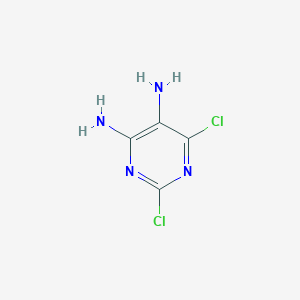
2,6-Dichloropyrimidine-4,5-diamine
Overview
Description
2,6-Dichloropyrimidine-4,5-diamine is a heterocyclic compound featuring a pyrimidine backbone with chlorine atoms at positions 2 and 6 and amino groups at positions 4 and 3. This structure renders it highly reactive in nucleophilic substitution reactions, making it a valuable intermediate in organic synthesis, particularly for constructing unsymmetrically substituted pyrimidines . The dichloro groups facilitate sequential amine substitutions under controlled conditions, enabling the synthesis of diverse derivatives for applications in medicinal chemistry and materials science.
Preparation Methods
Chlorination of 2,5-Diamino-4,6-dihydroxypyrimidine Hydrochloride
Reagent Systems and Stoichiometry
The chlorination process employs phosphorus oxychloride as the chlorinating agent, paired with a quaternary ammonium chloride (e.g., methyltriethylammonium chloride) or a weak tertiary amine base hydrochloride (e.g., diethylaniline hydrochloride) . Optimal results require 3–6 molar equivalents of POCl₃ and 2–6 equivalents of the ammonium salt relative to the dihydroxypyrimidine substrate. Excess POCl₃ acts both as a reactant and solvent, facilitating complete substitution of hydroxyl groups at positions 4 and 6.
Reaction Conditions and Kinetics
The reaction proceeds at elevated temperatures (100–105°C) under anhydrous conditions, typically over 24–30 hours . Prolonged heating ensures complete conversion, while ultrasonication may accelerate the process. Key parameters include:
Parameter | Optimal Range | Effect on Yield |
---|---|---|
Temperature | 100–105°C | Higher temperatures reduce reaction time but risk decomposition |
Reaction Time | 24–30 hours | Shorter durations yield mono-chlorinated byproducts |
POCl₃ Equivalents | 5–6 mol/mol substrate | Substoichiometric amounts lead to incomplete chlorination |
Large-Scale Synthesis: Case Studies
Example 1 (Patent EP0483204B1):
-
Substrate: 520 g vacuum-dried 2,5-diamino-4,6-dihydroxypyrimidine hydrochloride
-
Reagents: 700 mL POCl₃, 500 g methyltriethylammonium chloride
-
Procedure: Heating at 105°C for 28 hours, followed by vacuum distillation to remove excess POCl₃. Quenching in ice-water, pH adjustment to 7, and ethyl acetate extraction yielded 131 g (65%) of product .
Example 2:
Example 3:
Alternative Chlorination Strategies
Tertiary Amine Hydrochloride Systems
Substituting quaternary salts with tertiary amine hydrochlorides (e.g., diethylaniline hydrochloride) produced mixed results. High-performance liquid chromatography (HPLC) revealed a 1:1 ratio of 2,5-diamino-4-chloro-6-hydroxypyrimidine and the desired dichloro product, underscoring the inferiority of tertiary bases in achieving complete substitution .
Solvent Effects
While the reaction is ideally solvent-free, polar aprotic solvents like acetonitrile enhance solubility and heat transfer. Trials with tetrahydrofuran or dioxane resulted in slower kinetics due to reduced POCl₃ activity.
Mechanistic Insights
The chlorination follows a nucleophilic aromatic substitution (SNAr) mechanism:
-
Activation: POCl₃ reacts with the ammonium salt to generate a chlorophosphate intermediate, enhancing the electrophilicity of the pyrimidine ring.
-
Substitution: Chloride ions sequentially displace hydroxyl groups at positions 4 and 6, with the ammonium salt neutralizing liberated HCl .
Rate-Limiting Step: The first chlorination (C4–OH → Cl) is slower due to steric hindrance from the adjacent C5–NH₂ group.
Industrial-Scale Optimization
Process Intensification
-
Continuous Flow Reactors: Minimize thermal degradation by reducing residence time at high temperatures.
-
In Situ HCl Removal: Vacuum distillation or gas sparging improves reaction completeness.
Purification Protocols
Post-reaction workup involves:
-
Quenching: Controlled addition to ice-water prevents exothermic runaway.
-
Extraction: Ethyl acetate selectively isolates the product, with silica plug filtration removing polymeric byproducts .
-
Crystallization: Concentrating the organic phase yields high-purity crystals (97.1 g initial batch, 34.2 g secondary).
Characterization and Quality Control
Spectroscopic Data
-
¹³C NMR (DMSO-d₆): Peaks at 126.04 ppm (C4/6), 145.52 ppm (C2/5), 154.30 ppm (C1/3) .
-
Mass Spectrometry: Molecular ion cluster at m/z 178, 180, 182 (M⁺, Cl₂ isotopic pattern) .
Elemental Analysis
Element | Calculated (%) | Observed (%) |
---|---|---|
C | 26.84 | 26.65 |
H | 2.25 | 2.40 |
N | 31.30 | 31.40 |
Cl | 39.60 | 39.50 |
Challenges and Mitigation Strategies
Moisture Sensitivity
The substrate and reagents require rigorous drying (e.g., 24-hour vacuum drying at 85°C) to prevent hydrolysis of POCl₃ to phosphoric acid, which deactivates the chlorinating agent .
Byproduct Formation
Black phosphorous-containing solids, removed via Celite filtration, arise from side reactions between POCl₃ and residual moisture.
Chemical Reactions Analysis
Nucleophilic Substitution Reactions
The chlorine atoms at positions 2 and 6 undergo nucleophilic substitution (S<sub>N</sub>Ar) with amines, alkoxides, and thiols. Reaction conditions and outcomes vary based on the nucleophile and solvent system.
Key Examples:
Mechanistic Notes :
-
Reactions with bulky amines (e.g., adamantane derivatives) require elevated temperatures and extended reaction times due to steric hindrance .
-
Quaternary ammonium salts (e.g., methyltriethylammonium chloride) enhance reactivity in phosphorus oxychloride-mediated substitutions .
Hydrolysis Reactions
Controlled hydrolysis under acidic or basic conditions selectively replaces chlorine with hydroxyl groups.
Experimental Data:
Critical Factors :
-
pH control (pH 3–4) minimizes displacement of chloro groups by amines during hydrolysis .
-
Organic solvents (e.g., toluene, dichloroethane) stabilize intermediates and prevent decomposition .
Reduction Reactions
Catalytic hydrogenation reduces the pyrimidine ring, though competing degradation pathways are common.
Catalytic Hydrogenation Data:
Challenges :
-
Degradation products (e.g., propionaldehyde, ammonia) form due to ring-opening under acidic conditions .
-
Low yields arise from competing solvolysis and side reactions.
Condensation and Cyclization
The amino groups participate in condensation reactions to form fused heterocycles.
Case Study: Claisen-Schmidt Condensation
-
Reactants : 2-Amino-4,6-dichloropyrimidine-5-carbaldehyde + acetophenone
-
Conditions : NaOH (aq), ethanol, 60°C
-
Product : 2-Amino-4,6-dichloro-5-(3-phenylacryloyl)pyrimidine
-
Yield : 68%
Applications :
-
Forms α,β-unsaturated ketones for further functionalization.
-
Enables synthesis of bioactive pyrimidine derivatives.
Stability and Reactivity Trends
Industrial-Scale Considerations
Scientific Research Applications
Antiviral Applications
2,6-Dichloropyrimidine-4,5-diamine has been identified as a crucial intermediate for synthesizing antiviral nucleoside derivatives. These derivatives have shown efficacy against several viral infections, including HIV and hepatitis B virus (HBV). For instance, the compound is utilized in the preparation of 9-substituted-2-aminopurines, which are known to exhibit potent antiviral activity .
Case Study: HIV and HBV Treatments
Research indicates that certain nucleoside analogues derived from this compound have been effective in inhibiting the replication of HIV and HBV. These compounds work by mimicking natural nucleosides, thereby interfering with viral RNA synthesis.
Anticancer Properties
The compound has also garnered attention for its potential in cancer treatment. Pyrimidine derivatives, including those derived from this compound, have demonstrated activity against various cancer cell lines by inhibiting key growth factors and pathways involved in tumor proliferation.
Case Study: EGFR Inhibition
Substituted pyrimidines have been shown to block the epidermal growth factor receptor (EGFR), a critical target in lung cancer therapy. Studies have reported that these compounds can effectively inhibit mutant forms of EGFR, which are often resistant to standard treatments .
Synthesis of Pharmaceutical Intermediates
This compound serves as a versatile building block in synthesizing various pharmaceutical intermediates. Its reactivity allows for the introduction of diverse functional groups through electrophilic substitutions or coupling reactions.
Applications in Drug Development
- Antibiotics: Recent advancements have seen the use of this compound in developing new antibiotics targeting resistant bacterial strains.
- Anti-inflammatory Agents: Research has indicated that derivatives of this compound exhibit significant anti-inflammatory activity by inhibiting cyclooxygenase enzymes (COX-1 and COX-2), which play a role in pain and inflammation pathways .
Structure–Activity Relationships (SAR)
Understanding the structure–activity relationships of this compound derivatives is crucial for optimizing their pharmacological properties. Variations in substituents can significantly affect their biological activity and selectivity.
Key Findings
- Electron-withdrawing groups enhance the potency of certain derivatives against specific targets.
- The presence of halogens has been linked to increased lipophilicity and improved membrane permeability .
Summary Table of Applications
Application Type | Compound Role | Key Findings |
---|---|---|
Antiviral | Intermediate for nucleoside analogues | Effective against HIV and HBV |
Anticancer | EGFR inhibitors | Potent against lung cancer cell lines |
Antibiotic Development | Building block for new antibiotics | Targeting resistant bacteria |
Anti-inflammatory | COX inhibitors | Significant reduction in inflammation markers |
Mechanism of Action
The mechanism of action of 2,6-dichloropyrimidine-4,5-diamine involves its interaction with specific molecular targets. For instance, it can inhibit certain enzymes by binding to their active sites, thereby blocking their activity. This inhibition can lead to various biological effects, depending on the enzyme targeted .
Comparison with Similar Compounds
2,6-Diamino-4-methoxy Pyrimidine (CAS 3270-97-1)
Structural Differences :
- Substituents: Methoxy group at position 4 and amino groups at positions 2 and 6 (vs. 2,6-dichloro and 4,5-diamine in the target compound).
- Reactivity : The methoxy group is electron-donating, reducing electrophilicity at the pyrimidine ring compared to electron-withdrawing chlorine atoms. This diminishes reactivity in substitution reactions but enhances stability.
4,5-Diamino-2,6-dimercaptopyrimidine (CAS 31295-41-7)
Structural Differences :
- Substituents: Mercapto (-SH) groups at positions 2 and 6 (vs. Cl) and amino groups at 4 and 4.
- Reactivity : Thiol groups participate in redox reactions and metal coordination, enabling applications in catalysis and heavy metal chelation.
3,5-Diamino-2,6-dimethoxypyridine Dihydrochloride
Structural Differences :
- Core Structure: Pyridine ring (vs. pyrimidine), with methoxy groups at positions 2 and 6 and amino groups at 3 and 5.
- Reactivity : The pyridine ring is less electron-deficient than pyrimidine, reducing susceptibility to nucleophilic attack.
4,6-Diamino-2-methyl-5-nitropyrimidine
Structural Differences :
- Substituents: Methyl at position 2, nitro at position 5, and amino groups at 4 and 6.
- Reactivity : The nitro group enhances electrophilicity, facilitating aromatic substitution reactions at position 5.
Key Research Findings
- Reactivity Hierarchy : Chloro-substituted pyrimidines (e.g., 2,6-dichloropyrimidine-4,5-diamine) exhibit higher reactivity in substitution reactions than methoxy- or thiol-substituted analogs due to stronger electron-withdrawing effects .
- Virtual Screening Insights: Pyrimidine derivatives with amino groups show moderate affinity in epigenetic target inhibition (e.g., DNMT inhibitors), but 5-membered heterocycles (e.g., triazoles) often outperform them in binding affinity .
Biological Activity
2,6-Dichloropyrimidine-4,5-diamine (DCDA) is a heterocyclic compound with significant potential in medicinal chemistry, particularly as a precursor for various biologically active molecules. This article explores the biological activity of DCDA, focusing on its synthesis, mechanisms of action, and therapeutic applications.
Chemical Structure and Properties
DCDA has the molecular formula CHClN and features two chlorine atoms at the 2 and 6 positions of the pyrimidine ring, with amino groups at the 4 and 5 positions. This unique substitution pattern contributes to its reactivity and biological properties.
The biological activity of DCDA is primarily attributed to its ability to interact with various molecular targets within cells. These interactions can lead to:
- Inhibition of Enzymatic Activity : DCDA has been shown to inhibit specific enzymes involved in nucleotide metabolism, which can affect cell proliferation and survival.
- Antiviral Activity : As a precursor in the synthesis of antiviral agents, DCDA's derivatives have demonstrated efficacy against viral infections by interfering with viral replication mechanisms.
- Anticancer Properties : Research indicates that DCDA derivatives can induce apoptosis in cancer cells by activating specific signaling pathways.
Biological Activity Data
The following table summarizes key findings related to the biological activity of DCDA and its derivatives:
Case Studies
- FLT3 Inhibition Study : In a study evaluating the potential of pyrimidine derivatives for inhibiting FLT3 (Fms-like tyrosine kinase 3), DCDA was identified as a promising scaffold. The compound demonstrated significant inhibition in FLT3-driven cell lines, suggesting its utility in treating acute myeloid leukemia (AML) .
- Antiviral Research : DCDA has been investigated for its role in synthesizing antiviral compounds. A derivative showed promising results against influenza viruses, highlighting its potential in developing new antiviral therapies .
- Cancer Therapeutics : In studies involving various cancer cell lines, DCDA derivatives exhibited cytotoxic effects through apoptosis induction. These findings support further exploration into its application as an anticancer agent .
Synthesis and Derivatives
DCDA serves as a precursor for numerous derivatives with enhanced biological activity. Common synthetic routes include:
Q & A
Basic Research Questions
Q. What are the key considerations for synthesizing 2,6-Dichloropyrimidine-4,5-diamine derivatives?
- Methodological Answer : Derivatives can be synthesized via microwave-assisted reactions. For example, a two-step process involves heating this compound in ethyl orthoacetate (EtC(OEt)₃) at 160°C for 1 hour, followed by substitution with tert-butylamine (t-BuNH₂) in N-methylpyrrolidone (NMP) under similar conditions. Purification via flash chromatography (SiO₂, DCM/MeOH 98:2) is critical to isolate intermediates and final products. Monitoring reaction progress with NMR (e.g., characteristic δ 13.13 ppm for NH protons) and ESI–MS ensures structural fidelity .
Q. How should researchers characterize this compound derivatives?
- Methodological Answer : Use a combination of spectral techniques:
- ¹H/¹³C NMR : Identify substituents via chemical shifts (e.g., δ 1.57 ppm for tert-butyl groups) and coupling patterns.
- ESI–MS : Confirm molecular weights (e.g., m/z 254.24 [M+H]⁺ for N-(tert-butyl)-2-chloro-8-ethyl-9H-purin-6-amine).
- Chromatography : Monitor purity via HPLC or TLC (e.g., SiO₂ with DCM/MeOH gradients).
Consistency in spectral data across batches is essential to validate synthetic reproducibility .
Q. What safety precautions are necessary when handling this compound?
- Methodological Answer :
- Personal Protective Equipment (PPE) : Wear nitrile gloves, lab coats, and safety goggles to avoid skin/eye contact.
- Ventilation : Use fume hoods for reactions involving volatile solvents (e.g., NMP, EtOAc).
- Waste Management : Segregate halogenated waste for professional disposal to prevent environmental contamination.
- Emergency Protocols : Rinse exposed skin/eyes with water for 15 minutes and seek medical attention if irritation persists .
Advanced Research Questions
Q. How can substitution reactions on this compound be optimized for regioselectivity?
- Methodological Answer : Regioselectivity in substitutions (e.g., at C4 vs. C5 positions) depends on:
- Solvent Polarity : Polar aprotic solvents (e.g., DMF, NMP) favor nucleophilic attack at electron-deficient positions.
- Catalysts : Transition-metal catalysts (e.g., Pd or Cu) enhance coupling efficiency in cross-coupling reactions.
- Temperature : Microwave-assisted heating (160°C) accelerates kinetics while reducing side reactions.
Systematic screening of reaction conditions (e.g., amine equivalents, solvent ratios) is recommended to optimize yields .
Q. What strategies validate the biological activity of this compound derivatives?
- Methodological Answer :
- Enzyme Assays : Test inhibition of target enzymes (e.g., topoisomerase II) using fluorescence-based assays (IC₅₀ determination).
- Cellular Studies : Evaluate cytotoxicity in cancer cell lines (e.g., IC₅₀ via MTT assays) and compare to control compounds.
- Molecular Docking : Use software (e.g., AutoDock) to predict binding modes to active sites (e.g., ATP-binding pockets).
Correlate structural modifications (e.g., chloro vs. methoxy substituents) with activity trends to guide SAR studies .
Q. How can researchers resolve contradictions in synthetic yields across different batches?
- Methodological Answer :
- Purity Analysis : Use HPLC to detect impurities (e.g., unreacted starting material) that may depress yields.
- Reagent Quality : Ensure anhydrous conditions for moisture-sensitive steps (e.g., tert-butylamine reactions).
- Reaction Monitoring : Employ in-situ FTIR or LC-MS to identify kinetic bottlenecks (e.g., incomplete substitution).
Document deviations (e.g., temperature fluctuations) and perform Design of Experiments (DoE) to isolate critical variables .
Properties
IUPAC Name |
2,6-dichloropyrimidine-4,5-diamine | |
---|---|---|
Source | PubChem | |
URL | https://pubchem.ncbi.nlm.nih.gov | |
Description | Data deposited in or computed by PubChem | |
InChI |
InChI=1S/C4H4Cl2N4/c5-2-1(7)3(8)10-4(6)9-2/h7H2,(H2,8,9,10) | |
Source | PubChem | |
URL | https://pubchem.ncbi.nlm.nih.gov | |
Description | Data deposited in or computed by PubChem | |
InChI Key |
VJVAQTIZGIIBCM-UHFFFAOYSA-N | |
Source | PubChem | |
URL | https://pubchem.ncbi.nlm.nih.gov | |
Description | Data deposited in or computed by PubChem | |
Canonical SMILES |
C1(=C(N=C(N=C1Cl)Cl)N)N | |
Source | PubChem | |
URL | https://pubchem.ncbi.nlm.nih.gov | |
Description | Data deposited in or computed by PubChem | |
Molecular Formula |
C4H4Cl2N4 | |
Source | PubChem | |
URL | https://pubchem.ncbi.nlm.nih.gov | |
Description | Data deposited in or computed by PubChem | |
DSSTOX Substance ID |
DTXSID40286462 | |
Record name | 4,5-Diamino-2,6-dichloropyrimidine | |
Source | EPA DSSTox | |
URL | https://comptox.epa.gov/dashboard/DTXSID40286462 | |
Description | DSSTox provides a high quality public chemistry resource for supporting improved predictive toxicology. | |
Molecular Weight |
179.00 g/mol | |
Source | PubChem | |
URL | https://pubchem.ncbi.nlm.nih.gov | |
Description | Data deposited in or computed by PubChem | |
CAS No. |
130838-36-7 | |
Record name | 4,5-Diamino-2,6-dichloropyrimidine | |
Source | EPA DSSTox | |
URL | https://comptox.epa.gov/dashboard/DTXSID40286462 | |
Description | DSSTox provides a high quality public chemistry resource for supporting improved predictive toxicology. | |
Synthesis routes and methods
Procedure details
Retrosynthesis Analysis
AI-Powered Synthesis Planning: Our tool employs the Template_relevance Pistachio, Template_relevance Bkms_metabolic, Template_relevance Pistachio_ringbreaker, Template_relevance Reaxys, Template_relevance Reaxys_biocatalysis model, leveraging a vast database of chemical reactions to predict feasible synthetic routes.
One-Step Synthesis Focus: Specifically designed for one-step synthesis, it provides concise and direct routes for your target compounds, streamlining the synthesis process.
Accurate Predictions: Utilizing the extensive PISTACHIO, BKMS_METABOLIC, PISTACHIO_RINGBREAKER, REAXYS, REAXYS_BIOCATALYSIS database, our tool offers high-accuracy predictions, reflecting the latest in chemical research and data.
Strategy Settings
Precursor scoring | Relevance Heuristic |
---|---|
Min. plausibility | 0.01 |
Model | Template_relevance |
Template Set | Pistachio/Bkms_metabolic/Pistachio_ringbreaker/Reaxys/Reaxys_biocatalysis |
Top-N result to add to graph | 6 |
Feasible Synthetic Routes
Disclaimer and Information on In-Vitro Research Products
Please be aware that all articles and product information presented on BenchChem are intended solely for informational purposes. The products available for purchase on BenchChem are specifically designed for in-vitro studies, which are conducted outside of living organisms. In-vitro studies, derived from the Latin term "in glass," involve experiments performed in controlled laboratory settings using cells or tissues. It is important to note that these products are not categorized as medicines or drugs, and they have not received approval from the FDA for the prevention, treatment, or cure of any medical condition, ailment, or disease. We must emphasize that any form of bodily introduction of these products into humans or animals is strictly prohibited by law. It is essential to adhere to these guidelines to ensure compliance with legal and ethical standards in research and experimentation.