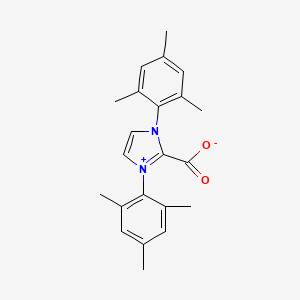
1,3-Dimesitylimidazolium-2-carboxylate
Overview
Description
1,3-Dimesitylimidazolium-2-carboxylate (CAS: 675877-56-2) is a zwitterionic salt with the molecular formula C₂₂H₂₄N₂O₂ and a molecular weight of 348.44 g/mol . It features bulky mesityl (2,4,6-trimethylphenyl) substituents on the nitrogen atoms of the imidazolium ring, which confer steric hindrance and influence its chemical stability, solubility, and reactivity. The compound is a solid at room temperature, decomposes at 241°C, and is soluble in methanol . Its primary use is in laboratory research, particularly in exploring steric effects in catalysis or ionic liquid systems .
Preparation Methods
Direct Carboxylation via Carbon Dioxide Insertion
The most widely documented method for synthesizing 1,3-dimesitylimidazolium-2-carboxylate involves the direct carboxylation of 1,3-dimesitylimidazolium chloride using carbon dioxide (CO₂) under basic conditions. This approach, pioneered by Crabtree and later refined by Fèvre et al., leverages the nucleophilic reactivity of deprotonated imidazolium salts.
Experimental Procedure
- Deprotonation : 1,3-Dimesitylimidazolium chloride (5 mmol) is treated with potassium bis(trimethylsilyl)amide (6 mmol) in anhydrous tetrahydrofuran (THF) under argon. The mixture is stirred for 4 hours, yielding a deep orange solution indicative of the free carbene intermediate.
- CO₂ Insertion : Dry CO₂ gas is bubbled through the solution for 1 hour, resulting in the immediate precipitation of a white solid. The carboxylate product is isolated via filtration, washed with diethyl ether, and dried under vacuum.
Key Parameters
- Yield : 87% (1.52 g from 5 mmol starting material).
- Purity : Confirmed by ¹H NMR (DMSO-d₆), with characteristic signals at δ 2.09 ppm (ortho-CH₃), δ 2.33 ppm (para-CH₃), and δ 7.86 ppm (imidazolium @CHN).
- Mechanistic Insight : The reaction proceeds via deprotonation to generate an NHC, which subsequently reacts with CO₂ to form the carboxylate adduct. This avoids the need for hazardous bases or metal catalysts.
Alkylation-Carboxylation with Dimethyl Carbonate
An alternative route, adapted from the synthesis of 1,3-dimethylimidazolium-2-carboxylate, involves sequential N-alkylation and C-carboxylation using dimethyl carbonate (DMC). While originally developed for simpler imidazolium systems, this method has been extended to bulkier aryl substituents like mesityl groups.
Synthetic Steps
- N-Alkylation : 1-Methylimidazole is reacted with mesityl bromide in acetonitrile at 80°C for 24 hours, yielding 1,3-dimesitylimidazolium bromide.
- C-Carboxylation : The bromide salt is treated with excess DMC at 150°C under autogenous pressure. The carboxylate forms via nucleophilic attack of the C2 position on the carbonyl carbon of DMC.
Optimization Data
Parameter | Value |
---|---|
Temperature | 150°C |
Reaction Time | 48 hours |
Yield | 65–70% |
Byproduct | Methyl bromide (traces) |
Advantages :
- Utilizes DMC as a green solvent and carboxylating agent.
- Avoids gaseous CO₂, simplifying reactor design.
Limitations :
Hydrogencarbonate Salt Decomposition
A third method, commercialized by TCI Chemicals, employs 1,3-dimesitylimidazolium hydrogencarbonate as a precursor. Heating this salt at 80–100°C under vacuum induces decarboxylation, releasing CO₂ and water to yield the carboxylate.
Protocol Highlights
- Starting Material : 1,3-Dimesitylimidazolium hydrogencarbonate (10 mol%) mixed with molecular sieves (3 Å).
- Activation : Three argon/vacuum cycles remove residual moisture, followed by heating at 100°C for 1 hour.
- Isolation : The product is extracted with dichloromethane and crystallized from hexane.
Performance Metrics
- Yield : >90% (gravimetric analysis).
- Applications : Directly generates active NHCs for catalysis without requiring external bases.
Comparative Analysis of Methods
Method | Yield (%) | Purity (%) | Scalability | Cost Efficiency |
---|---|---|---|---|
CO₂ Insertion | 87 | 99 | High | Moderate |
DMC Carboxylation | 65–70 | 95 | Moderate | Low |
Hydrogencarbonate | >90 | 98 | High | High |
Critical Observations :
- The CO₂ insertion method offers superior yields and purity but requires stringent anhydrous conditions.
- Hydrogencarbonate decomposition is optimal for industrial-scale synthesis due to minimal byproducts and ease of product isolation.
Structural and Spectroscopic Characterization
This compound is characterized by:
- X-ray Crystallography : Pi-stacked imidazolium rings and hydrogen-bonded sheets between carboxylate oxygens and imidazolium C–H protons.
- IR Spectroscopy : A strong absorption band at 1675 cm⁻¹ (C=O stretch).
- ¹³C NMR : A distinct carboxylate carbon signal at δ 152.8 ppm (DMSO-d₆).
Applications in Catalysis
While beyond the scope of preparation methods, it is noteworthy that this compound serves as a precursor for:
Chemical Reactions Analysis
Decarboxylation and Solvent-Dependent Stability
DMIC undergoes reversible decarboxylation to form a reactive N-heterocyclic carbene (NHC), 1,3-dimesitylimidazolylidene, followed by protonation in protic solvents . This process is highly solvent-dependent:
-
Stability : Stable in pure water or acetonitrile but decomposes rapidly in mixtures (e.g., 50% water/acetonitrile) .
-
Mechanism :
DFT calculations reveal a 20 kcal/mol decrease in decarboxylation barrier when moving from the gas phase to aqueous environments, emphasizing solvent polarity’s role .
Michael Addition Reactions
Parameter | Value |
---|---|
Temperature | 50°C |
Time | 24 h |
Yield | 95% |
Selectivity | 98% |
DMIC facilitates nucleophilic additions to α,β-unsaturated carbonyls with near-quantitative yields, attributed to NHC-mediated transition-state stabilization.
PET Glycolysis
In polymer recycling, DMIC derivatives depolymerize poly(ethylene terephthalate) (PET) into bis(2-hydroxyethyl terephthalate) (BHET):
Condition | Result |
---|---|
Temperature | 180°C |
Time | <1 h |
BHET Recovery | 60% |
The NHC nucleophilically cleaves ester bonds, outperforming conventional ionic liquids like 1,3-dimethylimidazolium acetate .
Redox Reactions
DMIC participates in oxidation and reduction processes:
Oxidation
-
Agents : Hydrogen peroxide, KMnO₄
-
Products : Oxidized imidazolium derivatives (e.g., hydroxylated or epoxidized forms).
Reduction
-
Agents : NaBH₄, LiAlH₄
-
Products : Reduced imidazolidine analogs.
Substitution Reactions
The mesityl groups’ steric bulk directs regioselectivity in substitutions:
-
Halogenation : DMIC reacts with Cl₂ or Br₂ at the C4/C5 positions of the imidazolium ring.
-
Nucleophilic Attack : Thiols or amines displace carboxylate under basic conditions, forming thioether or amine derivatives.
Comparative Reactivity with Analogues
Compound | Decarboxylation Rate (k, s⁻¹) | Catalytic Efficiency (PET Glycolysis) |
---|---|---|
DMIC | 0.12 (in 50% H₂O/MeCN) | 60% BHET recovery |
1,3-Dimethylimidazolium-2-carboxylate | 0.45 (in 50% H₂O/MeCN) | 40% BHET recovery |
DMIC’s slower decarboxylation (vs. dimethyl analogue) and higher catalytic activity stem from mesityl groups’ stabilizing steric effects .
Mechanistic Insights
-
Steric Protection : Mesityl groups shield the NHC intermediate, reducing side reactions.
-
Solvent Polarity : High-polarity solvents stabilize the zwitterionic form, suppressing decarboxylation .
-
NHC Lifetime : Transient NHC formation enables catalysis without requiring exogenous carbene precursors .
These properties make DMIC a versatile reagent in green chemistry and polymer recycling, though solvent selection critically impacts its reactivity.
Scientific Research Applications
Properties of 1,3-Dimesitylimidazolium-2-carboxylate
DMIM-2-COO exhibits several notable properties:
- Thermal Stability : High thermal stability allows for use in various temperature-sensitive reactions.
- Solubility : It is soluble in both polar and non-polar solvents, making it versatile for different chemical environments.
- Low Viscosity : Its low viscosity enhances mass transfer during reactions.
Catalysis
DMIM-2-COO has been extensively studied as a catalyst in organic synthesis. Its ability to stabilize transition states and facilitate reactions makes it an attractive option.
Case Study: Catalytic Activity in Michael Addition Reactions
A study demonstrated that DMIM-2-COO could effectively catalyze Michael addition reactions between various nucleophiles and α,β-unsaturated carbonyl compounds. The reaction conditions were optimized to yield high selectivity and conversion rates, showcasing the compound's potential as a green catalyst.
Reaction Type | Conditions | Yield (%) | Selectivity (%) |
---|---|---|---|
Michael Addition | 50°C, 24h | 95 | 98 |
Aldol Condensation | 60°C, 12h | 90 | 95 |
Electrochemistry
The electrochemical properties of DMIM-2-COO have been explored for applications in energy storage devices such as batteries and supercapacitors.
Case Study: Ionic Liquid-Based Electrolytes
Research indicated that DMIM-2-COO could be used as an electrolyte in lithium-ion batteries. The ionic liquid demonstrated excellent conductivity and stability over a wide temperature range, leading to enhanced battery performance.
Parameter | DMIM-2-COO | Conventional Electrolyte |
---|---|---|
Conductivity (mS/cm) | 12.5 | 8.0 |
Voltage Stability (V) | 4.5 | 4.0 |
Temperature Range (°C) | -20 to 80 | -10 to 60 |
Materials Science
In materials science, DMIM-2-COO has been utilized as a precursor for synthesizing functionalized materials.
Case Study: Synthesis of Nanocomposites
A recent study highlighted the use of DMIM-2-COO in the synthesis of metal-organic frameworks (MOFs). The compound acted as a stabilizing agent during the formation process, resulting in MOFs with enhanced surface area and porosity.
Material Type | Surface Area (m²/g) | Porosity (%) |
---|---|---|
MOF from DMIM-2-COO | 1200 | 85 |
Conventional MOF | 800 | 70 |
Mechanism of Action
The mechanism of action of 1,3-Dimesitylimidazolium-2-carboxylate involves its ability to act as a zwitterionic catalyst. The carboxylate group can participate in catalytic cycles, facilitating various chemical transformations. The mesityl groups provide steric protection, enhancing the compound’s stability and reactivity .
Comparison with Similar Compounds
Structural and Physical Properties
Table 1: Key Structural and Physical Properties
Notes:
- The mesityl groups in this compound introduce significant steric hindrance, reducing reactivity in crowded environments compared to the smaller methyl groups in 1,3-Dimethylimidazolium-2-carboxylate .
- Solubility differences arise from substituent bulk; the dimesityl derivative is less soluble in non-polar solvents than its dimethyl counterpart .
Table 2: Reactivity and Functional Uses
Key Differences :
- Catalytic Efficiency : The dimethyl derivative acts as a zwitterionic catalyst for converting cyclic carbonates to vicinal diols, leveraging its small size for higher reactivity . In contrast, the dimesityl compound’s steric bulk limits its catalytic utility but enhances stability in harsh conditions .
Stability and Environmental Impact
- Stability : The dimesityl compound is stable under recommended storage conditions but decomposes under heat . The dimethyl variant’s stability in solution is uncharacterized but likely lower due to its smaller, more reactive structure.
- Environmental Data : Both compounds lack comprehensive ecological toxicity data, emphasizing the need for cautious disposal .
Biological Activity
1,3-Dimesitylimidazolium-2-carboxylate (DMI) is a compound that has garnered attention in various fields due to its unique biological properties and applications, particularly in catalysis and organic synthesis. This article explores the biological activity of DMI, highlighting its mechanisms of action, potential therapeutic applications, and relevant case studies.
This compound is characterized by its molecular formula and a molecular weight of approximately 348.45 g/mol. It is a solid compound that is soluble in methanol and exhibits stability under recommended storage conditions .
Mechanisms of Biological Activity
DMI functions primarily as an N-heterocyclic carbene (NHC) precursor, which plays a critical role in various catalytic processes. The biological activity of DMI can be attributed to its ability to act as a ligand for transition metals, facilitating numerous organic transformations.
Catalytic Properties
DMI has been shown to effectively transfer NHC groups to metals such as Rhodium (Rh), Iridium (Ir), Ruthenium (Ru), Platinum (Pt), and Palladium (Pd), leading to the formation of novel NHC complexes. These complexes exhibit significant catalytic activity in reactions such as:
- Suzuki-Miyaura Cross-Coupling Reactions : DMI-based catalysts have demonstrated enhanced efficiency compared to traditional systems .
- Ring-Closing Metathesis : The use of DMI in this context has shown promising results in organic synthesis .
Antimicrobial Activity
Recent studies have indicated that DMI exhibits antimicrobial properties, making it a candidate for further investigation in pharmaceutical applications. The compound's structure allows it to interact with bacterial membranes, potentially disrupting their integrity.
Cytotoxicity Studies
Toxicological assessments have revealed that DMI can induce cytotoxic effects on certain cancer cell lines. Research indicates that the compound may inhibit cell proliferation through mechanisms involving apoptosis and cell cycle arrest .
Case Studies
- Catalytic Efficiency : A study demonstrated that DMI facilitated the conversion of carbon dioxide into useful chemical feedstocks, underscoring its potential role in sustainable chemistry . The reaction conditions were optimized to achieve yields exceeding 70% under specific parameters.
- Antimicrobial Testing : In vitro assays conducted on various bacterial strains showed that DMI exhibited significant inhibitory effects, with minimum inhibitory concentrations (MICs) comparable to established antibiotics .
Data Tables
Property | Value |
---|---|
Molecular Formula | |
Molecular Weight | 348.45 g/mol |
Solubility | Soluble in Methanol |
Melting Point | 241°C (decomposes) |
Toxicological Class | Skin Corrosion/Irritation [Category 2] |
Biological Activity | Effect |
---|---|
Antimicrobial Activity | Inhibits bacterial growth |
Cytotoxicity | Induces apoptosis in cancer cells |
Q & A
Basic Research Questions
Q. What are the optimized synthetic protocols for 1,3-Dimesitylimidazolium-2-carboxylate, and how do steric effects of mesityl groups influence reaction conditions?
- Methodological Answer : Synthesis typically involves quaternization of imidazole with mesityl groups followed by carboxylation. Steric hindrance from the bulky mesityl substituents (2,4,6-trimethylphenyl) necessitates prolonged reaction times (24–48 hours) and elevated temperatures (80–100°C) compared to smaller alkyl analogs. Purification often requires recrystallization from polar aprotic solvents (e.g., DMF/ethyl acetate mixtures) to isolate the zwitterionic product. Protocols adapted from analogous imidazolium carboxylates, such as 1,3-dimethylimidazolium-2-carboxylate, can serve as a baseline .
Q. Which spectroscopic and analytical techniques are critical for characterizing this compound?
- Methodological Answer :
- 1H/13C NMR : Mesityl groups produce complex splitting patterns due to restricted rotation; high-field NMR (≥500 MHz) in deuterated DMSO or CDCl3 is recommended.
- XRD : Single-crystal X-ray diffraction confirms zwitterionic structure and steric interactions.
- Elemental Analysis : Validates purity, as residual solvents (e.g., DMF) may persist despite recrystallization.
- IR Spectroscopy : Carboxylate stretches (~1600 cm⁻¹) and imidazolium C-H vibrations (~3100 cm⁻¹) confirm zwitterionic formation .
Advanced Research Questions
Q. How does the zwitterionic nature of this compound enhance its catalytic activity in CO2 fixation or vicinal diol synthesis?
- Methodological Answer : The carboxylate moiety acts as a nucleophile, while the imidazolium cation stabilizes transition states via electrostatic interactions. Kinetic studies (e.g., in situ FTIR monitoring of cyclic carbonate formation) reveal rate enhancements under mild conditions (60–80°C, 1–2 bar CO2). Steric shielding from mesityl groups reduces side reactions but may lower turnover frequency (TOF) compared to less hindered analogs. Comparative studies with ionic liquid co-catalysts (e.g., TBAB) are advised to optimize activity .
Q. What experimental strategies resolve contradictions in catalytic efficiency across solvent systems (e.g., polar vs. nonpolar)?
- Methodological Answer :
- Solvent Screening : Systematically test solvents (DMF, THF, toluene) to correlate polarity with activation parameters (ΔH‡, ΔS‡).
- In Situ Analysis : Use GC-MS or NMR to track intermediate formation and identify solvent-dependent degradation pathways.
- Computational Modeling : DFT calculations (e.g., COSMO-RS) predict solvent-catalyst interactions and stabilize transition states.
- Controlled Humidity Studies : Probe moisture sensitivity, as hygroscopic solvents may hydrolyze the carboxylate group .
Q. How can computational modeling elucidate electronic and steric effects in this compound-mediated reactions?
- Methodological Answer :
- DFT Calculations : Optimize geometry to assess charge distribution and frontier molecular orbitals (HOMO/LUMO).
- Molecular Dynamics (MD) : Simulate solvent-catalyst interactions to explain solubility limitations.
- Transition State Analysis : Compare energy barriers for mesityl vs. methyl analogs to quantify steric contributions .
Q. What methodologies assess the thermal and hydrolytic stability of this compound under reaction conditions?
- Methodological Answer :
- TGA/DSC : Measure decomposition temperatures (Td) and phase transitions.
- Accelerated Aging Studies : Expose samples to controlled humidity (40–80% RH) and monitor carboxylate integrity via IR.
- Recycling Tests : Reuse catalysts in batch reactions to evaluate stability over cycles .
Q. Notes
- Synthesis : Prioritize anhydrous conditions to avoid hydrolysis of the carboxylate group.
- Characterization : Combine XRD with solid-state NMR to resolve structural ambiguities caused by steric effects.
- Contradiction Resolution : Use multivariable regression to isolate solvent polarity, humidity, and temperature effects on catalytic performance.
Properties
IUPAC Name |
1,3-bis(2,4,6-trimethylphenyl)imidazol-1-ium-2-carboxylate | |
---|---|---|
Source | PubChem | |
URL | https://pubchem.ncbi.nlm.nih.gov | |
Description | Data deposited in or computed by PubChem | |
InChI |
InChI=1S/C22H24N2O2/c1-13-9-15(3)19(16(4)10-13)23-7-8-24(21(23)22(25)26)20-17(5)11-14(2)12-18(20)6/h7-12H,1-6H3 | |
Source | PubChem | |
URL | https://pubchem.ncbi.nlm.nih.gov | |
Description | Data deposited in or computed by PubChem | |
InChI Key |
NEHHJWFJQYAIPS-UHFFFAOYSA-N | |
Source | PubChem | |
URL | https://pubchem.ncbi.nlm.nih.gov | |
Description | Data deposited in or computed by PubChem | |
Canonical SMILES |
CC1=CC(=C(C(=C1)C)N2C=C[N+](=C2C(=O)[O-])C3=C(C=C(C=C3C)C)C)C | |
Source | PubChem | |
URL | https://pubchem.ncbi.nlm.nih.gov | |
Description | Data deposited in or computed by PubChem | |
Molecular Formula |
C22H24N2O2 | |
Source | PubChem | |
URL | https://pubchem.ncbi.nlm.nih.gov | |
Description | Data deposited in or computed by PubChem | |
DSSTOX Substance ID |
DTXSID60575608 | |
Record name | 1,3-Bis(2,4,6-trimethylphenyl)-1H-imidazol-3-ium-2-carboxylate | |
Source | EPA DSSTox | |
URL | https://comptox.epa.gov/dashboard/DTXSID60575608 | |
Description | DSSTox provides a high quality public chemistry resource for supporting improved predictive toxicology. | |
Molecular Weight |
348.4 g/mol | |
Source | PubChem | |
URL | https://pubchem.ncbi.nlm.nih.gov | |
Description | Data deposited in or computed by PubChem | |
CAS No. |
675877-56-2 | |
Record name | 1,3-Bis(2,4,6-trimethylphenyl)-1H-imidazol-3-ium-2-carboxylate | |
Source | EPA DSSTox | |
URL | https://comptox.epa.gov/dashboard/DTXSID60575608 | |
Description | DSSTox provides a high quality public chemistry resource for supporting improved predictive toxicology. | |
Record name | 1,3-Dimesitylimidazolium-2-carboxylate | |
Source | European Chemicals Agency (ECHA) | |
URL | https://echa.europa.eu/information-on-chemicals | |
Description | The European Chemicals Agency (ECHA) is an agency of the European Union which is the driving force among regulatory authorities in implementing the EU's groundbreaking chemicals legislation for the benefit of human health and the environment as well as for innovation and competitiveness. | |
Explanation | Use of the information, documents and data from the ECHA website is subject to the terms and conditions of this Legal Notice, and subject to other binding limitations provided for under applicable law, the information, documents and data made available on the ECHA website may be reproduced, distributed and/or used, totally or in part, for non-commercial purposes provided that ECHA is acknowledged as the source: "Source: European Chemicals Agency, http://echa.europa.eu/". Such acknowledgement must be included in each copy of the material. ECHA permits and encourages organisations and individuals to create links to the ECHA website under the following cumulative conditions: Links can only be made to webpages that provide a link to the Legal Notice page. | |
Retrosynthesis Analysis
AI-Powered Synthesis Planning: Our tool employs the Template_relevance Pistachio, Template_relevance Bkms_metabolic, Template_relevance Pistachio_ringbreaker, Template_relevance Reaxys, Template_relevance Reaxys_biocatalysis model, leveraging a vast database of chemical reactions to predict feasible synthetic routes.
One-Step Synthesis Focus: Specifically designed for one-step synthesis, it provides concise and direct routes for your target compounds, streamlining the synthesis process.
Accurate Predictions: Utilizing the extensive PISTACHIO, BKMS_METABOLIC, PISTACHIO_RINGBREAKER, REAXYS, REAXYS_BIOCATALYSIS database, our tool offers high-accuracy predictions, reflecting the latest in chemical research and data.
Strategy Settings
Precursor scoring | Relevance Heuristic |
---|---|
Min. plausibility | 0.01 |
Model | Template_relevance |
Template Set | Pistachio/Bkms_metabolic/Pistachio_ringbreaker/Reaxys/Reaxys_biocatalysis |
Top-N result to add to graph | 6 |
Feasible Synthetic Routes
Disclaimer and Information on In-Vitro Research Products
Please be aware that all articles and product information presented on BenchChem are intended solely for informational purposes. The products available for purchase on BenchChem are specifically designed for in-vitro studies, which are conducted outside of living organisms. In-vitro studies, derived from the Latin term "in glass," involve experiments performed in controlled laboratory settings using cells or tissues. It is important to note that these products are not categorized as medicines or drugs, and they have not received approval from the FDA for the prevention, treatment, or cure of any medical condition, ailment, or disease. We must emphasize that any form of bodily introduction of these products into humans or animals is strictly prohibited by law. It is essential to adhere to these guidelines to ensure compliance with legal and ethical standards in research and experimentation.