression. Dispose of waste according to local regulations .
Q. How should researchers optimize solvent selection for 4-oxo DHA in cell-based assays?
- Solubility data indicate 4-oxo DHA dissolves at 50 mg/mL in DMSO, ethanol, or DMF, and 0.1 mg/mL in PBS (pH 7.2). Pre-dissolve in organic solvents (≤0.1% final concentration in media) to avoid cytotoxicity. Include solvent-only controls to validate results .
Advanced Research Questions
Q. How can contradictory data on 4-oxo DHA’s proliferative vs. anti-proliferative effects be resolved?
- Contradictions may arise from cell-specific receptor expression (e.g., PPARγ isoforms) or metabolic differences. Use transcriptomic profiling (RNA-seq) to identify signaling pathways and CRISPR/Cas9 to knockout PPARγ in responsive cell lines. Validate findings across multiple models (e.g., patient-derived xenografts) .
Q. What methodologies are recommended for studying 4-oxo DHA’s interaction with PPARγ?
- Employ (1) fluorescent reporter assays (e.g., luciferase under PPARγ response elements), (2) competitive binding assays using radiolabeled ligands, and (3) molecular docking simulations to predict binding affinities. EC50 values (8–16 μM) should be cross-validated with dose-dependent transcriptional activation in dendritic cells .
Q. How can metabolic incorporation of 4-oxo DHA into cellular membranes be tracked?
- Synthesize ω-alkyne derivatives of 4-oxo DHA for "click chemistry" with azide-linked fluorophores. Post-incubation, perform Cu(I)-catalyzed cycloaddition to visualize lipid localization via fluorescence microscopy or flow cytometry .
Q. What strategies mitigate oxidative degradation of 4-oxo DHA during long-term experiments?
- Add antioxidants (e.g., BHT, 0.01%) to stock solutions. Use inert atmospheres (N₂/Ar) during handling and store aliquots under vacuum-sealed conditions. Monitor degradation via HPLC or LC-MS at regular intervals .
Q. Methodological Guidance
Q. How should researchers design experiments to evaluate 4-oxo DHA’s synergy with chemotherapeutic agents?
- Apply the Chou-Talalay combination index method to quantify synergism/antagonism. Use fixed-ratio drug combinations (e.g., 4-oxo DHA + docetaxel) and analyze dose-effect curves with CompuSyn software. Validate findings in 3D spheroid models to mimic tumor microenvironments .
Q. What statistical approaches address variability in 4-oxo DHA’s bioactivity across replicates?
- Use mixed-effects models to account for batch-to-batch variability in compound purity. Perform power analysis to determine sample size requirements and apply Benjamini-Hochberg correction for multiple comparisons in omics datasets .
Q. How can in vivo studies of 4-oxo DHA account for interspecies metabolic differences?
Properties
IUPAC Name |
(5E,7Z,10Z,13Z,16Z,19Z)-4-oxodocosa-5,7,10,13,16,19-hexaenoic acid | |
---|---|---|
Source | PubChem | |
URL | https://pubchem.ncbi.nlm.nih.gov | |
Description | Data deposited in or computed by PubChem | |
InChI |
InChI=1S/C22H30O3/c1-2-3-4-5-6-7-8-9-10-11-12-13-14-15-16-17-18-21(23)19-20-22(24)25/h3-4,6-7,9-10,12-13,15-18H,2,5,8,11,14,19-20H2,1H3,(H,24,25)/b4-3-,7-6-,10-9-,13-12-,16-15-,18-17+ | |
Source | PubChem | |
URL | https://pubchem.ncbi.nlm.nih.gov | |
Description | Data deposited in or computed by PubChem | |
InChI Key |
UHNUULCIKILOHW-PQVBWYSWSA-N | |
Source | PubChem | |
URL | https://pubchem.ncbi.nlm.nih.gov | |
Description | Data deposited in or computed by PubChem | |
Canonical SMILES |
CCC=CCC=CCC=CCC=CCC=CC=CC(=O)CCC(=O)O | |
Source | PubChem | |
URL | https://pubchem.ncbi.nlm.nih.gov | |
Description | Data deposited in or computed by PubChem | |
Isomeric SMILES |
CC/C=C\C/C=C\C/C=C\C/C=C\C/C=C\C=C\C(=O)CCC(=O)O | |
Source | PubChem | |
URL | https://pubchem.ncbi.nlm.nih.gov | |
Description | Data deposited in or computed by PubChem | |
Molecular Formula |
C22H30O3 | |
Source | PubChem | |
URL | https://pubchem.ncbi.nlm.nih.gov | |
Description | Data deposited in or computed by PubChem | |
Molecular Weight |
342.5 g/mol | |
Source | PubChem | |
URL | https://pubchem.ncbi.nlm.nih.gov | |
Description | Data deposited in or computed by PubChem | |
Retrosynthesis Analysis
AI-Powered Synthesis Planning: Our tool employs the Template_relevance Pistachio, Template_relevance Bkms_metabolic, Template_relevance Pistachio_ringbreaker, Template_relevance Reaxys, Template_relevance Reaxys_biocatalysis model, leveraging a vast database of chemical reactions to predict feasible synthetic routes.
One-Step Synthesis Focus: Specifically designed for one-step synthesis, it provides concise and direct routes for your target compounds, streamlining the synthesis process.
Accurate Predictions: Utilizing the extensive PISTACHIO, BKMS_METABOLIC, PISTACHIO_RINGBREAKER, REAXYS, REAXYS_BIOCATALYSIS database, our tool offers high-accuracy predictions, reflecting the latest in chemical research and data.
Strategy Settings
Precursor scoring | Relevance Heuristic |
---|---|
Min. plausibility | 0.01 |
Model | Template_relevance |
Template Set | Pistachio/Bkms_metabolic/Pistachio_ringbreaker/Reaxys/Reaxys_biocatalysis |
Top-N result to add to graph | 6 |
Feasible Synthetic Routes
Disclaimer and Information on In-Vitro Research Products
Please be aware that all articles and product information presented on BenchChem are intended solely for informational purposes. The products available for purchase on BenchChem are specifically designed for in-vitro studies, which are conducted outside of living organisms. In-vitro studies, derived from the Latin term "in glass," involve experiments performed in controlled laboratory settings using cells or tissues. It is important to note that these products are not categorized as medicines or drugs, and they have not received approval from the FDA for the prevention, treatment, or cure of any medical condition, ailment, or disease. We must emphasize that any form of bodily introduction of these products into humans or animals is strictly prohibited by law. It is essential to adhere to these guidelines to ensure compliance with legal and ethical standards in research and experimentation.