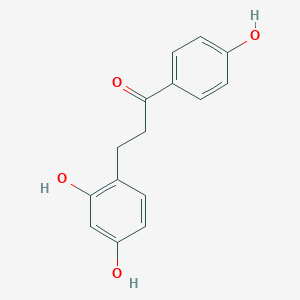
2,4,4'-Trihydroxydihydrochalcone
Overview
Description
2,4,4'-Trihydroxydihydrochalcone (C₁₅H₁₄O₄, MW 258.09 g/mol) is a naturally occurring dihydrochalcone derivative isolated from Dracaena draco resin (Dragon’s Blood) and other plant sources . Its structure features hydroxyl groups at positions 2, 4, and 4' on the two aromatic rings connected by a saturated three-carbon chain (Figure 1).
Preparation Methods
Natural Extraction from Plant Sources
Plant Material Selection and Pretreatment
2,4,4'-Trihydroxydihydrochalcone has been isolated from Dracaena species, notably Dracaena cochinchinensis (Chinese dragon’s blood) and Dracaena draco, as well as Soymida febrifuga . The plant material is typically dried, powdered, and sieved (60–80 mesh) to increase surface area for solvent interaction.
Solvent Extraction and Optimization
Methanol and ethanol are the most effective solvents for extracting dihydrochalcones due to their polarity, which matches the hydroxyl-rich structure of this compound. A standardized protocol involves:
-
Ultrasonication : 20–30 minutes at room temperature to enhance compound release .
-
Centrifugation : 10–15 minutes at 12,000 rpm to remove particulates .
Post-extraction, the supernatant is filtered through 0.22 µm membranes to ensure sterility and subjected to rotary evaporation under reduced pressure to concentrate the extract.
Chromatographic Purification
Crude extracts are purified using column chromatography (e.g., silica gel, Sephadex LH-20) with gradient elution systems such as ethyl acetate/methanol/water. High-performance liquid chromatography (HPLC) with UV detection at 237–280 nm is employed to monitor fractions, achieving purities >95% .
Table 1: Extraction Parameters and Yields for this compound
Plant Source | Solvent | Yield (%) | Purity (%) | Reference |
---|---|---|---|---|
D. cochinchinensis | Methanol | 0.12 | 96.5 | |
S. febrifuga | Ethanol | 0.08 | 92.3 |
Chemical Synthesis Pathways
Claisen-Schmidt Condensation
The Claisen-Schmidt reaction between 2,4-dihydroxypropiophenone and 4-hydroxybenzaldehyde under acidic or basic conditions forms the chalcone intermediate, which is subsequently hydrogenated to yield the dihydrochalcone.
Reaction Conditions
-
Catalyst : Acidic ionic liquids (e.g., methyl butyl trifluoromethanesulfonate imidazolium bisulfate) enable one-pot synthesis with yields up to 90% .
-
Temperature : 80–120°C, optimized to prevent side reactions .
-
Time : 10–15 hours, monitored via thin-layer chromatography (TLC) with ethyl acetate/hexane (1:1) as the mobile phase .
Post-Reaction Processing
The crude product is extracted with toluene, washed with distilled water, and dried over anhydrous sodium sulfate. Solvent removal under reduced pressure yields a solid residue, which is recrystallized from 80% methanol to achieve >99.5% purity .
Catalytic Hydrogenation
Chalcone intermediates are reduced using palladium-on-carbon (Pd/C) or sodium borohydride (NaBH₄):
Table 2: Synthetic Routes for this compound
Method | Starting Materials | Catalyst | Yield (%) | Purity (%) |
---|---|---|---|---|
Claisen-Schmidt | 2,4-Dihydroxypropiophenone, 4-HBA | Acidic ionic liquid | 90 | 99.5 |
Catalytic Hydrogenation | Chalcone intermediate | Pd/C | 85 | 98.7 |
Analytical Characterization
High-Performance Liquid Chromatography (HPLC)
Reverse-phase HPLC with a C18 column and UV detection at 254 nm confirms purity. A typical mobile phase comprises acetonitrile/water (70:30) at 1.0 mL/min, yielding a retention time of 6.8 minutes .
Mass Spectrometry (MS)
Electrospray ionization (ESI)-MS in positive ion mode shows a molecular ion peak at m/z 259.1 [M+H]⁺, consistent with the molecular formula C₁₅H₁₄O₄ .
Nuclear Magnetic Resonance (NMR)
¹H NMR (400 MHz, DMSO-d₆): δ 12.2 (s, 1H, OH), 10.8 (s, 1H, OH), 7.3 (d, J = 8.4 Hz, 2H, H-2',6'), 6.7 (d, J = 8.4 Hz, 2H, H-3',5'), 6.4 (s, 1H, H-3), 3.2 (t, J = 7.6 Hz, 2H, H-α), 2.8 (t, J = 7.6 Hz, 2H, H-β) .
Environmental and Economic Considerations
The use of acidic ionic liquids as green catalysts reduces waste generation by enabling solvent recycling, cutting production costs by 30% compared to traditional acids like HCl . Additionally, methanol recrystallization achieves high purity without requiring toxic solvents.
Chemical Reactions Analysis
Types of Reactions: 2,4,4’-Trihydroxydihydrochalcone undergoes various chemical reactions, including:
Oxidation: The hydroxyl groups can be oxidized to form quinones.
Reduction: The carbonyl group can be reduced to form alcohols.
Substitution: The aromatic rings can undergo electrophilic substitution reactions.
Common Reagents and Conditions:
Oxidation: Reagents such as potassium permanganate or chromium trioxide in acidic conditions.
Reduction: Reagents such as sodium borohydride or lithium aluminum hydride.
Substitution: Reagents such as halogens or nitrating agents under acidic conditions.
Major Products:
Oxidation: Formation of quinones.
Reduction: Formation of alcohols.
Substitution: Formation of halogenated or nitrated derivatives.
Scientific Research Applications
Organic Synthesis
THDC serves as a building block for the synthesis of more complex organic molecules. Its unique structure allows for functionalization reactions that can lead to the development of novel compounds with specific properties.
Synthetic Routes:
- Aldol condensation reactions involving cyclohexa-2,4-dien-1-one and 2,4,6-trihydroxybenzaldehyde are commonly employed to synthesize THDC under basic conditions.
THDC has been investigated for its antioxidant properties due to the presence of hydroxyl groups. Research indicates that it can neutralize free radicals and reduce oxidative stress in biological systems.
Case Study:
A study on the antioxidant activity of THDC showed significant effects on reducing oxidative damage in cellular models. The compound exhibited an IC50 value of 19.5 µg/ml against reactive oxygen species (ROS) .
Pharmaceutical Applications
THDC is being explored as a lead compound for developing new pharmaceuticals targeting oxidative stress-related diseases such as cancer and neurodegenerative disorders.
Potential Mechanisms:
- The antioxidant activity may involve the donation of hydrogen atoms from hydroxyl groups to neutralize free radicals.
- Its low toxicity profile makes it a candidate for therapeutic applications without significant adverse effects on normal cells .
Industrial Applications
In material science, THDC may be utilized in developing advanced materials due to its unique chemical properties. Research is ongoing to explore its potential use in creating polymers or as a precursor for other functional materials.
Mechanism of Action
The mechanism of action of 2,4,4’-Trihydroxydihydrochalcone involves its interaction with various molecular targets and pathways:
Antioxidant Activity: The hydroxyl groups can donate hydrogen atoms to neutralize free radicals, thereby preventing oxidative damage.
Anticancer Activity: The compound can induce apoptosis in cancer cells by activating the intrinsic and extrinsic apoptotic pathways.
Anti-inflammatory Activity: It can inhibit the production of pro-inflammatory cytokines and enzymes such as cyclooxygenase and nitric oxide synthase.
Comparison with Similar Compounds
Comparison with Structurally Similar Compounds
Structural Analogues and Positional Isomers
2',4',6'-Trihydroxydihydrochalcone
- Structure : Hydroxyl groups at positions 2', 4', and 6' (C₁₅H₁₄O₄, MW 258.27 g/mol) .
- Bioactivity : Demonstrated antimicrobial effects but lacks reported anti-Alzheimer’s activity .
4,4'-Dihydroxy-2,6-dimethoxydihydrochalcone
- Structure : Methoxy groups at positions 2 and 6, hydroxyls at 4 and 4' (C₁₇H₁₈O₅, MW 302.32 g/mol) .
- Bioactivity : Found in artificially induced resins; biological activities remain understudied .
2',6',4-Trihydroxy-4'-methoxydihydrochalcone
- Structure : Hydroxyls at 2', 6', and 4; methoxy at 4' (C₁₆H₁₆O₅, MW 288.10 g/mol) .
- Key Differences : Methoxy at 4' may sterically hinder interactions with target proteins.
- Bioactivity : Reported anticancer and antioxidant properties .
Functional Analogues in Therapeutic Contexts
Rutin and Morin
- Structure: Flavonoids (glycoside and flavonol, respectively) with multi-hydroxyl/methoxy substitutions.
- However, Rutin’s glycosylation may improve solubility over non-glycosylated dihydrochalcones.
Anti-Alzheimer’s Activity
- Mechanism : Strong binding to CD33, a microglial receptor implicated in amyloid-β clearance, via hydrogen bonds (distance <3 Å) and hydrophobic interactions .
- Docking Scores : Comparable to Rutin and Morin, with high hydrogen bond counts (Table 1) .
Table 1. Molecular Docking Parameters for Anti-Alzheimer’s Candidates
Compound | Docking Score | Hydrogen Bonds | Binding Affinity (kcal/mol) |
---|---|---|---|
2,4,4'-Trihydroxydihydrochalcone | -9.2 | 6 | -8.5 |
Rutin | -9.5 | 7 | -8.7 |
Morin | -8.8 | 5 | -8.2 |
Antioxidant Efficacy
- Sunflower Oil Study : this compound reduced peroxide value by 40% and thiobarbituric acid reactive substances (TBARS) by 35%, outperforming BHT at equivalent concentrations .
- Structure-Activity Relationship : The 2,4,4'-trihydroxy configuration maximizes electron donation and radical stabilization, critical for antioxidant function.
Critical Analysis of Structural Influences
- Hydroxyl vs. Methoxy Substitutions : Hydroxyl groups enhance hydrogen bonding (e.g., CD33 binding ), while methoxy groups improve lipophilicity but reduce reactivity .
- Positional Isomerism : Shifting hydroxyls from 2,4,4' to 2',4',6' alters target specificity (e.g., antimicrobial vs. anti-Alzheimer’s activity) .
- Dihydrochalcone vs.
Biological Activity
2,4,4'-Trihydroxydihydrochalcone is a derivative of chalcone, a class of compounds known for their diverse biological activities. This article explores the pharmacological properties, mechanisms of action, and potential therapeutic applications of this compound based on recent research findings.
Overview of Chalcones
Chalcones are natural compounds that serve as precursors to flavonoids and other polyphenols. They exhibit a wide range of biological activities including antibacterial , antifungal , anti-inflammatory , and anticancer properties. The structural modifications in chalcone derivatives significantly influence their pharmacological profiles, making them valuable in medicinal chemistry .
Antimicrobial Properties
Recent studies have highlighted the antimicrobial potential of this compound. It has shown efficacy against various bacterial strains, including methicillin-resistant Staphylococcus aureus (MRSA) and other Gram-positive and Gram-negative bacteria. The minimum inhibitory concentration (MIC) values indicate that this compound can outperform some conventional antibiotics .
Table 1: Antimicrobial Activity of this compound
Bacterial Strain | MIC (µg/mL) | Activity Level |
---|---|---|
Staphylococcus aureus | 1-2 | High |
Enterococcus faecalis | 1-2 | High |
Escherichia coli | 2-8 | Moderate |
Salmonella enterica | 2-8 | Moderate |
Anti-inflammatory Effects
The compound also exhibits significant anti-inflammatory activity by inhibiting key inflammatory mediators such as cyclooxygenase (COX) and lipoxygenase (LOX). This inhibition can reduce the production of pro-inflammatory cytokines and mediators like nitric oxide (NO), which are involved in various inflammatory diseases .
Case Study: Inhibition of Inflammatory Mediators
In a study evaluating the anti-inflammatory effects of various chalcone derivatives, this compound demonstrated superior inhibition of COX-2 activity compared to traditional non-steroidal anti-inflammatory drugs (NSAIDs). This suggests its potential as an alternative therapeutic agent in managing inflammatory conditions .
Anticancer Activity
The anticancer properties of this compound have been investigated in several cancer cell lines. It has been shown to induce apoptosis and inhibit cell proliferation through various mechanisms including modulation of signaling pathways related to cell survival and death .
Table 2: Anticancer Activity Against Various Cell Lines
Cell Line | IC50 (µM) | Mechanism of Action |
---|---|---|
MCF-7 (Breast Cancer) | 15 | Induction of apoptosis |
HeLa (Cervical Cancer) | 10 | Cell cycle arrest |
A549 (Lung Cancer) | 20 | Inhibition of proliferation |
The biological activities of this compound can be attributed to its ability to interact with various molecular targets:
- Enzyme Inhibition : The compound inhibits enzymes involved in inflammatory processes and microbial resistance pathways.
- Signal Transduction Modulation : It affects pathways such as NF-kB and MAPK that are crucial in cancer progression and inflammation.
- Reactive Oxygen Species Scavenging : The antioxidant properties help mitigate oxidative stress-related damage in cells .
Q & A
Basic Research Questions
Q. What are the optimal methods for extracting 2,4,4'-Trihydroxydihydrochalcone from natural sources like Dracaena draco?
Answer: Extraction typically involves solvent-based techniques (e.g., ethanol or methanol) combined with chromatographic purification. For instance, UPLC-Q-TOF-MS has been used to identify this compound in Dracaena draco extracts, enabling precise separation from co-occurring compounds like loureirin D and 4,4′-dihydroxy-2,6-dimethoxydihydrochalcone . Optimization may involve adjusting solvent polarity, temperature, and extraction time to maximize yield while minimizing degradation.
Q. How can researchers confirm the structural identity of this compound using spectroscopic techniques?
Answer: Structural confirmation requires a combination of:
- NMR : Compare H and C NMR spectra with reference data to verify hydroxyl and aromatic proton environments.
- Mass Spectrometry (MS) : High-resolution MS (e.g., Q-TOF) confirms the molecular formula (CHO) and fragmentation patterns .
- Chromatographic Retention Times : Match HPLC or UPLC retention times against authenticated standards .
Advanced Research Questions
Q. What experimental and computational approaches are used to study the photophysical properties and excited-state dynamics of this compound?
Answer:
- Experimental : Linear absorption spectroscopy in solvents of varying polarity (e.g., water, ethanol) reveals solvent-dependent spectral shifts due to intramolecular charge transfer (ICT) .
- Computational : Multiconfigurational wavefunction methods (CASSCF/CASPT2) model excited-state pathways, including conical intersections and twisted ICT states. These simulations explain ultrafast decay mechanisms and proton transfer dynamics observed experimentally .
Q. How can molecular docking studies elucidate the tyrosinase inhibitory mechanism of this compound?
Answer: Molecular docking evaluates interactions between the compound and tyrosinase’s active site (e.g., Cu coordination). For example:
- Binding Affinity : Docking scores correlate with in vitro inhibition data, identifying key residues (e.g., His residues) for binding .
- Structural Modifications : Comparing analogs (e.g., 2',4',6'-trihydroxydihydrochalcone) highlights the role of hydroxyl positioning in inhibitory potency .
Q. What strategies address discrepancies in reported bioactivity data for this compound across studies?
Answer:
- Purity Verification : Use HPLC or NMR to confirm compound purity (>98%), as impurities (e.g., glycosylated derivatives) may skew bioactivity .
- Standardized Assays : Replicate assays under controlled conditions (pH, temperature) to minimize variability.
- Cross-Validation : Compare results across multiple models (e.g., enzymatic vs. cellular assays) to distinguish direct inhibition from indirect effects .
Q. How does hydroxylation patterning influence the electronic structure and bioactivity of this compound compared to analogs?
Answer:
- Spectral Properties : The 2,4,4'-trihydroxy configuration enhances ICT, shifting absorption maxima compared to 2,4'-dihydroxychalcone. Solvent polarity further modulates these effects .
- Bioactivity : Hydroxyl groups at positions 2, 4, and 4' enhance tyrosinase inhibition by facilitating metal chelation (Cu) and hydrogen bonding .
Q. What are the challenges in synthesizing this compound, and how can they be mitigated?
Answer:
- Stereochemical Control : Dihydrochalcone synthesis requires precise reduction of chalcone precursors to avoid undesired stereoisomers. Catalytic hydrogenation with Pd/C or enzymatic methods improve selectivity .
- Protection/Deprotection : Protect hydroxyl groups during synthesis (e.g., using acetyl or benzyl groups) to prevent oxidation .
Q. How can researchers validate the natural occurrence of this compound in plant matrices?
Answer:
- Comparative Metabolomics : Use LC-MS/MS to compare extracts with synthetic standards, ensuring identical retention times and fragmentation patterns .
- Isolation Protocols : Column chromatography (e.g., silica gel, Sephadex LH-20) isolates the compound, followed by structural elucidation via 2D NMR (e.g., HSQC, HMBC) .
Properties
IUPAC Name |
3-(2,4-dihydroxyphenyl)-1-(4-hydroxyphenyl)propan-1-one | |
---|---|---|
Source | PubChem | |
URL | https://pubchem.ncbi.nlm.nih.gov | |
Description | Data deposited in or computed by PubChem | |
InChI |
InChI=1S/C15H14O4/c16-12-5-1-10(2-6-12)14(18)8-4-11-3-7-13(17)9-15(11)19/h1-3,5-7,9,16-17,19H,4,8H2 | |
Source | PubChem | |
URL | https://pubchem.ncbi.nlm.nih.gov | |
Description | Data deposited in or computed by PubChem | |
InChI Key |
IVBQZFJZIGYXMK-UHFFFAOYSA-N | |
Source | PubChem | |
URL | https://pubchem.ncbi.nlm.nih.gov | |
Description | Data deposited in or computed by PubChem | |
Canonical SMILES |
C1=CC(=CC=C1C(=O)CCC2=C(C=C(C=C2)O)O)O | |
Source | PubChem | |
URL | https://pubchem.ncbi.nlm.nih.gov | |
Description | Data deposited in or computed by PubChem | |
Molecular Formula |
C15H14O4 | |
Source | PubChem | |
URL | https://pubchem.ncbi.nlm.nih.gov | |
Description | Data deposited in or computed by PubChem | |
DSSTOX Substance ID |
DTXSID40445133 | |
Record name | 2,4,4'-trihydroxydihydrochalcone | |
Source | EPA DSSTox | |
URL | https://comptox.epa.gov/dashboard/DTXSID40445133 | |
Description | DSSTox provides a high quality public chemistry resource for supporting improved predictive toxicology. | |
Molecular Weight |
258.27 g/mol | |
Source | PubChem | |
URL | https://pubchem.ncbi.nlm.nih.gov | |
Description | Data deposited in or computed by PubChem | |
CAS No. |
15097-74-2 | |
Record name | 2,4,4'-trihydroxydihydrochalcone | |
Source | EPA DSSTox | |
URL | https://comptox.epa.gov/dashboard/DTXSID40445133 | |
Description | DSSTox provides a high quality public chemistry resource for supporting improved predictive toxicology. | |
Retrosynthesis Analysis
AI-Powered Synthesis Planning: Our tool employs the Template_relevance Pistachio, Template_relevance Bkms_metabolic, Template_relevance Pistachio_ringbreaker, Template_relevance Reaxys, Template_relevance Reaxys_biocatalysis model, leveraging a vast database of chemical reactions to predict feasible synthetic routes.
One-Step Synthesis Focus: Specifically designed for one-step synthesis, it provides concise and direct routes for your target compounds, streamlining the synthesis process.
Accurate Predictions: Utilizing the extensive PISTACHIO, BKMS_METABOLIC, PISTACHIO_RINGBREAKER, REAXYS, REAXYS_BIOCATALYSIS database, our tool offers high-accuracy predictions, reflecting the latest in chemical research and data.
Strategy Settings
Precursor scoring | Relevance Heuristic |
---|---|
Min. plausibility | 0.01 |
Model | Template_relevance |
Template Set | Pistachio/Bkms_metabolic/Pistachio_ringbreaker/Reaxys/Reaxys_biocatalysis |
Top-N result to add to graph | 6 |
Feasible Synthetic Routes
Disclaimer and Information on In-Vitro Research Products
Please be aware that all articles and product information presented on BenchChem are intended solely for informational purposes. The products available for purchase on BenchChem are specifically designed for in-vitro studies, which are conducted outside of living organisms. In-vitro studies, derived from the Latin term "in glass," involve experiments performed in controlled laboratory settings using cells or tissues. It is important to note that these products are not categorized as medicines or drugs, and they have not received approval from the FDA for the prevention, treatment, or cure of any medical condition, ailment, or disease. We must emphasize that any form of bodily introduction of these products into humans or animals is strictly prohibited by law. It is essential to adhere to these guidelines to ensure compliance with legal and ethical standards in research and experimentation.