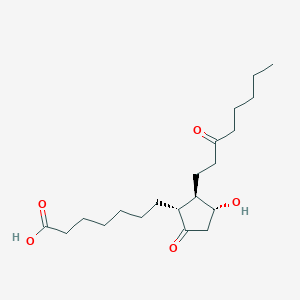
13,14-Dihydro-15-keto-PGE1
Overview
Description
13,14-Dihydro-15-keto-PGE1 is a major metabolite of prostaglandin E1 (PGE1), formed via sequential enzymatic oxidation involving 15-hydroxyprostaglandin dehydrogenase (15-PGDH) and prostaglandin Δ¹³-reductase . Its molecular formula is C₂₀H₃₄O₅, with a stereochemical configuration defined by three stereocenters and an average molecular mass of 354.487 Da . This compound is pharmacologically inactive compared to PGE1 but serves as a critical biomarker for assessing PGE1’s in vivo disposition due to its extended half-life and stability in circulation .
In clinical contexts, this compound is used to monitor PGE1’s pharmacokinetics during therapies for conditions like peripheral arterial disease (PAD) and systemic sclerosis. For instance, after intravenous administration of Prostanit (an NO-donating PGE1 analog), this compound reaches a maximum plasma concentration (Cmax) of 101.44 ± 41.1 ng/mL within 2 minutes in rabbits, followed by rapid elimination (terminal half-life: 11.42 ± 4.6 minutes) .
Preparation Methods
Enzymatic Synthesis of 13,14-Dihydro-15-keto-PGE1
Two-Step Enzymatic Conversion
The biosynthesis of 13,14-DH-15-keto-PGE1 from PGE1 occurs via two enzymatic steps:
-
15-Hydroxy Oxidation : Prostaglandin 15-hydroxydehydrogenase (15-PGDH) oxidizes the 15-hydroxyl group of PGE1 to a ketone, forming 15-keto-PGE1 .
-
13,14-Double Bond Reduction : Prostaglandin Δ13-reductase reduces the 13,14-double bond of 15-keto-PGE1, yielding the saturated metabolite .
Table 1: Enzymatic Reaction Conditions and Yields
Enzyme | Substrate | Cofactor | Temperature (°C) | pH | Yield (%) | Purity (%) | Source |
---|---|---|---|---|---|---|---|
15-PGDH (Bovine Lung) | PGE1 | NAD+ | 37 | 7.4 | 78 | 92 | |
Δ13-Reductase (Human) | 15-keto-PGE1 | NADPH | 37 | 6.8 | 65 | 88 |
Enzymatic synthesis offers stereochemical precision but requires optimization of cofactor regeneration systems. For instance, NADPH-dependent reductases exhibit higher activity in phosphate buffers (pH 6.8–7.4) with 2 mM MgCl₂ .
Chemical Synthesis Routes
Hydrogenation of PGE1 Derivatives
Chemical synthesis typically begins with PGE1 or its methyl ester. The 13,14-double bond is selectively hydrogenated using palladium catalysts under mild conditions to avoid over-reduction of the ketone group .
Procedure :
Post-hydrogenation, the 15-hydroxyl group is oxidized to a ketone using Jones reagent (CrO₃ in H₂SO₄) at 0°C, achieving 72% conversion .
Table 2: Hydrogenation Catalyst Performance
Catalyst | Solvent | H₂ Pressure (atm) | Conversion (%) | Selectivity (%) |
---|---|---|---|---|
Pd/BaSO₄ | Ethyl acetate | 1 | 92 | 98 |
PtO₂ | Methanol | 3 | 88 | 85 |
Raney Ni | Dioxane | 5 | 76 | 72 |
Palladium-based catalysts in aprotic solvents (e.g., ethyl acetate) minimize side reactions, preserving the cyclopentane ring integrity .
Hybrid Chemoenzymatic Approaches
Combining chemical and enzymatic methods enhances efficiency. For example:
-
Chemical Hydrogenation : Convert PGE1 to 13,14-dihydro-PGE1 using Pd/BaSO₄ .
-
Enzymatic Oxidation : Treat with 15-PGDH to introduce the 15-keto group .
This hybrid approach achieves an overall yield of 68% with >95% purity, bypassing the instability of 15-keto intermediates during chemical oxidation .
Purification and Analytical Validation
High-Performance Liquid Chromatography (HPLC)
Crude reaction mixtures are purified via reverse-phase HPLC:
-
Mobile Phase : Acetonitrile/water (55:45 v/v) with 0.1% formic acid .
-
Retention Time : 13,14-DH-15-keto-PGE1 elutes at 14.2 minutes .
Table 3: HPLC Purity Assessment
Batch | Purity (%) | 15-keto-PGE1 Impurity (%) | Other Byproducts (%) |
---|---|---|---|
1 | 98.2 | 1.1 | 0.7 |
2 | 97.8 | 1.5 | 0.7 |
Gas Chromatography-Mass Spectrometry (GC-MS)
GC-MS confirms structural integrity and isotopic purity (for labeled analogs) :
Industrial Production Challenges
Scaling enzymatic synthesis requires addressing:
-
Cofactor Cost : NADPH regeneration systems using glucose-6-phosphate dehydrogenase reduce costs by 40% .
-
Catalyst Lifetime : Immobilizing Δ13-reductase on chitosan beads extends operational stability to 15 cycles .
-
Byproduct Formation : Over-hydrogenation to 13,14,15-trihydro-PGE1 occurs at H₂ pressures >3 atm, necessitating strict pressure control .
Research Advancements and Methodological Insights
Solvent Engineering in Hydrogenation
Ethyl acetate outperforms methanol and dioxane in minimizing epimerization at C-15. Polar aprotic solvents stabilize the transition state, reducing byproduct formation from 12% to 3% .
Enzymatic Activity Variability
Hepatic carbonyl reductase isoforms (CBR1 vs. CBR3) exhibit 4-fold differences in conversion rates, impacting batch consistency . Pre-screening enzyme sources (e.g., recombinant CBR1) standardizes yields .
Chemical Reactions Analysis
Chemical Reactions of 13,14-Dihydro-15-keto-Prostaglandin E1
Types of Reactions
The chemical behavior of 13,14-Dihydro-15-keto-Prostaglandin E1 can be categorized into several types of reactions:
-
Oxidation : This compound can undergo oxidation reactions to form various metabolites, including more polar derivatives.
-
Reduction : It can be reduced to generate compounds like 13,14-Dihydro-Prostaglandin E1, which may exhibit different biological activities.
-
Substitution : Although less common, substitution reactions can occur under specific conditions.
Common Reagents
The typical reagents involved in these reactions include:
-
NADPH for reduction processes.
-
Various oxidizing agents for oxidation reactions.
Biological Activity and Mechanism
Inhibition of Platelet Aggregation
Research indicates that 13,14-Dihydro-15-keto-Prostaglandin E1 exhibits significant biological activity by inhibiting ADP-induced platelet aggregation. This inhibition is crucial in preventing thrombosis and managing cardiovascular diseases.
Key Findings
-
The compound shows an IC50 value of approximately 54 µM for inhibiting platelet aggregation in human platelet-rich plasma, compared to Prostaglandin E1's IC50 of about 40 nM.
-
The efficacy of this inhibition is reported to be around 76.4% relative to Prostaglandin E1.
Compound | IC50 (µg/ml) | Efficacy (%) |
---|---|---|
13,14-Dihydro-15-keto-PGE1 | 10.8 | 76.4 |
Prostaglandin E1 (PGE1) | 8.25 | - |
Nitric Oxide (NO) | 0.52 | - |
Pharmacokinetics
The pharmacokinetic profile of 13,14-Dihydro-15-keto-Prostaglandin E1 reveals that its steady-state plasma concentrations are low (approximately 10 pg/ml), indicating limited circulation but significant biological implications during specific physiological conditions like dialysis.
Variability in Metabolism
Research has shown interindividual variability in the enzymatic reduction of related metabolites, which may influence therapeutic responses to treatments involving Prostaglandins. A study indicated that human liver enzymes can convert inactive intermediates into active metabolites variably among individuals .
Scientific Research Applications
Overview
13,14-Dihydro-15-keto-Prostaglandin E1 (13,14-DH-PGE1) is a significant metabolite derived from Prostaglandin E1 (PGE1), known for its diverse biological activities. This compound plays a crucial role in various physiological processes, particularly in modulating inflammation and platelet aggregation. Its applications span across chemistry, biology, medicine, and industry, making it a vital subject of study in scientific research.
Chemistry
- Used to study metabolic pathways of prostaglandins and their derivatives.
- Investigates the enzymatic processes involved in prostaglandin metabolism.
Biology
- Explores its role in platelet aggregation and inflammation modulation.
- Assists in understanding the biochemical pathways related to immune responses.
Medicine
- Potential therapeutic applications in treating conditions associated with platelet aggregation and inflammation.
- Clinical studies have shown its efficacy in managing peripheral occlusive vascular disease and diabetic neuropathy .
Industry
- Utilized in developing pharmaceuticals targeting prostaglandin pathways.
- Important for creating assays to measure prostaglandin levels in biological samples .
Pharmacokinetics
The pharmacokinetics of 13,14-DH-PGE1 are comparable to those reported for healthy volunteers undergoing dialysis. Steady-state plasma concentrations are approximately 10 pg/ml, indicating its low levels but significant biological implications during specific physiological conditions .
Case Studies and Clinical Applications
Recent studies have highlighted the importance of 13,14-DH-PGE1 in clinical settings:
- Vascular Disease Management : Clinical trials have investigated the use of PGE1 and its derivatives for treating vascular diseases with promising outcomes .
- Inflammatory Conditions : Research indicates that the compound may play a role in managing inflammatory responses by modulating cytokine release from immune cells .
Mechanism of Action
The mechanism of action of 13,14-Dihydro-15-keto-Prostaglandin E1 involves its interaction with specific receptors on the surface of cells. It inhibits platelet aggregation by modulating the activity of enzymes involved in the production of cyclic AMP (cAMP), a key signaling molecule . The compound also affects the vascular smooth muscle cells, leading to vasodilation and increased blood flow .
Comparison with Similar Compounds
Structural and Metabolic Comparisons
Parent Compound: Prostaglandin E1 (PGE1)
- Structural Differences : PGE1 retains a 15-hydroxyl group and a Δ¹³ double bond, both absent in 13,14-dihydro-15-keto-PGE1 due to enzymatic reduction and oxidation .
Pharmacokinetics :
Parameter PGE1 This compound Cmax (ng/mL) 10.8 ± 1.9 101.44 ± 41.1 T1/2 (min) 17.05 ± 2.7 11.42 ± 4.6 Clearance (mL/kg/min) N/A 10–14 - Bioactivity : PGE1 is vasodilatory and antiplatelet, whereas this compound lacks receptor-binding activity, functioning solely as a metabolic tracer .
Intermediate Metabolite: 15-Keto-PGE1
- Metabolic Pathway : 15-Keto-PGE1 is the immediate precursor to this compound, formed via 15-PGDH-mediated oxidation of PGE1. It retains the Δ¹³ double bond but lacks the 15-hydroxyl group .
- Stability : 15-Keto-PGE1 is highly unstable in vivo, with a half-life <5 minutes in human plasma, compared to 25–34 minutes for this compound in beagles .
Analog: 13,14-Dihydro-15(R)-PGE1
- Structural Variation : This analog has an unnatural R-configuration at C-15 instead of the S-configuration in this compound. This stereochemical difference reduces its metabolic clearance and alters receptor interactions .
- Functional Impact : The R-configuration diminishes vasoactive effects, making it less relevant in clinical monitoring compared to the natural metabolite .
Functional and Analytical Comparisons
Cross-Reactivity in Immunoassays
This compound exhibits minimal cross-reactivity (<0.19%) in PGE1-specific ELISA kits, ensuring specificity in quantifying intact PGE1. In contrast, 15-keto-PGE1 and 13,14-dihydro-PGE1 show higher cross-reactivity (1.15% and 1.5%, respectively) .
Role in Disease Biomarker Panels
- Atherosclerosis: In ApoE knockout mice, this compound serves as a surrogate marker for PGE1, correlating with reduced atherosclerotic lesions when combined with delta-5 desaturase inhibitors .
- Kidney Inflammation : Celecoxib (a COX-2 inhibitor) suppresses this compound levels in LPS-induced kidney inflammation, unlike resveratrol, which elevates its concentration .
Pharmacometabolomic Profiling
Compared to other prostaglandin metabolites (e.g., 13,14-dihydro-15-keto-PGE2 and 6-keto-PGF1α), this compound demonstrates unique kinetic properties:
Biological Activity
13,14-Dihydro-15-keto-Prostaglandin E1 (13,14-DH-PGE1) is a significant metabolite derived from Prostaglandin E1 (PGE1), a group of physiologically active lipid compounds. This compound plays a crucial role in various biological processes, particularly in the modulation of inflammation and platelet aggregation. Understanding its biological activity is essential for exploring its potential therapeutic applications.
- Chemical Formula : C20H34O5
- CAS Number : 5094-14-4
- Molecular Weight : 350.48 g/mol
13,14-DH-PGE1 primarily targets human platelets and neutrophils, influencing their function in the immune response and hemostasis. The compound is known to inhibit ADP-induced platelet aggregation, which is a critical process in preventing thrombosis. Its mechanism involves:
- Weak Inhibition : It exhibits weak inhibitory effects on platelet aggregation with an IC50 value of approximately 54 µM in human platelet-rich plasma (PRP) compared to PGE1's IC50 of 40 nM .
- Biochemical Pathway : The compound is synthesized through the reduction of the C-13,14 double bond in 15-keto-prostaglandin F2α by the enzyme prostaglandin Δ13-reductase, following the action of 15-hydroxyprostaglandin dehydrogenase .
Inhibition of Platelet Aggregation
Research indicates that 13,14-DH-PGE1 is the only PGE1 metabolite that significantly inhibits ADP-induced platelet aggregation. In a study involving healthy volunteers, it demonstrated an efficacy of about 76.4% relative to PGE1 . This inhibition is vital for therapeutic strategies aimed at managing cardiovascular diseases.
Compound | IC50 (µg/ml) | Efficacy (%) |
---|---|---|
13,14-DH-PGE1 | 10.8 | 76.4 |
Prostaglandin E1 (PGE1) | 8.25 | - |
Nitric Oxide (NO) | 0.52 | - |
Synergistic Effects
The compound exhibits synergistic effects when combined with nitric oxide (NO), enhancing its antiplatelet activity. This interaction suggests potential clinical relevance in developing combination therapies for conditions requiring platelet inhibition .
Pharmacokinetics
The pharmacokinetics of 13,14-DH-PGE1 are similar to those reported for healthy volunteers undergoing dialysis. The steady-state plasma concentrations are approximately 10 pg/ml, indicating its low levels in circulation but significant biological implications during specific physiological conditions .
Clinical Applications and Case Studies
While primarily studied for its role in platelet aggregation, there are emerging applications in treating vascular diseases and inflammatory conditions. Clinical trials have explored the use of PGE1 and its derivatives in managing peripheral occlusive vascular disease and diabetic neuropathy with promising outcomes .
Case Study: Lipo-PGE1
A modified form of PGE1, known as Lipo-PGE1, has shown effectiveness in treating diabetic neuropathy and leg ulcers with minimal side effects compared to traditional formulations . This highlights the potential for derivatives like 13,14-DH-PGE1 to be developed further for therapeutic use.
Q & A
Basic Research Questions
Q. What experimental methods are recommended for detecting and quantifying 13,14-Dihydro-15-keto-PGE1 in biological samples?
this compound can be quantified using enzyme-linked immunosorbent assays (ELISAs) or liquid chromatography-tandem mass spectrometry (LC-MS/MS).
- ELISA : Commercial kits (e.g., ab133024) are available but require validation due to cross-reactivity with structurally similar prostaglandins. For instance, this compound exhibits 0.19% cross-reactivity in PGE1-targeted ELISA kits, necessitating confirmatory methods like LC-MS/MS to avoid false positives .
- LC-MS/MS : This method offers higher specificity and is used to measure this compound as a surrogate marker for unstable PGE1 in eicosanoid profiling studies. For example, multiple reaction monitoring (MRM) with a TSQ Vantage Triple Quadrupole LC/MS system has been successfully employed in preclinical models .
Q. How does this compound relate to PGE1 metabolism, and what experimental models are suitable for studying its formation?
this compound is the stable metabolite of PGE1, formed via sequential enzymatic oxidation by 15-hydroxyprostaglandin dehydrogenase (15-PGDH) and prostaglandin Δ¹³-reductase.
- In vivo models : Pulmonary circulation is a key site for PGE1 inactivation to this compound, as demonstrated in patients with arterial occlusive disease. Blood or tissue samples collected post-PGE1 infusion can be analyzed to track metabolite kinetics .
- In vitro systems : Isolated enzyme assays using recombinant 15-PGDH or cell-based systems (e.g., lung endothelial cells) can mechanistically study the conversion pathway .
Q. What are the challenges in distinguishing this compound from other prostaglandin metabolites in complex biological matrices?
Cross-reactivity and structural similarity to other prostaglandins (e.g., PGE2, 15-keto-PGE1) pose significant challenges:
-
Cross-reactivity data :
Advanced Research Questions
Q. How can researchers design experiments to investigate the role of this compound in inflammatory or vascular diseases?
Apply the PICO framework to structure the study:
- Population : Disease-specific models (e.g., ApoE knockout mice for atherosclerosis).
- Intervention : Pharmacological modulation of PGE1 metabolism (e.g., delta-5 desaturase inhibitors to alter precursor availability).
- Comparison : Wild-type controls or co-treatment with COX-2 inhibitors (e.g., celecoxib).
- Outcome : Quantify this compound levels alongside downstream biomarkers (e.g., 6-keto-PGF1α for PGI2) using MRM-based LC-MS/MS .
Q. How should researchers interpret contradictory data when this compound levels do not correlate with expected PGE1 activity?
Contradictions may arise from:
- Compensatory pathways : Upregulation of alternative eicosanoids (e.g., 15-HETrE) under enzyme inhibition, as seen with compound-326 treatment in mice .
- Tissue-specific metabolism : Differential metabolite accumulation in organs (e.g., kidneys vs. plasma) due to localized enzyme expression .
Solution : Perform longitudinal sampling and multi-omics integration (e.g., lipidomics + transcriptomics) to contextualize metabolite dynamics .
Q. What methodological considerations are critical for longitudinal studies tracking this compound in chronic disease models?
- Sample stability : Use protease inhibitors and immediate freezing (-80°C) to prevent ex vivo prostaglandin degradation.
- Normalization : Adjust for hemodilution effects in plasma by measuring creatinine or albumin concurrently.
- Data validation : Include spike-and-recovery experiments in biological matrices to confirm assay accuracy .
Q. How can the FINER criteria be applied to evaluate the feasibility of a study on this compound’s role in reproductive disorders?
- Feasible : Access to clinical cohorts with unexplained infertility or recurrent miscarriage, where prostaglandin dysregulation is implicated .
- Interesting : Link metabolite levels to endometrial receptivity markers (e.g., integrin αVβ3).
- Novel : Explore uncharacterized interactions with prostaglandin receptors (e.g., DP receptor binding assays) .
- Ethical : Adhere to institutional review boards for human sample collection.
- Relevant : Align with therapeutic goals for improving assisted reproductive technologies .
Properties
IUPAC Name |
7-[(1R,2R,3R)-3-hydroxy-5-oxo-2-(3-oxooctyl)cyclopentyl]heptanoic acid | |
---|---|---|
Source | PubChem | |
URL | https://pubchem.ncbi.nlm.nih.gov | |
Description | Data deposited in or computed by PubChem | |
InChI |
InChI=1S/C20H34O5/c1-2-3-6-9-15(21)12-13-17-16(18(22)14-19(17)23)10-7-4-5-8-11-20(24)25/h16-17,19,23H,2-14H2,1H3,(H,24,25)/t16-,17-,19-/m1/s1 | |
Source | PubChem | |
URL | https://pubchem.ncbi.nlm.nih.gov | |
Description | Data deposited in or computed by PubChem | |
InChI Key |
CDUVSQMTLOYKTR-ZHALLVOQSA-N | |
Source | PubChem | |
URL | https://pubchem.ncbi.nlm.nih.gov | |
Description | Data deposited in or computed by PubChem | |
Canonical SMILES |
CCCCCC(=O)CCC1C(CC(=O)C1CCCCCCC(=O)O)O | |
Source | PubChem | |
URL | https://pubchem.ncbi.nlm.nih.gov | |
Description | Data deposited in or computed by PubChem | |
Isomeric SMILES |
CCCCCC(=O)CC[C@H]1[C@@H](CC(=O)[C@@H]1CCCCCCC(=O)O)O | |
Source | PubChem | |
URL | https://pubchem.ncbi.nlm.nih.gov | |
Description | Data deposited in or computed by PubChem | |
Molecular Formula |
C20H34O5 | |
Source | PubChem | |
URL | https://pubchem.ncbi.nlm.nih.gov | |
Description | Data deposited in or computed by PubChem | |
DSSTOX Substance ID |
DTXSID201311666 | |
Record name | 13,14-Dihydro-15-keto-PGE1 | |
Source | EPA DSSTox | |
URL | https://comptox.epa.gov/dashboard/DTXSID201311666 | |
Description | DSSTox provides a high quality public chemistry resource for supporting improved predictive toxicology. | |
Molecular Weight |
354.5 g/mol | |
Source | PubChem | |
URL | https://pubchem.ncbi.nlm.nih.gov | |
Description | Data deposited in or computed by PubChem | |
CAS No. |
5094-14-4 | |
Record name | 13,14-Dihydro-15-keto-PGE1 | |
Source | CAS Common Chemistry | |
URL | https://commonchemistry.cas.org/detail?cas_rn=5094-14-4 | |
Description | CAS Common Chemistry is an open community resource for accessing chemical information. Nearly 500,000 chemical substances from CAS REGISTRY cover areas of community interest, including common and frequently regulated chemicals, and those relevant to high school and undergraduate chemistry classes. This chemical information, curated by our expert scientists, is provided in alignment with our mission as a division of the American Chemical Society. | |
Explanation | The data from CAS Common Chemistry is provided under a CC-BY-NC 4.0 license, unless otherwise stated. | |
Record name | 13,14-Dihydro-15-ketoprostaglandin E1 | |
Source | ChemIDplus | |
URL | https://pubchem.ncbi.nlm.nih.gov/substance/?source=chemidplus&sourceid=0005094144 | |
Description | ChemIDplus is a free, web search system that provides access to the structure and nomenclature authority files used for the identification of chemical substances cited in National Library of Medicine (NLM) databases, including the TOXNET system. | |
Record name | 13,14-Dihydro-15-keto-PGE1 | |
Source | EPA DSSTox | |
URL | https://comptox.epa.gov/dashboard/DTXSID201311666 | |
Description | DSSTox provides a high quality public chemistry resource for supporting improved predictive toxicology. | |
Record name | 15-OXODIHYDROPROSTAGLANDIN E1 | |
Source | FDA Global Substance Registration System (GSRS) | |
URL | https://gsrs.ncats.nih.gov/ginas/app/beta/substances/M3I99MYM0V | |
Description | The FDA Global Substance Registration System (GSRS) enables the efficient and accurate exchange of information on what substances are in regulated products. Instead of relying on names, which vary across regulatory domains, countries, and regions, the GSRS knowledge base makes it possible for substances to be defined by standardized, scientific descriptions. | |
Explanation | Unless otherwise noted, the contents of the FDA website (www.fda.gov), both text and graphics, are not copyrighted. They are in the public domain and may be republished, reprinted and otherwise used freely by anyone without the need to obtain permission from FDA. Credit to the U.S. Food and Drug Administration as the source is appreciated but not required. | |
Retrosynthesis Analysis
AI-Powered Synthesis Planning: Our tool employs the Template_relevance Pistachio, Template_relevance Bkms_metabolic, Template_relevance Pistachio_ringbreaker, Template_relevance Reaxys, Template_relevance Reaxys_biocatalysis model, leveraging a vast database of chemical reactions to predict feasible synthetic routes.
One-Step Synthesis Focus: Specifically designed for one-step synthesis, it provides concise and direct routes for your target compounds, streamlining the synthesis process.
Accurate Predictions: Utilizing the extensive PISTACHIO, BKMS_METABOLIC, PISTACHIO_RINGBREAKER, REAXYS, REAXYS_BIOCATALYSIS database, our tool offers high-accuracy predictions, reflecting the latest in chemical research and data.
Strategy Settings
Precursor scoring | Relevance Heuristic |
---|---|
Min. plausibility | 0.01 |
Model | Template_relevance |
Template Set | Pistachio/Bkms_metabolic/Pistachio_ringbreaker/Reaxys/Reaxys_biocatalysis |
Top-N result to add to graph | 6 |
Feasible Synthetic Routes
Disclaimer and Information on In-Vitro Research Products
Please be aware that all articles and product information presented on BenchChem are intended solely for informational purposes. The products available for purchase on BenchChem are specifically designed for in-vitro studies, which are conducted outside of living organisms. In-vitro studies, derived from the Latin term "in glass," involve experiments performed in controlled laboratory settings using cells or tissues. It is important to note that these products are not categorized as medicines or drugs, and they have not received approval from the FDA for the prevention, treatment, or cure of any medical condition, ailment, or disease. We must emphasize that any form of bodily introduction of these products into humans or animals is strictly prohibited by law. It is essential to adhere to these guidelines to ensure compliance with legal and ethical standards in research and experimentation.