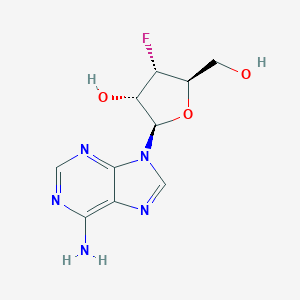
3'-Fluoro-3'-deoxyadenosine
Overview
Description
3'-Fluoro-3'-deoxyadenosine (CAS: 75059-22-2; molecular formula: C₁₀H₁₂FN₅O₃) is a fluorinated nucleoside analog characterized by the substitution of a fluorine atom at the 3'-position of the ribose moiety, replacing the hydroxyl group. This structural modification enhances metabolic stability and alters biological activity compared to unmodified adenosine . The compound has demonstrated potent antiviral activity against poliovirus, coxsackievirus, Colorado tick fever virus, and orbiviruses, though its exact mechanism remains unclear. Synthetically, regioselective fluorination methods have been developed, such as the stereospecific introduction of fluorine via phosphonomethoxy group modifications or bromine-to-fluorine rearrangements .
Preparation Methods
Synthetic Routes and Reaction Conditions: The synthesis of 3’-Deoxy-3’-fluoroadenosine typically involves a multi-step process. One common method starts with methyl 2-O-benzyl-5-O-t-butyldimethylsilyl-β-D-xylofuranoside. The key steps include:
- Activation of the O-3 position by forming a triflate.
- Nucleophilic displacement to introduce the fluoride ion in a stereospecific manner at the C-3 position .
- Desilylation and acidic methanolysis in a single pot to yield the final product .
Industrial Production Methods: Industrial production methods for 3’-Deoxy-3’-fluoroadenosine are not extensively documented in the public domain. the synthetic routes used in laboratory settings can be scaled up with appropriate modifications to reaction conditions and purification processes to meet industrial demands.
Chemical Reactions Analysis
Fluorination Using DAST
DAST is critical for introducing fluorine while preserving the nucleoside’s bioactive conformation:
-
Mechanism : DAST reacts with the 3′-hydroxyl group, replacing it with fluorine via an S<sub>N</sub>2 pathway, confirmed by <sup>19</sup>F NMR .
-
Stereochemical Outcome : Inversion at C3′ ensures the β-<i>arabino</i> configuration, essential for antiviral activity .
Structural and Conformational Analysis
The 3′-fluoro substituent significantly alters the ribose ring’s conformation:
-
North vs. South Conformation : 3′-F-dA adopts a North-type (C3′-endo) conformation due to hyperconjugative interactions between σ<sub>C–F</sub> and σ*<sub>C–O</sub> orbitals . This stabilizes the analog’s binding to viral polymerases.
-
X-ray Crystallography : Confirms the fluorine atom’s axial position, enhancing base stacking and hydrogen bonding in RNA-like structures .
Antiviral Activity Correlated with Chemical Modifications
3′-F-dA’s efficacy stems from its resistance to enzymatic cleavage and ability to inhibit viral RNA synthesis:
Scientific Research Applications
Antiviral Activity
3'-Fluoro-3'-deoxyadenosine has demonstrated significant antiviral properties against a range of viruses, including both RNA and DNA viruses. Key findings include:
- Broad-Spectrum Efficacy : The compound exhibits antiviral activity against tick-borne encephalitis virus (TBEV), Zika virus, and West Nile virus (WNV), with effective concentrations ranging from 1.1 μM to 4.7 μM in cell cultures. Notably, it has shown no cytotoxicity at concentrations up to 25 μM, although it does exhibit cytostatic effects at higher doses .
- In Vivo Studies : Research indicates that 3'-F-3'-dAdo is effective in vivo, inhibiting viral replication in mouse models infected with TBEV and WNV. This suggests its potential as a therapeutic candidate for treating flavivirus infections .
Synthesis and Chemical Properties
The synthesis of 3'-F-3'-dAdo has been achieved through various methods, including:
- Triflate Activation : One synthesis route involves converting adenosine into a protected intermediate, followed by triflate activation and subsequent fluorination using diethylaminosulfur trifluoride (DAST). This method allows for efficient production of the compound for research purposes .
Comparative Analysis with Other Nucleosides
To understand the unique aspects of 3'-F-3'-dAdo, it is useful to compare it with other nucleoside analogs:
Compound Name | Key Features | Unique Aspects |
---|---|---|
2',3'-Dideoxycytidine | Lacks hydroxyl groups at 2' and 3' positions | Primarily used as an antiviral agent |
2',3'-Dideoxyadenosine | Similar structure but without fluorination | Commonly used in HIV treatments |
2-Fluoro-2'-deoxyadenosine | Fluorination at the 2' position | Different antiviral spectrum |
3'-Azido-3'-deoxyadenosine | Contains an azido group instead of fluorine | Used in nucleic acid labeling |
The unique feature of 3'-F-3'-dAdo lies in its specific antiviral activity profile and lower cytotoxicity compared to its analogs, making it particularly promising for therapeutic development against viral infections .
Mechanism of Action
The mechanism of action of 3’-Deoxy-3’-fluoroadenosine involves its incorporation into nucleic acids, leading to the inhibition of DNA synthesis and induction of apoptosis. This compound targets essential viral or cellular enzymes active in nucleic acid replication and transcription, thereby exerting its antiviral and antitumor effects .
Comparison with Similar Compounds
The following analysis compares 3'-fluoro-3'-deoxyadenosine with structurally or functionally related nucleoside analogs, focusing on synthesis, biological activity, and mechanisms.
3'-Substituted Deoxyadenosine Analogs
Key Differences :
- Activity Spectrum: this compound shows broader antiviral activity compared to cordycepin, which is primarily used in antitrypanosomal and cancer research.
- Mechanism: While cordycepin directly incorporates into nucleic acids, this compound may act via enzyme inhibition .
- Synthesis: 3'-amino derivatives require multi-step coupling with Fmoc-amino acids (up to 10 steps, 23% yield), whereas fluorinated analogs utilize regioselective fluorination for efficiency .
Fluorinated Nucleoside Analogs
Key Differences :
- Application: FLT is used diagnostically in oncology, whereas this compound is therapeutic against RNA viruses.
- Stereochemistry: The α-fluorine configuration in 2',3'-dideoxyadenosine may reduce antiviral efficacy compared to β-fluorine in this compound .
Other Antiviral Nucleosides
Key Differences :
- Nucleobase Specificity: 3-Deazaguanine targets guanine-dependent processes, while this compound disrupts adenosine metabolism .
- Mechanistic Clarity: Ribavirin’s RNA mutagenesis is well-characterized, whereas this compound’s mechanism remains speculative .
Pharmacological Modifications
- N6-Substituted 3’-Fluoro Analogues: Addition of an N6-(3-iodobenzyl) group converts 3’-fluoro-3’-deoxyadenosine into a partial agonist or antagonist at A3 adenosine receptors, demonstrating structural sensitivity in receptor binding .
Biological Activity
3'-Fluoro-3'-deoxyadenosine (3'-F-3'-dA) is a nucleoside analogue that has garnered significant attention due to its antiviral and potential anticancer properties. This article provides a comprehensive overview of its biological activity, supported by research findings, case studies, and data tables.
Chemical Structure and Properties
3'-F-3'-dA is characterized by the substitution of a fluorine atom at the 3' position of the ribose sugar, which alters its biochemical interactions compared to natural adenosine. This modification enhances its stability against enzymatic degradation and influences its interaction with viral and cellular targets.
Research indicates that 3'-F-3'-dA exhibits broad-spectrum antiviral activity against several viruses, including flaviviruses such as the Tick-Borne Encephalitis Virus (TBEV), Zika Virus (ZIKV), and West Nile Virus (WNV). The compound acts primarily by inhibiting viral replication through incorporation into viral RNA, leading to termination of viral genome synthesis.
In Vitro Studies
In vitro studies have demonstrated that 3'-F-3'-dA has low-micromolar antiviral effects, with effective concentrations (EC50) ranging from 1.1 ± 0.1 μM to 4.7 ± 1.5 μM against various viruses . Importantly, it exhibits minimal cytotoxicity up to concentrations of 25 μM, although it does show cytostatic effects at higher concentrations (>12.5 μM) .
Virus | EC50 (μM) | Cytotoxicity | Notes |
---|---|---|---|
Tick-Borne Encephalitis | 1.1 ± 0.1 | Low | Effective in neural cell lines |
Zika Virus | 4.7 ± 1.5 | Low | Significant antiviral effect |
West Nile Virus | Variable | Low | Broad-spectrum efficacy |
In Vivo Studies
In vivo studies further support the antiviral efficacy of 3'-F-3'-dA. For instance, it has been shown to inhibit tail lesion formation in mice infected with vaccinia virus . Additionally, it demonstrated significant antiviral activity in mouse models for TBEV and WNV infections, reinforcing its potential as a therapeutic agent against emerging viral threats .
Anticancer Activity
While primarily studied for its antiviral properties, 3'-F-3'-dA also exhibits anticancer activity. It has been reported to induce apoptosis in various cancer cell lines through mechanisms involving adenosine receptors and death receptors . The compound's ability to modulate cellular pathways associated with tumor growth presents opportunities for further exploration in cancer therapy.
Case Studies
Several case studies have highlighted the effectiveness of 3'-F-3'-dA in both laboratory and clinical settings:
- Case Study on Flavivirus Inhibition : A study evaluated the compound's efficacy against ZIKV in vitro and found that it significantly reduced viral load in infected cells without causing cytotoxic effects .
- Anticancer Efficacy : Another investigation focused on glioma cell lines where 3'-F-3'-dA was shown to activate apoptotic pathways, suggesting potential for use in glioblastoma treatment .
Q & A
Basic Research Questions
Q. What are the established synthetic routes for 3'-Fluoro-3'-deoxyadenosine, and what key challenges exist in achieving high purity?
The synthesis of this compound typically involves fluorination of deoxyadenosine precursors using reagents like DAST (diethylaminosulfur trifluoride) or fluorinated building blocks. Key challenges include regioselective fluorination at the 3'-position and minimizing side reactions. Purification often requires reverse-phase HPLC or column chromatography to separate isomers and byproducts. Stability during synthesis must be monitored due to the compound’s sensitivity to hydrolysis .
Q. Which in vitro assays are commonly used to evaluate the antiviral activity of this compound?
Standard assays include plaque reduction assays in poliovirus and coxsackievirus models, cytotoxicity assessments in WI-38 or Vero cells, and inhibition of viral replication via RT-PCR quantification. EC50 values are determined using dose-response curves, with parallel cytotoxicity profiling (e.g., CC50 in uninfected cells) to establish selectivity indices .
Q. How does fluorination at the 3'-position influence the metabolic stability of deoxyadenosine analogues?
The 3'-fluoro substitution enhances metabolic stability by resisting enzymatic deamination and phosphorylase cleavage. Comparative studies with non-fluorinated analogues (e.g., 3'-deoxyadenosine) show prolonged half-life in plasma and reduced catabolism in liver microsomes. Stability assays using LC-MS or NMR track degradation products under physiological conditions .
Advanced Research Questions
Q. How can transcriptomic and epigenomic profiling elucidate the role of this compound in modulating circadian rhythms and aging?
Transcriptomic analysis (RNA-seq) of hypothalamic tissues in aging mice reveals differential expression of circadian genes (e.g., Bmal1, Per2) and metabolic regulators. Epigenomic profiling (bisulfite sequencing or MeDIP-seq) identifies DNA methylation changes at promoters of aging-related genes (e.g., Sirt1). Whole-brain c-FOS+ mapping can pinpoint neural activation patterns in the paraventricular nucleus (PVN), linking circadian modulation to lifespan extension .
Q. What experimental approaches are recommended to resolve contradictions regarding the molecular targets of this compound's antiviral action?
Mechanistic studies should combine enzymatic inhibition assays (e.g., testing activity against S-adenosyl-L-homocysteine hydrolase), siRNA knockdown of putative targets, and structural analysis (X-ray crystallography or molecular docking). Comparative metabolomics in infected vs. treated cells can identify disrupted pathways (e.g., nucleotide pools, methylation cycles) .
Q. What methodologies are optimal for assessing the impact of this compound on DNA methylation patterns in aging models?
Use reduced-representation bisulfite sequencing (RRBS) or whole-genome bisulfite sequencing to quantify methylation changes in tissues like liver or brain. Validate findings with pyrosequencing of candidate loci (e.g., age-related CpG islands). Correlate methylation shifts with phenotypic outcomes (e.g., locomotor activity, hormone levels) in high-fat diet or progeria mouse models .
Q. What in vivo models and parameters should be prioritized when investigating the lifespan-extending effects of this compound?
Prioritize aged C57BL/6 mice or premature aging models (e.g., Ercc1 mutants). Monitor lifespan, frailty indices, and metabolic parameters (e.g., energy expenditure via indirect calorimetry). Include histological analysis of senescence markers (SA-β-gal, p16INK4a) in tissues like liver and muscle. Pair with circadian rhythm tracking (wheel-running assays) to assess synchronization .
Q. How can chemogenetic and optogenetic tools be integrated to map the neural circuits affected by this compound?
Use chemogenetic actuators (DREADDs) or optogenetic probes (Channelrhodopsin) in PVN-specific Cre mouse lines. Combine with c-FOS immunofluorescence to map acute neural activation. Behavioral assays (e.g., feeding, locomotor activity) under light/dark cycles can link circuit modulation to circadian outputs. Validate with pharmacological inhibition of candidate pathways (e.g., melanocortin receptors) .
Properties
IUPAC Name |
(2R,3S,4S,5R)-2-(6-aminopurin-9-yl)-4-fluoro-5-(hydroxymethyl)oxolan-3-ol | |
---|---|---|
Source | PubChem | |
URL | https://pubchem.ncbi.nlm.nih.gov | |
Description | Data deposited in or computed by PubChem | |
InChI |
InChI=1S/C10H12FN5O3/c11-5-4(1-17)19-10(7(5)18)16-3-15-6-8(12)13-2-14-9(6)16/h2-5,7,10,17-18H,1H2,(H2,12,13,14)/t4-,5-,7-,10-/m1/s1 | |
Source | PubChem | |
URL | https://pubchem.ncbi.nlm.nih.gov | |
Description | Data deposited in or computed by PubChem | |
InChI Key |
QCDAWXDDXYQEJJ-QYYRPYCUSA-N | |
Source | PubChem | |
URL | https://pubchem.ncbi.nlm.nih.gov | |
Description | Data deposited in or computed by PubChem | |
Canonical SMILES |
C1=NC(=C2C(=N1)N(C=N2)C3C(C(C(O3)CO)F)O)N | |
Source | PubChem | |
URL | https://pubchem.ncbi.nlm.nih.gov | |
Description | Data deposited in or computed by PubChem | |
Isomeric SMILES |
C1=NC(=C2C(=N1)N(C=N2)[C@H]3[C@@H]([C@@H]([C@H](O3)CO)F)O)N | |
Source | PubChem | |
URL | https://pubchem.ncbi.nlm.nih.gov | |
Description | Data deposited in or computed by PubChem | |
Molecular Formula |
C10H12FN5O3 | |
Source | PubChem | |
URL | https://pubchem.ncbi.nlm.nih.gov | |
Description | Data deposited in or computed by PubChem | |
DSSTOX Substance ID |
DTXSID70226033 | |
Record name | 3'-Fluoro-3'-deoxyadenosine | |
Source | EPA DSSTox | |
URL | https://comptox.epa.gov/dashboard/DTXSID70226033 | |
Description | DSSTox provides a high quality public chemistry resource for supporting improved predictive toxicology. | |
Molecular Weight |
269.23 g/mol | |
Source | PubChem | |
URL | https://pubchem.ncbi.nlm.nih.gov | |
Description | Data deposited in or computed by PubChem | |
CAS No. |
75059-22-2 | |
Record name | 3'-Fluoro-3'-deoxyadenosine | |
Source | ChemIDplus | |
URL | https://pubchem.ncbi.nlm.nih.gov/substance/?source=chemidplus&sourceid=0075059222 | |
Description | ChemIDplus is a free, web search system that provides access to the structure and nomenclature authority files used for the identification of chemical substances cited in National Library of Medicine (NLM) databases, including the TOXNET system. | |
Record name | 3'-Fluoro-3'-deoxyadenosine | |
Source | EPA DSSTox | |
URL | https://comptox.epa.gov/dashboard/DTXSID70226033 | |
Description | DSSTox provides a high quality public chemistry resource for supporting improved predictive toxicology. | |
Retrosynthesis Analysis
AI-Powered Synthesis Planning: Our tool employs the Template_relevance Pistachio, Template_relevance Bkms_metabolic, Template_relevance Pistachio_ringbreaker, Template_relevance Reaxys, Template_relevance Reaxys_biocatalysis model, leveraging a vast database of chemical reactions to predict feasible synthetic routes.
One-Step Synthesis Focus: Specifically designed for one-step synthesis, it provides concise and direct routes for your target compounds, streamlining the synthesis process.
Accurate Predictions: Utilizing the extensive PISTACHIO, BKMS_METABOLIC, PISTACHIO_RINGBREAKER, REAXYS, REAXYS_BIOCATALYSIS database, our tool offers high-accuracy predictions, reflecting the latest in chemical research and data.
Strategy Settings
Precursor scoring | Relevance Heuristic |
---|---|
Min. plausibility | 0.01 |
Model | Template_relevance |
Template Set | Pistachio/Bkms_metabolic/Pistachio_ringbreaker/Reaxys/Reaxys_biocatalysis |
Top-N result to add to graph | 6 |
Feasible Synthetic Routes
Disclaimer and Information on In-Vitro Research Products
Please be aware that all articles and product information presented on BenchChem are intended solely for informational purposes. The products available for purchase on BenchChem are specifically designed for in-vitro studies, which are conducted outside of living organisms. In-vitro studies, derived from the Latin term "in glass," involve experiments performed in controlled laboratory settings using cells or tissues. It is important to note that these products are not categorized as medicines or drugs, and they have not received approval from the FDA for the prevention, treatment, or cure of any medical condition, ailment, or disease. We must emphasize that any form of bodily introduction of these products into humans or animals is strictly prohibited by law. It is essential to adhere to these guidelines to ensure compliance with legal and ethical standards in research and experimentation.