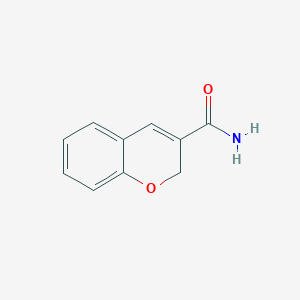
2H-chromene-3-carboxamide
Overview
Description
2H-Chromene-3-carboxamide is a bicyclic heterocyclic compound characterized by a benzopyran core fused with a carboxamide group at position 3. Its synthesis typically involves condensation reactions of salicylaldehydes with cyanoacetamide derivatives in the presence of catalysts like piperidine, yielding 2-imino-2H-chromene-3-carboxamide intermediates. These intermediates undergo rearrangements with N-nucleophiles (e.g., benzohydrazide) in acidic media to form diverse derivatives, including oxadiazolylchromenes and tetrahydrobenzo[b]thiophene hybrids . The compound’s structural versatility enables applications in medicinal chemistry, particularly as tyrosine kinase inhibitors and intermediates for bioactive heterocycles .
Preparation Methods
Knoevenagel Condensation for Core Chromene Formation
Base-Catalyzed Cyclization in Aqueous Media
The Knoevenagel condensation between salicylaldehyde derivatives 4 and N-substituted cyanoacetamides 3 offers a one-step route to 2-imino-2H-chromene-3-carboxamides 5 (Fig. 1). Conducted in aqueous sodium carbonate or hydrogen carbonate solutions at room temperature, this method achieves >90% atom economy and eliminates organic solvents . For instance, reacting 5-chlorosalicylaldehyde with N-ethyl cyanoacetamide produces the corresponding carboxamide in 92% yield after 6 hours. The aqueous conditions prevent racemization of chiral centers, making this approach suitable for stereosensitive applications.
Post-synthetic modification of the 2-imino group via HCl treatment yields 2-oxo-2H-chromene-3-carboxamides 6 , expanding the derivative library. This two-stage process demonstrates exceptional functional group tolerance, accommodating electron-withdrawing (Cl, Br) and donating (OCH₃) substituents on the aromatic ring .
Baylis-Hillman Adduct-Based Multistep Synthesis
Tert-Butyl Acrylate Coupling and Cyclization
A robust multigram synthesis begins with Baylis-Hillman adduct formation between salicylaldehydes 1a-c and tert-butyl acrylate using diazabicyclo[2.2.2]octane (DABCO) catalyst (Scheme 1). Reaction in chloroform at ambient temperature for 3 weeks yields tert-butyl-3-(2-hydroxyphenyl)-2-methylenepropanoates 2a-c with 28–47% isolated yields . Subsequent base-mediated cyclization with potassium hydroxide (4 eq.) under reflux for 46 hours generates 2H-chromene-3-carboxylic acids 3a-c in 37–62% yield.
DCC-Mediated Amide Coupling
Carboxamide formation employs dicyclohexylcarbodiimide (DCC) to activate the carboxylic acid for reaction with primary amines (Table 1). For example, treating 2H-chromene-3-carboxylic acid 3a (1.14 mmol) with benzylamine (1.14 mmol) in dichloromethane at 0°C produces N-benzyl-2H-chromene-3-carboxamide 4a in 56.5% yield after 16 hours . Halogenated derivatives (4b-c ) show reduced yields (30–33%) due to increased steric hindrance from chloro/bromo substituents.
Table 1. Yields and Characterization of Selected 2H-Chromene-3-Carboxamides
Compound | R₁ | R₂ | Yield (%) | M.p. (°C) | IR ν(C=O) (cm⁻¹) |
---|---|---|---|---|---|
4a | H | CH₂Ph | 56.5 | 153–156 | 1631 |
4b | Cl | CH₂Ph | 32.7 | 159–162 | 1627 |
4c | Br | CH₂Ph | 30.3 | 164–166 | 1624 |
4d | H | H | 37.6 | 137–141 | 1660 |
Ultrasound-Assisted Green Synthesis
Solvent-Free Aminolysis Under Irradiation
A breakthrough in scalability involves ultrasound-mediated (675 W, 5 hours) coupling of 2-oxo-2H-chromene-3-carbonyl chloride with ethanolamine, achieving 83% yield for N-(2-hydroxyethyl)-2-oxo-2H-chromene-3-carboxamide at 0.38 mol scale . Compared to traditional heating, ultrasonication reduces reaction time from 24 hours to 5 hours while improving purity by minimizing side reactions. This method’s energy efficiency (45°C vs. 80°C under reflux) makes it ideal for thermally labile substrates.
Direct Aminolysis of Chromene Esters
Ethyl 2-Oxo-2H-chromene-3-carboxylate Reactivity
Ethyl 2-oxo-2H-chromene-3-carboxylate 7 serves as a versatile precursor for carboxamides. Reacting 7 (1 mmol) with 4-methoxyphenethylamine 6 (1.2 mmol) in ethanol under reflux for 6 hours affords N-(4-methoxyphenethyl)-2-oxo-2H-chromene-3-carboxamide 8 in 84% yield . Toluene proves superior to ethanol for ester synthesis, reducing reaction time from 12 hours to 1 hour at reflux.
Table 2. Comparative Analysis of Preparation Methods
Mechanistic Insights and Optimization Strategies
Knoevenagel Reaction Pathway
The reaction proceeds via deprotonation of cyanoacetamide’s α-hydrogen by carbonate, forming a resonance-stabilized enolate. Nucleophilic attack on the salicylaldehyde carbonyl generates an intermediate aldol adduct, which undergoes intramolecular cyclization and dehydration to yield the chromene core .
DCC Coupling Mechanism
DCC activates the carboxylic acid through O-acylisourea intermediate formation. Amine nucleophilic attack displaces dicyclohexylurea, yielding the carboxamide. Excess DCC (1.1 eq.) compensates for side reactions with hydroxyl groups in substituted chromenes .
Chemical Reactions Analysis
Reaction with Phosphorus Compounds
The reactivity of 2H-chromene-3-carboxamide also extends to phosphorus-containing compounds. For instance, treatment of 2-imino-2H-chromene-3-carboxamide with phosphorus esters leads to the formation of various phosphonates and phosphinates through Michael addition mechanisms. These reactions often involve catalytic amounts of acids such as p-toluenesulfonic acid to promote cyclization and rearrangement processes.
Key Reaction Pathway:
-
Formation of an intermediate through nucleophilic attack.
-
Rearrangement leading to the formation of phosphonate derivatives.
This pathway has been shown to yield products such as chromenyl phosphonates and other complex derivatives .
Hydrazone Formation
Another notable reaction involves the synthesis of hydrazones from ethyl 2-oxo-2H-chromene-3-carboxylate when reacted with hydrazine hydrate. This reaction can produce multiple products, including malonohydrazide alongside the expected hydrazone, showcasing the versatility of the chromene structure in forming diverse chemical entities.
Example Reaction:
This reaction highlights the potential for further functionalization of the chromene scaffold in synthetic organic chemistry .
Characterization Techniques
The characterization of synthesized compounds typically involves various spectroscopic techniques:
NMR Spectroscopy
Nuclear Magnetic Resonance (NMR) spectroscopy is crucial for confirming the structure of synthesized compounds. For example, shifts in and NMR spectra provide insights into the environment of hydrogen and carbon atoms within the molecule, allowing for verification against expected values based on literature precedents.
Infrared Spectroscopy
Infrared (IR) spectroscopy is employed to identify functional groups present in the compounds. Characteristic absorption bands corresponding to N-H stretching (around 3300 cm) and C=O stretching (around 1650 cm) are indicative of successful amide formation .
Tables
Scientific Research Applications
Synthesis of 2H-Chromene-3-Carboxamide Derivatives
The synthesis of this compound derivatives typically involves coupling reactions between chromene-3-carboxylic acid derivatives and amines. For example, a study reported the synthesis of N-(2-hydroxyethyl)-2-oxo-2H-chromene-3-carboxamide using ultrasound irradiation as a green chemistry method, achieving an 83% yield under optimal conditions . This method not only enhances efficiency but also minimizes environmental impact.
Antimicrobial Activities
One of the most notable applications of this compound derivatives is their antimicrobial properties. Research has demonstrated that these compounds exhibit significant antibacterial and antifungal activities. For instance, a study evaluated various synthesized derivatives against gram-positive and gram-negative bacteria as well as fungi. Compounds such as 4a showed potent activity against Bacillus cereus with a minimum inhibitory concentration (MIC) of 0.062 mg/mL, outperforming standard drugs like streptomycin .
Table 1: Antimicrobial Activity of this compound Derivatives
Compound | Bacterial Strain | MIC (mg/mL) |
---|---|---|
4a | Bacillus cereus | 0.062 |
4b | Candida albicans | 0.125 |
4c | Aspergillus niger | 0.125 |
Antidiabetic Potential
Recent studies have explored the hypoglycemic effects of chromene derivatives, particularly their ability to inhibit α-amylase and α-glucosidase enzymes. A specific derivative, the 2-oxo-6-sulfamoyl-2H-chromene-3-carboxamide, demonstrated remarkable inhibitory activity against α-amylase, indicating its potential use in managing diabetes .
Table 2: α-Amylase Inhibitory Activity
Compound | Inhibitory Percentage (%) | IC50 (μM) |
---|---|---|
9 | 96.6 | 1.08 |
Acarbose | 96.5 | 0.43 |
Neuroprotective Effects
The neuroprotective potential of certain derivatives has also been investigated, particularly in relation to monoamine oxidase (MAO) inhibition. Compounds synthesized from this compound have shown selectivity towards MAO-B, which is relevant for treating neurodegenerative diseases like Parkinson's . The compound with the highest activity (IC50 = 0.93 μM) suggests that further development could lead to effective neuroprotective agents.
Antioxidant and Cytotoxic Activities
Additionally, some derivatives have exhibited antioxidant properties alongside cytotoxic effects against various cancer cell lines. For example, one study indicated that a specific chromenyl phosphonate exhibited significant antioxidant activity (IC50 = 2.8 µg/mL) and cytotoxicity against four cancer cell lines . This dual functionality positions these compounds as promising candidates for cancer therapeutics.
Mechanism of Action
The mechanism of action of 2H-chromene-3-carboxamide involves its interaction with specific molecular targets, such as enzymes or receptors. For example, it has been shown to inhibit pancreatic lipase by binding to the active site of the enzyme, thereby preventing the hydrolysis of triglycerides . This interaction is often mediated by hydrogen bonding and hydrophobic interactions with key amino acid residues in the enzyme’s active site .
Comparison with Similar Compounds
3-Oxo-3H-Benzo[f]chromene-2-Carboxamides
- Synthesis : 3-Oxo-3H-benzo[f]chromene-2-carboxamides are synthesized via Meldrum’s acid condensation with 2-hydroxy-1-naphthaldehyde, followed by conversion to acid chlorides and subsequent amidation. Yields exceed 90% for the carboxylic acid intermediate .
- Structural Features : The benzo[f]chromene scaffold introduces extended aromaticity compared to 2H-chromene, altering electronic properties. For example, compound 5a (N-(3,5-dimethylphenyl)-3-oxo-3H-benzo[f]chromene-2-carboxamide) exhibits distinct NMR shifts (δ 9.82 ppm for NH proton) due to conjugation effects .
- Applications : These derivatives are explored for anticancer activity but lack reported kinase inhibition, contrasting with 2H-chromene-3-carboxamide’s tyrosine kinase targeting .
Table 1: Comparison of Key Chromene Carboxamides
4H-Chromene-3-Carboxamides
- Synthesis: 4H-chromenes are synthesized via one-pot multicomponent reactions (e.g., aldehydes, malononitrile, and β-naphthol) using organocatalysts like potassium phthalimide-N-oxyl .
- Structural Differences : The saturated 4H-ring reduces π-conjugation, increasing stability but decreasing reactivity compared to 2H-chromenes.
- Bioactivity : Derivatives such as indolyl-4H-chromene-3-carboxamides exhibit antioxidant and antibacterial activity, differing from this compound’s kinase inhibition .
Substituent Effects on 2H-Chromene Derivatives
- Electron-Donating Groups : Methoxy or hydroxy substituents (e.g., 8-methoxy-2-oxo-2H-chromene-3-carboxamide) enhance solubility and alter UV-Vis absorption, critical for sensor applications .
Biological Activity
2H-chromene-3-carboxamide is a compound that has garnered significant interest in the field of medicinal chemistry due to its diverse biological activities. This article delves into the synthesis, biological evaluation, and potential therapeutic applications of this compound, supported by data tables and relevant case studies.
Synthesis of this compound Derivatives
The synthesis of this compound derivatives typically involves the condensation of salicylaldehyde with cyanoacetamide, followed by cyclization to form the chromene structure. Variations in substituents on the benzene ring and modifications at the carboxamide position can lead to compounds with enhanced biological properties.
1. Anticancer Activity
Several studies have highlighted the anticancer potential of this compound derivatives. For instance, a series of coumarin-3-carboxamide derivatives were synthesized and tested against various cancer cell lines, including HepG2 and HeLa. Notably, some derivatives exhibited IC50 values comparable to doxorubicin, a standard chemotherapeutic agent:
Compound | IC50 (μM) against HepG2 | IC50 (μM) against HeLa |
---|---|---|
14b | 2.62 | 0.39 |
14e | 4.85 | 0.75 |
Control (Doxorubicin) | - | - |
These results suggest that modifications to the carboxamide group significantly enhance anticancer activity, with specific substituents on the phenyl ring playing a crucial role in potency .
2. Antibacterial Activity
The antibacterial properties of this compound derivatives have also been investigated. A study reported moderate antibacterial activity against Gram-positive bacteria such as Staphylococcus aureus and Bacillus cereus. The minimum inhibitory concentration (MIC) values indicated that certain derivatives were effective at lower concentrations compared to standard antibiotics:
Compound | MIC (μg/mL) against Staphylococcus aureus |
---|---|
10 | 64 |
12 | 32 |
Control (Penicillin G) | <1 |
This highlights the potential of these compounds as new antibacterial agents .
3. Enzyme Inhibition
Recent research has explored the role of this compound derivatives as enzyme inhibitors. For example, a derivative was found to inhibit α-amylase effectively, which is significant for managing diabetes:
Compound | Inhibitory Percentage at 100 μg/mL | IC50 (μM) ± SD |
---|---|---|
9 | 96.6 | 1.08 ± 0.02 |
Acarbose | 96.5 | 0.43 ± 0.01 |
The high inhibitory activity suggests that these compounds could be developed into therapeutic agents for diabetes management .
The biological activities of this compound derivatives can be attributed to their ability to interact with specific biological targets, such as enzymes and receptors involved in cancer progression and bacterial growth. Molecular docking studies have shown that these compounds can bind effectively to active sites of target enzymes, demonstrating favorable binding energies and interactions with key amino acid residues .
Case Studies
Case Study: Anticancer Activity Evaluation
In a study evaluating various coumarin derivatives, researchers synthesized multiple analogs of this compound and assessed their anticancer activities through in vitro assays on different cancer cell lines. The study concluded that structural modifications led to significant variations in potency, emphasizing the importance of substituent effects on biological activity.
Case Study: Antimicrobial Properties
Another research effort focused on synthesizing and testing a series of new chromene derivatives for their antimicrobial properties against a range of pathogens. The results indicated that specific modifications enhanced activity against resistant strains, suggesting a promising avenue for developing new antibiotics based on this scaffold .
Q & A
Basic Research Questions
Q. What are the common synthetic routes for 2H-chromene-3-carboxamide derivatives, and how can reaction conditions be optimized for yield improvement?
- Methodological Answer : Synthesis typically involves cyclocondensation of substituted salicylaldehydes with active methylene compounds, followed by carboxamide functionalization. Key methods include:
-
Ru-catalyzed double C–H activation for regioselective alkylation (e.g., allylation) .
-
NHC (N-heterocyclic carbene)-catalyzed transformations to generate chromenone derivatives, optimized by adjusting solvent polarity (e.g., DMF vs. THF) and catalyst loading .
-
Coumarin-based routes using piperidine or quinoline amines for carboxamide coupling, with yields improved via microwave-assisted synthesis (e.g., 67% yield for HIV inhibitor derivatives) .
Optimization strategies: Use kinetic studies to identify rate-limiting steps, employ gradient temperature control, and substitute conventional heating with microwave irradiation to reduce side reactions.- Table 1: Representative Synthetic Methods
Substrate | Catalyst/Reagent | Yield (%) | Key Condition | Reference |
---|---|---|---|---|
2H-chromene-3-carboxaldehyde | Ru(II) complexes | 75–82 | Toluene, 100°C, 12h | |
7,8-Dihydroxy-6-nitro-derivative | Piperidine-4-amine | 67 | Microwave, 150°C, 30 min | |
Allylated derivative | NHC catalyst | 68 | DMF, 80°C, 6h |
Q. How can X-ray crystallography and Hirshfeld surface analysis be applied to determine the structural features of this compound derivatives?
- Methodological Answer :
- X-ray crystallography resolves bond lengths, angles, and packing interactions. For example, the crystal structure of 6-methyl-2-oxo-N-(quinolin-6-yl)-2H-chromene-3-carboxamide revealed planar chromene rings and intermolecular hydrogen bonds (N–H⋯O) stabilizing the lattice .
- Hirshfeld surface analysis quantifies intermolecular interactions (e.g., π-π stacking, C–H⋯O bonds). In the same study, >25% of interactions were attributed to H⋯O contacts, critical for crystal stability .
Q. What in vitro assays are recommended for initial screening of this compound's biological activity, such as MAO-B inhibition or antimicrobial effects?
- Methodological Answer :
- MAO-B inhibition : Use fluorometric assays with kynuramine as a substrate, comparing IC₅₀ values against reference inhibitors (e.g., selegiline). Derivatives with halogen substituents (e.g., Cl, Br) show enhanced activity due to hydrophobic interactions .
- Antimicrobial screening : Employ microdilution assays (MIC determination) against Gram-positive bacteria (e.g., S. aureus) and fungi (e.g., C. albicans). Derivatives with allyl or nitro groups exhibit improved activity .
Advanced Research Questions
Q. What computational strategies (e.g., DFT, molecular docking) are effective in predicting the binding affinity of this compound derivatives to targets like MAO-B?
- Methodological Answer :
- Density Functional Theory (DFT) : Calculate frontier molecular orbitals (HOMO-LUMO gaps) to assess electron-donating/withdrawing effects of substituents. For example, electron-withdrawing nitro groups reduce HOMO energy, enhancing binding to MAO-B’s FAD cofactor .
- Molecular docking : Use AutoDock Vina to simulate binding poses. Halogen substituents (e.g., Cl) in para positions show stronger van der Waals interactions with MAO-B’s hydrophobic pocket .
Q. How can structure-activity relationship (SAR) studies be systematically designed to identify critical functional groups in this compound derivatives for enhanced pharmacological activity?
- Methodological Answer :
- Step 1 : Synthesize a library with varied substituents (e.g., alkyl, aryl, halogens) at positions 6, 7, and 8 of the chromene ring.
- Step 2 : Test bioactivity in dose-response assays (e.g., IC₅₀ for MAO-B inhibition). For example, 8-allyl derivatives show 10-fold higher potency than unsubstituted analogs .
- Step 3 : Use multivariate regression to correlate electronic (Hammett σ) and steric (Taft Es) parameters with activity. Nitro groups at position 6 improve antiviral activity by enhancing π-stacking with HIV reverse transcriptase .
Q. What methodologies address discrepancies in biological activity data across different studies on this compound derivatives?
- Methodological Answer :
- Replicate assays under standardized conditions (e.g., cell line consistency, serum-free media). For instance, MAO-B inhibition assays vary based on enzyme source (recombinant vs. tissue-extracted) .
- Purity validation : Use HPLC (>95% purity) and LC-MS to confirm compound integrity. Impurities from incomplete allylation (e.g., in Ru-catalyzed reactions) may skew results .
- Meta-analysis : Compare datasets using statistical tools (e.g., Cohen’s d for effect size). Contradictions in antimicrobial activity often stem from differing bacterial strains or inoculum sizes .
Properties
IUPAC Name |
2H-chromene-3-carboxamide | |
---|---|---|
Source | PubChem | |
URL | https://pubchem.ncbi.nlm.nih.gov | |
Description | Data deposited in or computed by PubChem | |
InChI |
InChI=1S/C10H9NO2/c11-10(12)8-5-7-3-1-2-4-9(7)13-6-8/h1-5H,6H2,(H2,11,12) | |
Source | PubChem | |
URL | https://pubchem.ncbi.nlm.nih.gov | |
Description | Data deposited in or computed by PubChem | |
InChI Key |
UVEVMKODWDQIAN-UHFFFAOYSA-N | |
Source | PubChem | |
URL | https://pubchem.ncbi.nlm.nih.gov | |
Description | Data deposited in or computed by PubChem | |
Canonical SMILES |
C1C(=CC2=CC=CC=C2O1)C(=O)N | |
Source | PubChem | |
URL | https://pubchem.ncbi.nlm.nih.gov | |
Description | Data deposited in or computed by PubChem | |
Molecular Formula |
C10H9NO2 | |
Source | PubChem | |
URL | https://pubchem.ncbi.nlm.nih.gov | |
Description | Data deposited in or computed by PubChem | |
DSSTOX Substance ID |
DTXSID80144274 | |
Record name | Compound 69-20 | |
Source | EPA DSSTox | |
URL | https://comptox.epa.gov/dashboard/DTXSID80144274 | |
Description | DSSTox provides a high quality public chemistry resource for supporting improved predictive toxicology. | |
Molecular Weight |
175.18 g/mol | |
Source | PubChem | |
URL | https://pubchem.ncbi.nlm.nih.gov | |
Description | Data deposited in or computed by PubChem | |
CAS No. |
10185-00-9 | |
Record name | Delta(3)-chromene-3-carboxamide | |
Source | ChemIDplus | |
URL | https://pubchem.ncbi.nlm.nih.gov/substance/?source=chemidplus&sourceid=0010185009 | |
Description | ChemIDplus is a free, web search system that provides access to the structure and nomenclature authority files used for the identification of chemical substances cited in National Library of Medicine (NLM) databases, including the TOXNET system. | |
Record name | Compound 69-20 | |
Source | EPA DSSTox | |
URL | https://comptox.epa.gov/dashboard/DTXSID80144274 | |
Description | DSSTox provides a high quality public chemistry resource for supporting improved predictive toxicology. | |
Retrosynthesis Analysis
AI-Powered Synthesis Planning: Our tool employs the Template_relevance Pistachio, Template_relevance Bkms_metabolic, Template_relevance Pistachio_ringbreaker, Template_relevance Reaxys, Template_relevance Reaxys_biocatalysis model, leveraging a vast database of chemical reactions to predict feasible synthetic routes.
One-Step Synthesis Focus: Specifically designed for one-step synthesis, it provides concise and direct routes for your target compounds, streamlining the synthesis process.
Accurate Predictions: Utilizing the extensive PISTACHIO, BKMS_METABOLIC, PISTACHIO_RINGBREAKER, REAXYS, REAXYS_BIOCATALYSIS database, our tool offers high-accuracy predictions, reflecting the latest in chemical research and data.
Strategy Settings
Precursor scoring | Relevance Heuristic |
---|---|
Min. plausibility | 0.01 |
Model | Template_relevance |
Template Set | Pistachio/Bkms_metabolic/Pistachio_ringbreaker/Reaxys/Reaxys_biocatalysis |
Top-N result to add to graph | 6 |
Feasible Synthetic Routes
Disclaimer and Information on In-Vitro Research Products
Please be aware that all articles and product information presented on BenchChem are intended solely for informational purposes. The products available for purchase on BenchChem are specifically designed for in-vitro studies, which are conducted outside of living organisms. In-vitro studies, derived from the Latin term "in glass," involve experiments performed in controlled laboratory settings using cells or tissues. It is important to note that these products are not categorized as medicines or drugs, and they have not received approval from the FDA for the prevention, treatment, or cure of any medical condition, ailment, or disease. We must emphasize that any form of bodily introduction of these products into humans or animals is strictly prohibited by law. It is essential to adhere to these guidelines to ensure compliance with legal and ethical standards in research and experimentation.