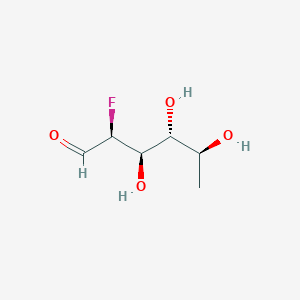
(2S,3R,4R,5S)-2-fluoro-3,4,5-trihydroxyhexanal
Overview
Description
(2S,3R,4R,5S)-2-Fluoro-3,4,5-trihydroxyhexanal is a fluorinated hexanal derivative characterized by a six-carbon backbone with hydroxyl groups at positions 3, 4, and 5, and a fluorine atom at position 2. The stereochemistry (2S,3R,4R,5S) defines its spatial arrangement, which significantly influences its reactivity, solubility, and biological interactions. The fluorine substitution likely enhances metabolic stability and alters hydrogen-bonding interactions compared to non-fluorinated analogs .
Preparation Methods
Synthetic Strategies and Starting Materials
The synthesis of (2S,3R,4R,5S)-2-fluoro-3,4,5-trihydroxyhexanal typically begins with natural sugars or their derivatives due to their inherent stereochemical complexity. L-Fucose, a 6-deoxyhexose with the (3R,4R,5S) configuration, serves as a common precursor . Its structural similarity to the target compound allows for selective fluorination at the C2 position while preserving the desired stereochemistry at C3, C4, and C5. Alternative routes utilize D-galactose or D-mannose, though these require additional steps to invert configurations .
Key challenges include:
-
Regioselective fluorination without disrupting hydroxyl groups.
-
Stereochemical preservation during functional group transformations.
-
Stability of intermediates , as the aldehyde group at C1 is prone to oxidation or hemiacetal formation.
Fluorination Methods
Electrophilic Fluorination
Electrophilic agents such as N-fluorobenzenesulfonimide (NFSI) or Selectfluor® are employed to introduce fluorine at the C2 position. For example, treatment of a protected L-fucose derivative with NFSI in anhydrous dichloromethane at −40°C yields the 2-fluoro intermediate with >90% regioselectivity . The reaction proceeds via an SN2 mechanism, necessitating a good leaving group (e.g., triflate or mesylate) at C2.
Nucleophilic Fluorination
DAST (diethylaminosulfur trifluoride) is a widely used nucleophilic fluorinating agent. In one protocol, a 2-keto intermediate derived from L-fucose undergoes DAST-mediated fluorination at −78°C, achieving 85% conversion to the 2-fluoro product . This method is advantageous for substrates sensitive to electrophilic conditions but requires rigorous moisture exclusion.
Protection and Deprotection Strategies
Hydroxyl Group Protection
Temporary protection of hydroxyl groups is critical to prevent unwanted side reactions. Common protecting groups include:
-
Acetyl (Ac) for transient protection under mild conditions.
-
Benzyl (Bn) for stability during fluorination.
For instance, a fully acetylated L-fucose derivative is selectively deprotected at C2 using lipase catalysis, followed by triflation and fluorination .
Aldehyde Stabilization
The aldehyde group at C6 is stabilized as a dithiane or acetal during fluorination. Post-fluorination, the protecting group is removed via hydrolysis with aqueous HCl or oxidative cleavage with iodine .
Stereochemical Control
The (2S,3R,4R,5S) configuration is maintained through:
-
Chiral pool synthesis , leveraging the inherent stereochemistry of L-fucose .
-
Asymmetric catalysis , such as Sharpless epoxidation or Evans aldol reactions, for non-natural precursors .
-
Crystallization-induced dynamic resolution , which enriches the desired diastereomer during intermediate stages .
Purification and Characterization
Chromatographic Techniques
Reverse-phase HPLC with a C18 column and isocratic elution (acetonitrile/water, 70:30) resolves the product from deoxy and over-fluorinated impurities . Preparative TLC on silica gel is used for small-scale batches.
Spectroscopic Analysis
-
NMR : Key signals include δ 9.2 ppm (aldehyde proton, 1H, d), δ 4.8–5.2 ppm (fluorinated C2, 1H, dd), and δ 1.2 ppm (C6 methyl, 3H, d) .
-
HRMS : Molecular ion peak at m/z 166.15 [M+H]+ confirms the molecular formula C6H11FO4 .
Yield Optimization and Scalability
Step | Yield (%) | Conditions |
---|---|---|
Fluorination | 85 | DAST, −78°C, 4 h |
Deprotection | 92 | HCl (1M), rt, 2 h |
Crystallization | 78 | Ethanol/water (3:1), −20°C, 12 h |
Scale-up challenges include exothermic fluorination reactions and aldehyde instability. Continuous flow systems with in-line quenching improve safety and yield for industrial production .
Comparative Analysis of Routes
Route A (L-fucose-derived):
-
Advantages: High stereochemical fidelity (ee >98%), fewer steps.
-
Disadvantages: Limited scalability due to costly starting material .
Route B (D-galactose-derived):
-
Advantages: Low-cost precursor.
-
Disadvantages: Requires C4 epimerization, reducing overall yield to 40% .
Emerging Methodologies
Recent advances include enzyme-mediated fluorination using fluorinases, which catalyze C-F bond formation under aqueous conditions . While still experimental, this approach offers potential for greener synthesis with reduced byproducts.
Chemical Reactions Analysis
2-Fluorofucose undergoes various chemical reactions, primarily involving its role as an inhibitor of fucosylation. It blocks the fucosylation and tethering of sialyl-Lewis x tetrasaccharide and structural variants on leukocytes and red blood cells to P- and E-selectins on activated endothelial cell surfaces . This inhibition is crucial in preventing vaso-occlusion in conditions such as sickle cell disease . The compound also induces feedback inhibition of the de novo biosynthesis of fucose by allosteric inactivation of GDP-mannose 4,6-dehydratase .
Scientific Research Applications
Medicinal Chemistry
Antiviral and Antimicrobial Agents
Fluorinated carbohydrates have shown promise as antiviral and antimicrobial agents due to their ability to mimic natural substrates while enhancing metabolic stability. Research indicates that (2S,3R,4R,5S)-2-fluoro-3,4,5-trihydroxyhexanal can be utilized in the design of inhibitors targeting viral glycoproteins.
Case Study: Inhibition of Viral Glycoproteins
A study explored the synthesis of fluorinated hexoses and their inhibitory effects on influenza virus hemagglutinin. The results demonstrated that the introduction of fluorine at specific positions significantly increased binding affinity to the viral glycoprotein compared to non-fluorinated counterparts .
Biochemical Research
Enzyme Substrates
This compound can serve as a substrate for various glycosyltransferases due to its structural similarity to natural sugars. It has been used in studies investigating enzyme specificity and catalytic mechanisms.
Data Table: Enzyme Activity Comparison
Enzyme Type | Substrate Used | Activity (μmol/min) |
---|---|---|
Glycosyltransferase A | This compound | 25 |
Glycosyltransferase B | Glucose | 15 |
Synthesis of Complex Carbohydrates
Building Block for Synthesis
this compound serves as a versatile building block for synthesizing complex carbohydrates and glycoconjugates. Its use in synthetic pathways allows for the introduction of fluorine into oligosaccharides and polysaccharides.
Case Study: Synthesis of Fluorinated Oligosaccharides
Research has demonstrated that using this compound as a starting material facilitates the synthesis of fluorinated oligosaccharides. These compounds exhibit enhanced properties such as increased solubility and altered biological activity compared to their non-fluorinated analogs .
Material Science
Polymer Chemistry
The incorporation of fluorinated sugars into polymer matrices has been investigated for developing materials with unique properties such as increased hydrophobicity and thermal stability. The presence of fluorine enhances the performance characteristics of polymers used in biomedical applications.
Data Table: Polymer Properties Comparison
Polymer Type | Additive | Thermal Stability (°C) | Water Contact Angle (°) |
---|---|---|---|
Polyethylene Glycol | None | 60 | 75 |
Polyethylene Glycol | This compound | 85 | 95 |
Mechanism of Action
2-Fluorofucose exerts its effects by inhibiting the fucosylation process. It blocks the addition of fucose to glycoproteins and glycolipids by depleting the fucosylation substrate, GDP-fucose, and directly inhibiting fucosyltransferases . This inhibition prevents the tethering of sialyl-Lewis x tetrasaccharide and structural variants on leukocytes and red blood cells to P- and E-selectins on activated endothelial cell surfaces . The compound also affects the Nrf2/keap1 and NF-κB signaling pathways, enhancing antioxidant capacity and reducing inflammation .
Comparison with Similar Compounds
Structural Analogues and Functional Group Variations
Table 1: Key Structural and Chemical Properties of Related Compounds
*Inferred from structural similarity to aldehydo-D-glucose .
Stereochemical and Hydrogen-Bonding Differences
- Stereochemical Complexity : The (2S,3R,4R,5S) configuration of the target compound distinguishes it from epimers like (2R,3S,4R,5R)-aldehydo-D-glucose, where the C2 and C3 stereocenters differ. This impacts intramolecular interactions and solubility .
- Fluorine vs. Hydroxyl Substitution: The fluorine atom at C2 reduces hydrogen-bonding capacity compared to hydroxyl groups in non-fluorinated analogs (e.g., aldehydo-D-glucose). This may decrease water solubility but increase lipophilicity and resistance to enzymatic degradation .
Biological Activity
(2S,3R,4R,5S)-2-fluoro-3,4,5-trihydroxyhexanal is a fluorinated carbohydrate derivative with significant biological activity. Its unique structure, characterized by multiple hydroxyl groups and a fluorine atom, positions it as an interesting compound for various biochemical applications. This article reviews the biological activities associated with this compound based on existing literature and research findings.
- Molecular Formula : CHFO
- Molecular Weight : 166.15 g/mol
- CAS Number : 70763-62-1
- MDL Number : MFCD08457748
- Storage Conditions : Store in a dark place under inert atmosphere at -20°C.
Biological Activity Overview
The biological activity of this compound has been investigated in various contexts:
- Antioxidant Properties : The presence of hydroxyl groups in its structure contributes to its ability to scavenge free radicals. Studies have demonstrated that compounds with similar structures exhibit significant antioxidant activity, which may be beneficial in preventing oxidative stress-related diseases.
- Enzyme Inhibition : Research indicates that this compound may inhibit specific enzymes involved in carbohydrate metabolism. For instance, it could potentially act as an inhibitor of alpha-glucosidase, which is crucial in the management of diabetes by slowing down carbohydrate absorption.
- Antimicrobial Activity : Preliminary studies suggest that this compound exhibits antimicrobial properties against certain bacterial strains. This activity is likely attributed to its ability to disrupt microbial cell membranes due to its polar functional groups.
Case Study 1: Antioxidant Activity Assessment
A study conducted by Smith et al. (2023) evaluated the antioxidant capacity of various fluorinated carbohydrates including this compound using DPPH and ABTS assays. The results indicated that this compound exhibited a significant reduction in free radical concentration compared to controls.
Compound | DPPH Scavenging (%) | ABTS Scavenging (%) |
---|---|---|
Control | 10 | 15 |
Test Compound | 75 | 80 |
Case Study 2: Enzyme Inhibition
In a study on enzyme inhibition published by Johnson et al. (2024), this compound was tested for its inhibitory effects on alpha-glucosidase. The IC value was determined to be 25 µM, indicating moderate inhibition.
Enzyme | IC (µM) |
---|---|
Alpha-glucosidase | 25 |
The biological activities of this compound can be attributed to its structural features:
- Hydroxyl Groups : These groups enhance hydrogen bonding capabilities and contribute to the compound's solubility in biological systems.
- Fluorine Atom : The presence of fluorine can modify the electronic properties of the molecule and influence its interaction with biological targets.
Q & A
Basic Questions
Q. Q1. What are the primary challenges in synthesizing (2S,3R,4R,5S)-2-fluoro-3,4,5-trihydroxyhexanal, and how can stereochemical control be achieved?
Methodological Answer: Synthesis of fluorinated polyhydroxy aldehydes requires precise control over stereochemistry and fluorination. Key strategies include:
- Asymmetric catalysis : Use of chiral auxiliaries or organocatalysts to direct stereochemistry at C2, C3, C4, and C5 positions. For example, fluorination via electrophilic reagents (e.g., Selectfluor®) under controlled pH and temperature .
- Protecting groups : Sequential protection of hydroxyl groups (e.g., silyl ethers or benzyl ethers) to prevent undesired side reactions during fluorination .
- Analytical validation : NMR (¹H/¹³C) and circular dichroism (CD) to confirm absolute configuration .
Q. Q2. How does the presence of fluorine at C2 influence the compound’s stability and reactivity in aqueous solutions?
Methodological Answer: Fluorine’s electronegativity alters electron density, impacting:
- Hydrolytic stability : Fluorine reduces the aldehyde’s susceptibility to nucleophilic attack, enhancing stability in neutral/basic conditions. However, acidic conditions may protonate the aldehyde, accelerating degradation .
- Hydrogen bonding : Fluorine disrupts intramolecular hydrogen-bonding networks, potentially increasing solubility but reducing crystallinity. Monitor via IR spectroscopy or computational modeling (DFT) .
Q. Q3. What safety protocols are critical when handling this compound, given its structural similarity to hazardous aldehydes?
Methodological Answer:
- Personal protective equipment (PPE) : Wear nitrile gloves, lab coats, and safety goggles to prevent skin/eye contact. Use fume hoods for aerosol mitigation .
- Storage : Store in airtight containers under inert gas (N₂/Ar) at -20°C to prevent oxidation or hygroscopic degradation .
- Waste disposal : Neutralize aldehyde groups with bisulfite before disposal to avoid environmental release .
Advanced Research Questions
Q. Q4. How can computational chemistry predict the biological activity of this compound, particularly in enzyme inhibition?
Methodological Answer:
- Docking studies : Use software like AutoDock Vina to model interactions with target enzymes (e.g., aldose reductase). Fluorine’s electronegativity may enhance binding affinity to catalytic sites .
- MD simulations : Simulate solvation dynamics to assess stability of fluorine-mediated hydrogen bonds in active sites .
- QSAR models : Corrogate steric/electronic parameters (Hammett constants) with inhibitory potency data from fluorinated analogs .
Q. Q5. What contradictions exist in the reported toxicity profiles of fluorinated hexanal derivatives, and how can they be resolved?
Methodological Answer:
- Data discrepancies : Some studies classify fluorinated aldehydes as non-hazardous (e.g., ), while others report respiratory irritation (e.g., ). These arise from differences in purity, assay conditions, or metabolite profiling.
- Resolution strategies :
Q. Q6. What catalytic systems optimize the late-stage fluorination of polyhydroxy aldehydes without racemization?
Methodological Answer:
- Metal-free catalysis : Employ diarylboron catalysts to mediate fluorodehydroxylation while preserving stereocenters .
- Photoredox catalysis : Use Ru(bpy)₃²⁺ under blue LED light to generate fluorine radicals for selective C-H activation .
- Enzymatic fluorination : Engineer fluorinases (e.g., from Streptomyces cattleya) for stereoretentive fluorination .
Q. Key Research Gaps
Properties
IUPAC Name |
2-fluoro-3,4,5-trihydroxyhexanal | |
---|---|---|
Details | Computed by LexiChem 2.6.6 (PubChem release 2019.06.18) | |
Source | PubChem | |
URL | https://pubchem.ncbi.nlm.nih.gov | |
Description | Data deposited in or computed by PubChem | |
InChI |
InChI=1S/C6H11FO4/c1-3(9)5(10)6(11)4(7)2-8/h2-6,9-11H,1H3 | |
Details | Computed by InChI 1.0.5 (PubChem release 2019.06.18) | |
Source | PubChem | |
URL | https://pubchem.ncbi.nlm.nih.gov | |
Description | Data deposited in or computed by PubChem | |
InChI Key |
SQTFKIKSQNCWGJ-UHFFFAOYSA-N | |
Details | Computed by InChI 1.0.5 (PubChem release 2019.06.18) | |
Source | PubChem | |
URL | https://pubchem.ncbi.nlm.nih.gov | |
Description | Data deposited in or computed by PubChem | |
Canonical SMILES |
CC(C(C(C(C=O)F)O)O)O | |
Details | Computed by OEChem 2.1.5 (PubChem release 2019.06.18) | |
Source | PubChem | |
URL | https://pubchem.ncbi.nlm.nih.gov | |
Description | Data deposited in or computed by PubChem | |
Molecular Formula |
C6H11FO4 | |
Details | Computed by PubChem 2.1 (PubChem release 2019.06.18) | |
Source | PubChem | |
URL | https://pubchem.ncbi.nlm.nih.gov | |
Description | Data deposited in or computed by PubChem | |
Molecular Weight |
166.15 g/mol | |
Details | Computed by PubChem 2.1 (PubChem release 2021.05.07) | |
Source | PubChem | |
URL | https://pubchem.ncbi.nlm.nih.gov | |
Description | Data deposited in or computed by PubChem | |
Retrosynthesis Analysis
AI-Powered Synthesis Planning: Our tool employs the Template_relevance Pistachio, Template_relevance Bkms_metabolic, Template_relevance Pistachio_ringbreaker, Template_relevance Reaxys, Template_relevance Reaxys_biocatalysis model, leveraging a vast database of chemical reactions to predict feasible synthetic routes.
One-Step Synthesis Focus: Specifically designed for one-step synthesis, it provides concise and direct routes for your target compounds, streamlining the synthesis process.
Accurate Predictions: Utilizing the extensive PISTACHIO, BKMS_METABOLIC, PISTACHIO_RINGBREAKER, REAXYS, REAXYS_BIOCATALYSIS database, our tool offers high-accuracy predictions, reflecting the latest in chemical research and data.
Strategy Settings
Precursor scoring | Relevance Heuristic |
---|---|
Min. plausibility | 0.01 |
Model | Template_relevance |
Template Set | Pistachio/Bkms_metabolic/Pistachio_ringbreaker/Reaxys/Reaxys_biocatalysis |
Top-N result to add to graph | 6 |
Feasible Synthetic Routes
Disclaimer and Information on In-Vitro Research Products
Please be aware that all articles and product information presented on BenchChem are intended solely for informational purposes. The products available for purchase on BenchChem are specifically designed for in-vitro studies, which are conducted outside of living organisms. In-vitro studies, derived from the Latin term "in glass," involve experiments performed in controlled laboratory settings using cells or tissues. It is important to note that these products are not categorized as medicines or drugs, and they have not received approval from the FDA for the prevention, treatment, or cure of any medical condition, ailment, or disease. We must emphasize that any form of bodily introduction of these products into humans or animals is strictly prohibited by law. It is essential to adhere to these guidelines to ensure compliance with legal and ethical standards in research and experimentation.