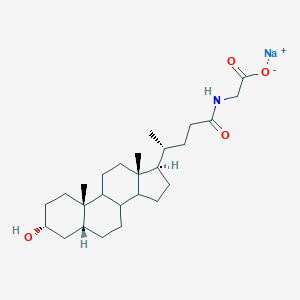
Glycolithocholic acid sodium salt
Overview
Description
Glycolithocholic acid sodium salt is a bile acid that plays a crucial role in cholesterol metabolism . It promotes the synthesis of bile acids within the liver, which are subsequently released into the small intestine to aid in the absorption of dietary lipids . It is a conjugate of cholic acid and glycine .
Synthesis Analysis
The microbiota produces Glycolithocholic acid sodium salt in the small intestine by the catalysis of enzymes . In the mode of low-energy collision-induced dissociation, Maekawa et al. detected CA, CDCA, DCA, LCA and ursodeoxycholic acid (UDCA) and their conjugated products with glycine, taurine, C3-sulfuric acid, C3-glucuronic acid and C24-glucuronic acid .Molecular Structure Analysis
The molecular formula of Glycolithocholic acid sodium salt is C26H42NNaO4 . Its average mass is 455.606 Da and its monoisotopic mass is 455.301147 Da .Chemical Reactions Analysis
Glycolithocholic acid sodium salt is a metabolite of glycolithocholic acid . It is formed from glycolithocholic acid by hepatocytes .Physical And Chemical Properties Analysis
Glycolithocholic acid sodium salt is soluble in most organic solvents and in water . Its melting point is 201.3-207.4°C .Scientific Research Applications
Endocrinology & Metabolism
Glycolithocholic acid is a glycine-conjugated form of the secondary bile acid lithocholic acid . It plays a significant role in endocrinology and metabolism, particularly in metabolic diseases and obesity .
Results or Outcomes
While specific results or outcomes can vary greatly depending on the context of the research, studies have shown that glycolithocholic acid levels can increase with age in children . It’s also been found to be increased in the liver of mice fed a diet supplemented with ursodeoxycholic acid .
Synthesis of Sulfate Esters
Glycolithocholic acid sodium salt is used in the synthesis of sulfate esters of lithocholic acid, glycolithocholic acid, and taurolithocholic acid .
Method of Application
The synthesis involves reacting lithocholic acid or glycolithocholic acid with sulfur trioxide-triethylamine complex in dimethylformamide for 0.5-1 hr . Taurolithocholic acid sulfate is obtained by conjugating lithocholic acid sulfate with taurine in dimethylformamide at 90°C for 0.5 hr .
Results or Outcomes
The procedure produced the desired products in over 90% yield . This synthesis method is significant as sulfation plays an important role in the metabolism of bile acids .
Biomarker for Disease Severity in COVID-19 Patients
Glycolithocholic Acid 3-Sulfate has been studied as a potential serum biomarker for disease severity and mortality in COVID-19 patients .
Method of Application
The specific methods of application or experimental procedures would involve mass spectrometry approaches .
Results or Outcomes
While the specific results or outcomes are not detailed in the source, the study suggests potential applications of Glycolithocholic Acid 3-Sulfate in understanding and managing COVID-19 .
Obesity Research
Glycolithocholic acid is used in obesity research . It’s a glycine-conjugated form of the secondary bile acid lithocholic acid .
Results or Outcomes
While specific results or outcomes can vary greatly depending on the context of the research, studies have shown that glycolithocholic acid levels can increase with age in children . It’s also been found to be increased in the liver of mice fed a diet supplemented with ursodeoxycholic acid .
Metabolic Diseases Research
Glycolithocholic acid is used in the research of metabolic diseases . It’s a glycine-conjugated form of the secondary bile acid lithocholic acid .
Results or Outcomes
While specific results or outcomes can vary greatly depending on the context of the research, studies have shown that glycolithocholic acid levels can increase with age in children . It’s also been found to be increased in the liver of mice fed a diet supplemented with ursodeoxycholic acid .
Role in Metabolic Regulation
Glycolithocholic acid plays a significant role in metabolic regulation . It’s a glycine-conjugated form of the secondary bile acid lithocholic acid .
Results or Outcomes
Studies have shown that glycolithocholic acid levels can increase with age in children . It’s also been found to be increased in the liver of mice fed a diet supplemented with ursodeoxycholic acid .
Safety And Hazards
Avoid dust formation. Avoid breathing mist, gas or vapours. Avoid contacting with skin and eye. Use personal protective equipment. Wear chemical impermeable gloves. Ensure adequate ventilation. Remove all sources of ignition. Evacuate personnel to safe areas. Keep people away from and upwind of spill/leak .
properties
IUPAC Name |
sodium;2-[[(4R)-4-[(3R,5R,8R,9S,10S,13R,14S,17R)-3-hydroxy-10,13-dimethyl-2,3,4,5,6,7,8,9,11,12,14,15,16,17-tetradecahydro-1H-cyclopenta[a]phenanthren-17-yl]pentanoyl]amino]acetate | |
---|---|---|
Source | PubChem | |
URL | https://pubchem.ncbi.nlm.nih.gov | |
Description | Data deposited in or computed by PubChem | |
InChI |
InChI=1S/C26H43NO4.Na/c1-16(4-9-23(29)27-15-24(30)31)20-7-8-21-19-6-5-17-14-18(28)10-12-25(17,2)22(19)11-13-26(20,21)3;/h16-22,28H,4-15H2,1-3H3,(H,27,29)(H,30,31);/q;+1/p-1/t16-,17-,18-,19+,20-,21+,22+,25+,26-;/m1./s1 | |
Source | PubChem | |
URL | https://pubchem.ncbi.nlm.nih.gov | |
Description | Data deposited in or computed by PubChem | |
InChI Key |
LQKBJAKZKFBLIB-LGURPPGFSA-M | |
Source | PubChem | |
URL | https://pubchem.ncbi.nlm.nih.gov | |
Description | Data deposited in or computed by PubChem | |
Canonical SMILES |
CC(CCC(=O)NCC(=O)[O-])C1CCC2C1(CCC3C2CCC4C3(CCC(C4)O)C)C.[Na+] | |
Source | PubChem | |
URL | https://pubchem.ncbi.nlm.nih.gov | |
Description | Data deposited in or computed by PubChem | |
Isomeric SMILES |
C[C@H](CCC(=O)NCC(=O)[O-])[C@H]1CC[C@@H]2[C@@]1(CC[C@H]3[C@H]2CC[C@H]4[C@@]3(CC[C@H](C4)O)C)C.[Na+] | |
Source | PubChem | |
URL | https://pubchem.ncbi.nlm.nih.gov | |
Description | Data deposited in or computed by PubChem | |
Molecular Formula |
C26H42NNaO4 | |
Source | PubChem | |
URL | https://pubchem.ncbi.nlm.nih.gov | |
Description | Data deposited in or computed by PubChem | |
Molecular Weight |
455.6 g/mol | |
Source | PubChem | |
URL | https://pubchem.ncbi.nlm.nih.gov | |
Description | Data deposited in or computed by PubChem | |
Product Name |
Glycolithocholic acid sodium salt |
Retrosynthesis Analysis
AI-Powered Synthesis Planning: Our tool employs the Template_relevance Pistachio, Template_relevance Bkms_metabolic, Template_relevance Pistachio_ringbreaker, Template_relevance Reaxys, Template_relevance Reaxys_biocatalysis model, leveraging a vast database of chemical reactions to predict feasible synthetic routes.
One-Step Synthesis Focus: Specifically designed for one-step synthesis, it provides concise and direct routes for your target compounds, streamlining the synthesis process.
Accurate Predictions: Utilizing the extensive PISTACHIO, BKMS_METABOLIC, PISTACHIO_RINGBREAKER, REAXYS, REAXYS_BIOCATALYSIS database, our tool offers high-accuracy predictions, reflecting the latest in chemical research and data.
Strategy Settings
Precursor scoring | Relevance Heuristic |
---|---|
Min. plausibility | 0.01 |
Model | Template_relevance |
Template Set | Pistachio/Bkms_metabolic/Pistachio_ringbreaker/Reaxys/Reaxys_biocatalysis |
Top-N result to add to graph | 6 |
Feasible Synthetic Routes
Citations
Disclaimer and Information on In-Vitro Research Products
Please be aware that all articles and product information presented on BenchChem are intended solely for informational purposes. The products available for purchase on BenchChem are specifically designed for in-vitro studies, which are conducted outside of living organisms. In-vitro studies, derived from the Latin term "in glass," involve experiments performed in controlled laboratory settings using cells or tissues. It is important to note that these products are not categorized as medicines or drugs, and they have not received approval from the FDA for the prevention, treatment, or cure of any medical condition, ailment, or disease. We must emphasize that any form of bodily introduction of these products into humans or animals is strictly prohibited by law. It is essential to adhere to these guidelines to ensure compliance with legal and ethical standards in research and experimentation.