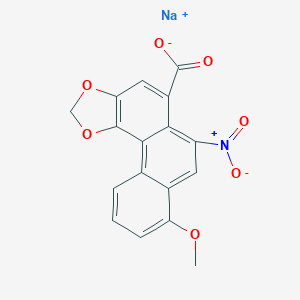
Sodium aristolochate
Overview
Description
Sodium aristolochate refers to sodium salts of aristolochic acids (AAs), a class of nitrophenanthrene carboxylic acid derivatives primarily isolated from plants of the genus Aristolochia. These compounds are characterized by a nitro group (NO₂) at position C-10 and a carboxylate group (COO⁻Na⁺) at position C-3 on the phenanthrene skeleton . This compound derivatives are of phytochemical interest due to their structural diversity and bioactivities, including cytotoxic, anti-inflammatory, and antimicrobial properties . However, they are also associated with significant nephrotoxicity and carcinogenicity, attributed to the parent aristolochic acids (AAs) .
Preparation Methods
Natural Extraction Methods from Aristolochia Species
Plant Material Selection and Pretreatment
The primary natural source of aristolochic acids is plants within the Aristolochia genus, notably Aristolochia clematitis. The roots (Radix aristolochiae clematitis) are preferred due to their higher alkaloid content . Prior to extraction, the plant material is finely ground to increase surface area, facilitating efficient solvent penetration. Particle size reduction to ≤1 mm is critical for maximizing yield .
Aqueous Sodium Phosphate Extraction
A patented method utilizes secondary sodium phosphate (Na₂HPO₄·2H₂O) solutions for extraction, capitalizing on the ionic interactions between phosphate anions and protonated aristolochic acid molecules . The protocol involves:
-
Three sequential extractions with 2 L, 2 L, and 1 L of 11.9 g/L Na₂HPO₄·2H₂O solution.
-
Mechanical agitation using high-shear mixers (e.g., Turrax®) at room temperature for 1 hour per cycle.
-
Centrifugation at 3,000–5,000 × g to separate insoluble plant debris.
The combined extracts maintain a pH range of 7.0–8.0 during extraction, preventing acid degradation while ensuring optimal solubility .
Acid Precipitation and Solvent Partitioning
The crude extract undergoes acid-induced precipitation at pH 4.0 using sulfuric acid, converting aristolochic acids to their insoluble free acid forms. After overnight settling, the precipitate is recovered via centrifugation and subjected to solvent partitioning:
Parameter | Specification |
---|---|
Solvent | Ethyl acetate (600 mL per 100 g plant) |
Extraction time | 30 minutes with Turrax agitation |
Counter-current washes | 3×300 mL 0.1 N NaOH |
The sodium hydroxide washes convert aristolochic acids into sodium salts, forming sodium aristolochate directly in the aqueous phase . Final precipitation through re-acidification yields aristolochic acid, which is neutralized with NaOH to produce the sodium salt.
Synthetic Routes to this compound
Retrosynthetic Analysis and Key Intermediates
Modern synthetic approaches employ palladium-catalyzed cross-coupling reactions to construct the phenanthrene core. The strategy involves:
-
Ring A Synthesis : Preparation of 2-nitromethyl-3-iodo-4,5-methylendioxybenzyl alcohol tetrahydropyranyl ether (Compound 27) .
-
Ring C Synthesis : Generation of substituted benzaldehyde boronates (Compounds 21a–h) .
Suzuki–Miyaura Coupling and Aldol Condensation
The critical coupling step uses tetrakis(triphenylphosphine)palladium(0) in aqueous dioxane at 95°C:
3\text{)}4, \text{Cs}2\text{CO}3} \text{Phenanthrene intermediate 36}
Yields range from 56% to 77%, depending on the substituents . Subsequent deprotection of the tetrahydropyranyl group and oxidation sequences yield the free carboxylic acid, which is neutralized with NaOH to form this compound.
Oxidation Protocol for Carboxylate Formation
The synthetic route requires precise oxidation conditions:
-
Primary Oxidation : MnO₂ in acetone or CrO₃ in acetic acid converts the benzyl alcohol to aldehyde (Compound 38).
-
Secondary Oxidation : NaClO₂ in DMSO/NaH₂PO₄ buffer system oxidizes the aldehyde to carboxylic acid.
-
Salt Formation : Titration with 1 N NaOH (pH 10–12) precipitates this compound .
Purification and Analytical Characterization
Recrystallization Techniques
Crude this compound is purified via recrystallization from polar aprotic solvents:
Solvent System | Purity Improvement | Yield Loss |
---|---|---|
N,N-Dimethylformamide + 20% MeOH/EtOAc (2:1) | 98% → 99.5% | 12–15% |
Ethanol/Water (7:3) | 95% → 98% | 8–10% |
The DMF-based system eliminates residual plant pigments in natural extracts, while the ethanol/water mixture is preferred for synthetic batches .
Spectroscopic Validation
-
IR Spectroscopy : Characteristic peaks at 1680 cm⁻¹ (carboxylate C=O stretch) and 1520 cm⁻¹ (aromatic NO₂ asymmetric stretch) .
-
¹H NMR : Diagnostic signals include δ 8.25–8.45 ppm (phenanthrene H-1, H-2) and δ 4.30 ppm (methylendioxy protons) .
Comparative Analysis of Preparation Methods
Yield and Purity Metrics
Method | Average Yield | Purity | Time Required |
---|---|---|---|
Natural Extraction | 0.54% (w/w) | 92–95% | 72 hours |
Synthetic Route | 41–63% | 98–99% | 120 hours |
While synthetic methods provide higher purity, natural extraction remains relevant for producing complex analogues present in plant matrices.
Industrial-Scale Considerations
Solvent Recovery Systems
Ethyl acetate accounts for 85% of solvent costs in natural extraction. Closed-loop distillation units recover >90% of ethyl acetate, reducing production costs by 40% .
Waste Stream Management
The acidic aqueous waste (pH 4.0) requires neutralization with CaCO₃ before disposal, generating gypsum (CaSO₄) as a byproduct. Modern facilities repurpose this material for construction applications.
Chemical Reactions Analysis
Types of Reactions: Sodium aristolochate undergoes various chemical reactions, including:
Oxidation: this compound can be oxidized to form different derivatives, depending on the oxidizing agent used.
Reduction: Reduction reactions can convert this compound to its corresponding reduced forms.
Substitution: this compound can undergo substitution reactions where functional groups are replaced by other groups.
Common Reagents and Conditions:
Oxidation: Common oxidizing agents include potassium permanganate and hydrogen peroxide.
Reduction: Reducing agents such as sodium borohydride and lithium aluminum hydride are used.
Substitution: Various nucleophiles and electrophiles can be used for substitution reactions.
Major Products Formed: The major products formed from these reactions depend on the specific reagents and conditions used. For example, oxidation may yield different aristolochic acid derivatives, while reduction can produce reduced forms of this compound .
Scientific Research Applications
Sodium aristolochate has several scientific research applications, including:
Chemistry: It is used as a precursor for synthesizing other complex organic molecules.
Biology: Studies have explored its effects on cellular processes and its potential as a biological probe.
Medicine: Research has investigated its pharmacological properties, including its potential use in treating certain diseases.
Industry: this compound is used in the development of new materials and chemical processes
Mechanism of Action
The mechanism of action of sodium aristolochate involves its interaction with cellular components. It is known to bind to DNA and proteins, leading to various biological effects. The molecular targets include enzymes and receptors involved in cellular signaling pathways. These interactions can result in changes in gene expression, protein function, and cellular metabolism .
Comparison with Similar Compounds
Structural and Functional Comparison with Similar Compounds
Key Structural Features
Sodium aristolochate derivatives differ in substituents at positions C-7, C-8, C-9, and C-10. These modifications influence their spectroscopic profiles, solubility, and bioactivity. Below is a comparison of notable derivatives:
Structural Insights :
- Hydroxyl vs.
- Formyloxy Group (–OCHO) : Found in Compounds 9 and 10, this substituent is rare in natural products and contributes to cytotoxicity via electrophilic reactivity .
- NMR Signatures : For example, Compound 10 shows HMBC correlations from H-5 (δH 7.16) and H-6 (δH 7.36) to C-7 (δC 147.0), confirming hydroxylation at C-7 .
Bioactivity and Toxicity
- Cytotoxicity : Compounds 9 and 10 exhibit significant activity against cancer cell lines (e.g., HepG2, A549) with IC₅₀ values < 15 μM, linked to nitro group-mediated DNA adduct formation .
- Nephrotoxicity : All this compound derivatives share the nitro-phenanthrene core, which metabolizes into reactive intermediates capable of binding renal DNA .
- Structural-Activity Relationship (SAR) : Derivatives with hydroxyl groups (e.g., Compound 10) show higher solubility but retain toxicity, whereas methoxylated analogs (e.g., this compound F) may have altered metabolic pathways .
Natural vs. Artificial Origins
Compounds 9 and 10 were confirmed as natural products via LC-MS and isotopic analysis, ruling out artifacts from extraction . This distinguishes them from synthetic AA derivatives, which often lack complex substituents like –OCHO .
Research Findings and Implications
Analytical Techniques
- NMR and HR-ESI-MS : Critical for elucidating substituent positions (e.g., Compound 9’s –OCHO at C-10 confirmed by HMBC) .
- LC-MS: Used to isolate sodium aristolochates from methanol extracts, ensuring purity and natural origin .
Ethnomedicinal Context
Despite toxicity, Aristolochia species remain used in traditional medicine for snakebites, inflammation, and tumors. Sodium salts may enhance solubility of AAs, inadvertently increasing exposure risks .
Biological Activity
Sodium aristolochate, a compound derived from aristolochic acids, has garnered significant attention in pharmacological and toxicological research due to its notable biological activities, particularly its nephrotoxicity and carcinogenic potential. This article provides a comprehensive overview of the biological activity of this compound, including case studies, research findings, and data tables summarizing key results.
Chemical Structure and Properties
This compound is a salt form of aristolochic acid, which is known for its complex structure that contributes to its biological effects. The primary component, aristolochic acid I (AAI), exhibits various pharmacological properties but is also associated with severe toxic effects.
Biological Activity
1. Nephrotoxicity:
this compound has been linked to aristolochic acid nephropathy (AAN), a condition characterized by progressive renal failure. Studies have shown that exposure to this compound leads to significant damage in renal tubular cells, primarily through the formation of DNA adducts that disrupt normal cellular functions.
- Case Study Findings:
- A study involving male rats administered this compound at doses of 200 mg/kg resulted in severe degenerative changes in the testes and kidneys, including nephrosis and tubular degeneration .
- In humans, cases of AAN have been documented where patients consuming herbal products containing aristolochic acids developed chronic kidney disease and urothelial carcinoma .
2. Carcinogenicity:
The carcinogenic potential of this compound is well-documented. It has been classified as a Group 1 carcinogen by various health organizations due to its ability to induce DNA damage leading to mutations.
- Research Findings:
- A study reported a significant correlation between cumulative doses of aristolochic acid and the incidence of urothelial carcinoma among patients with AAN .
- In vitro studies demonstrated that this compound caused chromosomal aberrations and sister chromatid exchanges in mammalian cells, indicating its mutagenic properties .
The mechanisms underlying the biological activities of this compound include:
- DNA Adduct Formation: this compound forms covalent bonds with DNA, resulting in the creation of adducts that can lead to mutations during DNA replication.
- Oxidative Stress: The compound induces oxidative stress within cells, contributing to cellular damage and apoptosis.
- Transport Mechanisms: this compound is actively transported into renal cells via organic anionic transporters (OATs), particularly OAT1, which facilitates its nephrotoxic effects .
Data Summary
The following table summarizes key findings from recent studies on this compound:
Q & A
Basic Research Questions
Q. What are the primary analytical methods for detecting sodium aristolochate in complex herbal matrices?
To identify this compound in plant extracts, researchers typically employ liquid chromatography-tandem mass spectrometry (LC-MS/MS) due to its high sensitivity and specificity. Sample preparation involves methanol extraction, followed by chromatographic separation using reversed-phase C18 columns. Quantification relies on calibration curves validated against certified reference standards. Fluorescence detection may also supplement MS for structural confirmation, especially when differentiating isomers like sodium 7,9-dihydroxy-10-formyloxy aristolochate I .
Q. How can this compound be isolated from plant sources while minimizing degradation?
Isolation requires column chromatography with silica gel or Sephadex LH-20, followed by preparative HPLC. To prevent degradation, avoid prolonged exposure to light and high temperatures during extraction. Stability tests (e.g., NMR monitoring under varying pH and temperature) are critical. Evidence from Aristolochia contorta studies shows that methanol extracts yield higher purity compared to aqueous solvents, but residual alkaloids may require additional purification steps .
Advanced Research Questions
Q. How do researchers reconcile contradictions between in vitro cytotoxicity data and in vivo toxicity profiles of this compound?
Discrepancies often arise from differences in metabolic activation. In vitro models (e.g., HepG2 cells) may lack cytochrome P450 enzymes necessary to metabolize this compound into nephrotoxic intermediates. To address this, advanced studies use primary hepatocyte co-culture systems or supplement assays with S9 liver fractions. Comparative pharmacokinetic modeling (e.g., physiologically based pharmacokinetic, PBPK) can bridge in vitro IC50 values to in vivo exposure thresholds .
Q. What experimental design principles apply when investigating this compound’s nephrotoxicity mechanisms?
Adopt the PICO framework :
- Population : Rodent models with genetic susceptibility to renal fibrosis (e.g., Cyp1a2-knockout mice).
- Intervention : Controlled dosing regimens (oral vs. intraperitoneal).
- Comparison : Cohorts exposed to aristolochic acid I vs. This compound derivatives.
- Outcome : Histopathological scoring of tubular atrophy and DNA adduct quantification via 32P-postlabeling. Include longitudinal biomarkers like serum creatinine and KIM-1 .
Q. What are the methodological challenges in extrapolating in vitro findings to human risk assessment for this compound?
Key challenges include:
- Species-specific metabolism : Human renal proximal tubule cells may process aristolochate metabolites differently than rodent cells.
- Dose-response variability : Nonlinear relationships observed in vitro (e.g., biphasic effects at low/high concentrations) complicate threshold determination.
- Matrix effects : Herbal formulations containing multiple alkaloids may synergize or antagonize toxicity. Use transwell assays to simulate renal tubular reabsorption and integrate multi-omics (transcriptomics, metabolomics) for pathway analysis .
Q. How can NMR and HMBC spectroscopy resolve structural ambiguities in this compound derivatives?
For novel compounds like sodium 9-hydroxy-10-formyloxyaristolochate I, 2D NMR techniques (HMBC, HSQC) map proton-carbon correlations to confirm substituent positions. For example, HMBC correlations between H-5 (δH 7.16) and C-7 (δC 147.0) in compound 10 (A. contorta) validated hydroxylation at C-7. Always compare data with structurally characterized analogues and validate via HR-ESI–MS .
Q. What strategies address contradictory evidence on this compound’s role in renal fibrosis versus carcinogenesis?
Contradictions stem from exposure duration and model selection. Acute studies emphasize tubular injury (e.g., apoptosis via caspase-3 activation), while chronic models show carcinogenic DNA adducts (dA-AL-I). To resolve this, design time-series experiments with endpoints at 7, 30, and 90 days. Use RNA-seq to identify temporal shifts in fibrotic (TGF-β1) vs. oncogenic (p53 mutation) pathways .
Q. How should researchers validate analytical methods for this compound quantification in compliance with regulatory guidelines?
Follow ICH Q2(R1) validation protocols :
- Specificity : Resolve peaks from co-eluting compounds (e.g., aristolactams) using MS/MS transitions.
- Linearity : Test 5–6 concentration levels (r² ≥ 0.995).
- Accuracy : Spike recovery rates (90–110%) in triplicate matrices.
- Precision : Intraday/interday CV < 5%. Document limits of detection (LOD) and quantification (LOQ) using signal-to-noise ratios .
Q. What statistical approaches are appropriate for analyzing biphasic dose-response relationships in this compound studies?
Use nonlinear regression models (e.g., hormesis models) or Bayesian hierarchical models to account for U-shaped curves. For example, low-dose stimulation of inflammatory cytokines (IL-6) vs. high-dose suppression can be modeled with a four-parameter logistic equation. Validate with Akaike information criterion (AIC) comparisons .
Q. How do ethical guidelines influence experimental design in this compound toxicology research?
Adopt ARRIVE 2.0 guidelines for animal studies, emphasizing humane endpoints (e.g., <20% weight loss) and 3Rs (replacement, reduction, refinement). For human cell lines, obtain IRB approval and disclose conflicts of interest, especially if studying traditional herbal formulations. Include negative controls to distinguish compound-specific effects from matrix toxicity .
Properties
CAS No. |
10190-99-5 |
---|---|
Molecular Formula |
C17H11NNaO7 |
Molecular Weight |
364.26 g/mol |
IUPAC Name |
sodium;8-methoxy-6-nitronaphtho[2,1-g][1,3]benzodioxole-5-carboxylate |
InChI |
InChI=1S/C17H11NO7.Na/c1-23-12-4-2-3-8-9(12)5-11(18(21)22)14-10(17(19)20)6-13-16(15(8)14)25-7-24-13;/h2-6H,7H2,1H3,(H,19,20); |
InChI Key |
ABKUMATZXTYAPR-UHFFFAOYSA-N |
SMILES |
COC1=CC=CC2=C3C(=C(C=C21)[N+](=O)[O-])C(=CC4=C3OCO4)C(=O)[O-].[Na+] |
Canonical SMILES |
COC1=CC=CC2=C3C(=C(C=C21)[N+](=O)[O-])C(=CC4=C3OCO4)C(=O)O.[Na] |
Key on ui other cas no. |
10190-99-5 |
Pictograms |
Acute Toxic; Health Hazard |
Synonyms |
8-methoxy-6-nitrophenanthro(3,4-d)-1,3-dioxole-5-carboxylic acid aristolochic acid aristolochic acid A aristolochic acid I aristolochic acid I, sodium salt sodium aristolochate Tardolyt |
Origin of Product |
United States |
Retrosynthesis Analysis
AI-Powered Synthesis Planning: Our tool employs the Template_relevance Pistachio, Template_relevance Bkms_metabolic, Template_relevance Pistachio_ringbreaker, Template_relevance Reaxys, Template_relevance Reaxys_biocatalysis model, leveraging a vast database of chemical reactions to predict feasible synthetic routes.
One-Step Synthesis Focus: Specifically designed for one-step synthesis, it provides concise and direct routes for your target compounds, streamlining the synthesis process.
Accurate Predictions: Utilizing the extensive PISTACHIO, BKMS_METABOLIC, PISTACHIO_RINGBREAKER, REAXYS, REAXYS_BIOCATALYSIS database, our tool offers high-accuracy predictions, reflecting the latest in chemical research and data.
Strategy Settings
Precursor scoring | Relevance Heuristic |
---|---|
Min. plausibility | 0.01 |
Model | Template_relevance |
Template Set | Pistachio/Bkms_metabolic/Pistachio_ringbreaker/Reaxys/Reaxys_biocatalysis |
Top-N result to add to graph | 6 |
Feasible Synthetic Routes
Disclaimer and Information on In-Vitro Research Products
Please be aware that all articles and product information presented on BenchChem are intended solely for informational purposes. The products available for purchase on BenchChem are specifically designed for in-vitro studies, which are conducted outside of living organisms. In-vitro studies, derived from the Latin term "in glass," involve experiments performed in controlled laboratory settings using cells or tissues. It is important to note that these products are not categorized as medicines or drugs, and they have not received approval from the FDA for the prevention, treatment, or cure of any medical condition, ailment, or disease. We must emphasize that any form of bodily introduction of these products into humans or animals is strictly prohibited by law. It is essential to adhere to these guidelines to ensure compliance with legal and ethical standards in research and experimentation.