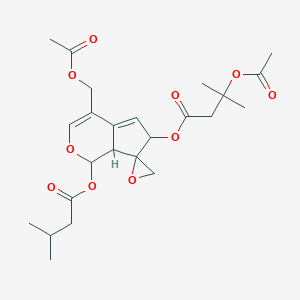
Acevaltrate
Overview
Description
Preparation Methods
Synthetic Routes and Reaction Conditions
Acevaltrate can be synthesized through the esterification of valtrate, another iridoid compound. The reaction involves the use of acetic anhydride and pyridine as reagents . The reaction is typically carried out at room temperature and requires purification through column chromatography to obtain the pure product .
Industrial Production Methods
Industrial production of this compound involves the extraction of the compound from the roots of Valeriana glechomifolia. The extraction process includes drying and grinding the plant material, followed by solvent extraction using ethanol or methanol . The extract is then subjected to various purification steps, including liquid-liquid extraction and chromatography, to isolate this compound .
Chemical Reactions Analysis
Types of Reactions
Acevaltrate undergoes several types of chemical reactions, including:
Oxidation: This compound can be oxidized to form various degradation products.
Reduction: The compound can be reduced under specific conditions to yield different iridoid derivatives.
Substitution: This compound can undergo substitution reactions, particularly esterification and transesterification.
Common Reagents and Conditions
Oxidation: Common oxidizing agents include hydrogen peroxide and potassium permanganate.
Reduction: Reducing agents such as sodium borohydride and lithium aluminum hydride are used.
Substitution: Esterification reactions typically involve acetic anhydride and pyridine.
Major Products Formed
The major products formed from these reactions include various oxidized and reduced derivatives of this compound, as well as esterified products .
Scientific Research Applications
Anti-Cancer Activity
Mechanism of Action:
Acevaltrate has demonstrated significant anti-cancer properties, particularly against multiple myeloma (MM). Research indicates that this compound inhibits the Otub1/c-Maf axis, which is crucial for myeloma cell survival. By disrupting the interaction between Otub1 and c-Maf, this compound promotes c-Maf polyubiquitination and degradation, leading to decreased transcriptional activity of genes essential for myeloma growth .
Case Studies:
- In vitro studies showed that this compound triggers apoptosis in MM cells and inhibits their proliferation. Notably, it was effective in reducing tumor growth in myeloma xenograft models without exhibiting significant toxicity .
- A study highlighted that this compound downregulated the expression of c-Maf target genes such as CCND2 and ITGB7, which are involved in cell cycle regulation and adhesion, respectively .
Neurological Applications
Calcium Channel Inhibition:
this compound exhibits dual inhibition of calcium channels (Ca v2.2 and Ca v3.1), which are implicated in various neurological disorders. The compound has shown an average inhibitory ratio of approximately 60.74% for Ca v2.2 and 36.34% for Ca v3.1, suggesting its potential use in treating conditions associated with calcium dysregulation .
Antioxidant Properties:
Research indicates that this compound possesses antioxidant capabilities, which may protect neuronal cells from oxidative stress—a contributing factor in neurodegenerative diseases .
Cardiovascular Benefits
Impact on Blood Pressure:
Preliminary studies suggest that compounds from the Valeriana genus, including this compound, may have a role in cardiovascular health by promoting vasodilation and potentially lowering blood pressure through their action on calcium channels .
Antimicrobial Properties
Inhibition of Pathogens:
this compound has been studied for its antimicrobial effects against various pathogens. Its efficacy as an antibacterial agent makes it a candidate for further exploration in developing natural antimicrobial therapies .
Phytochemical Research
Extraction and Analysis:
this compound is often isolated during phytochemical studies of Valeriana species. Research has focused on optimizing extraction methods to enhance yield and bioactivity of this compound alongside other valepotriates .
Data Summary Table
Mechanism of Action
Acevaltrate exerts its effects primarily by inhibiting Na+/K±ATPase activity . This inhibition disrupts ion transport across cell membranes, leading to various physiological effects. Additionally, this compound disrupts the interaction between Otub1 and c-Maf, leading to the polyubiquitination and degradation of c-Maf in proteasomes . This mechanism is particularly relevant in the context of its anti-myeloma activity .
Comparison with Similar Compounds
. These compounds share similar structures and biological activities but differ in their specific chemical modifications. Acevaltrate is unique in its potent inhibition of Na+/K±ATPase and its ability to disrupt the Otub1/c-Maf axis .
List of Similar Compounds
- Valtrate
- Didrovaltrate
- IVHD valtrate
This compound stands out due to its specific bioactivities and potential therapeutic applications, making it a compound of significant interest in various fields of research .
Biological Activity
Acevaltrate, an iridoid compound derived from Valeriana species, has garnered attention in recent years due to its diverse biological activities. This article explores the biological activity of this compound, focusing on its antioxidant properties, effects on cancer cells, and potential mechanisms of action, supported by relevant research findings and case studies.
1. Antioxidant Activity
Research has demonstrated that this compound exhibits significant antioxidant activity. A study conducted by Wang et al. (2017) investigated the structure-antioxidant activity relationships of iridoid valepotriates, including this compound. The findings indicated that this compound can effectively scavenge free radicals and reduce reactive oxygen species (ROS) levels through several mechanisms:
- Hydroxyl Group Generation : this compound can generate hydroxyl groups via intramolecular transfer from an oxirane ring, contributing to its antioxidant profile.
- GABAergic Signaling Pathways : It interacts with GABAergic signaling pathways to mitigate oxidative stress in cells .
The study also highlighted that the stability of the oxirane nucleus is crucial for maintaining these antioxidant properties.
2. Anti-Cancer Properties
This compound has shown promising anti-cancer effects, particularly against various cancer cell lines. A notable study by Zhang et al. (2023) reported that this compound inhibits hypoxia-inducible factor 1-alpha (HIF-1α), a critical regulator in cancer progression:
- Mechanism of Action : this compound significantly decreased HIF-1α levels in cancer cells exposed to hypoxic conditions, leading to reduced cell proliferation and increased apoptosis.
- Cell Line Sensitivity : The compound exhibited selective cytotoxicity against malignant cells while sparing non-malignant cells, suggesting a potential therapeutic window for its use in cancer treatment .
Table 1: Summary of Biological Activities of this compound
4. Future Directions for Research
The promising biological activities of this compound warrant further investigation into its potential therapeutic applications:
- In Vivo Studies : Future research should focus on in vivo models to better understand the pharmacokinetics and therapeutic efficacy of this compound.
- Mechanistic Studies : Detailed mechanistic studies are needed to elucidate the pathways through which this compound exerts its effects, particularly in cancer biology and neuropharmacology.
Q & A
Basic Research Questions
Q. What analytical methods are recommended for quantifying Acevaltrate in plant extracts, and how are they validated?
Reverse-phase high-performance liquid chromatography (HPLC) with UV detection is the standard method, using validated protocols for peak identification and quantification. For example, studies on Valeriana jatamansi chemotypes utilized HPLC with C18 columns and gradient elution systems to separate this compound from co-occurring valepotriates like valtrate and baldrinal . Method validation includes linearity checks (R² > 0.99), recovery tests (85–115%), and precision assessments (RSD < 5%) to ensure reproducibility.
Q. What are the primary pharmacological mechanisms of this compound identified in preclinical studies?
this compound exhibits cytotoxicity via Na+/K+-ATPase inhibition (IC₅₀ = 22.8–42.3 μM in rat tissues) and apoptosis induction in cancer cells. In multiple myeloma (MM), it disrupts the Otub1/c-Maf interaction, leading to proteasomal degradation of c-Maf, a transcription factor critical for MM survival . Mechanistic studies employ siRNA knockdown, co-immunoprecipitation, and luciferase reporter assays to validate target engagement.
Q. Which in vitro models are commonly used to assess this compound’s cytotoxicity and therapeutic potential?
Human cancer cell lines such as GLC4 (small-cell lung cancer) and COLO320 (colorectal adenocarcinoma) are standard models, with IC₅₀ values ranging from 1–6 μM . Dose-response curves are generated using MTT or resazurin assays, with normalization to untreated controls. Parallel testing in non-cancerous cell lines (e.g., HEK293) ensures selectivity.
Q. How do extraction methodologies impact this compound yield from Valeriana species?
Ultrasonic-assisted extraction (UAE) with ethanol/water mixtures (70–80% v/v) optimizes yield, balancing solubility and compound stability. Soxhlet extraction risks thermal degradation, while supercritical CO₂ methods require polarity modifiers. Post-extraction, preparative TLC or semi-preparative HPLC isolates this compound from complex matrices .
Q. What statistical approaches are used to analyze variability in this compound content across chemotypes?
Multivariate analyses, including AMMI (Additive Main Effects and Multiplicative Interaction) and BLUP (Best Linear Unbiased Prediction), account for genotype-environment interactions. For example, chemotype stability studies on Valeriana jatamansi reported 43.5% phenotypic variation explained by genetic factors, with residual variation attributed to environmental stressors .
Advanced Research Questions
Q. How can researchers resolve contradictions in reported IC₅₀ values of this compound across cancer cell lines?
Discrepancies may arise from assay conditions (e.g., serum concentration, incubation time) or cell line heterogeneity. Standardized protocols (e.g., 48-hour exposure in serum-free media) and cross-laboratory validation are critical. Meta-analyses using mixed-effects models can quantify variability sources, while RNA-seq profiling identifies resistance markers like ATP-binding cassette transporters .
Q. What experimental designs are optimal for studying this compound’s effects on protein-protein interactions like Otub1/c-Maf?
Structure-activity relationship (SAR) studies combined with molecular docking (e.g., AutoDock Vina) identify binding interfaces. Surface plasmon resonance (SPR) quantifies binding affinity (KD), while Förster resonance energy transfer (FRET) assays monitor real-time interaction dynamics in MM cells . CRISPR-Cas9 knockout models confirm pathway specificity.
Q. What multi-omics strategies are recommended to explore this compound’s systemic effects?
Integrate transcriptomics (RNA-seq), proteomics (LC-MS/MS), and metabolomics (NMR or UHPLC-QTOF) to map signaling networks. For example, network pharmacology analyses linked this compound’s anti-MM effects to downregulation of PI3K/AKT and NF-κB pathways . Pathway enrichment tools (e.g., DAVID, Metascape) prioritize targets, while machine learning (e.g., Random Forest) predicts off-target effects.
Q. How do environmental factors versus genetic factors influence this compound biosynthesis in Valeriana jatamansi?
Multi-environment trials (METs) with genotype × environment (G×E) interaction models reveal that 60–70% of this compound variation is genetic, while drought and soil pH modulate secondary metabolite production. Chemometric tools like PCA (Principal Component Analysis) and MTSI (Multi-Trait Stability Index) identify stable high-yielding chemotypes (e.g., A/B/31, U/B/1) for cultivation .
Q. What in silico approaches are validated for predicting this compound’s molecular targets?
Pharmacophore modeling (e.g., PharmaGist) and reverse docking (e.g., DRAR-CPI) screen against human proteome databases. Molecular dynamics simulations (e.g., GROMACS) assess binding stability, while systems biology platforms (e.g., Cytoscape) reconstruct interaction networks. Experimental validation via kinase profiling (e.g., KINOMEscan) confirms predicted targets .
Properties
IUPAC Name |
[4-(acetyloxymethyl)-1-(3-methylbutanoyloxy)spiro[6,7a-dihydro-1H-cyclopenta[c]pyran-7,2'-oxirane]-6-yl] 3-acetyloxy-3-methylbutanoate | |
---|---|---|
Details | Computed by LexiChem 2.6.6 (PubChem release 2019.06.18) | |
Source | PubChem | |
URL | https://pubchem.ncbi.nlm.nih.gov | |
Description | Data deposited in or computed by PubChem | |
InChI |
InChI=1S/C24H32O10/c1-13(2)7-19(27)33-22-21-17(16(11-30-22)10-29-14(3)25)8-18(24(21)12-31-24)32-20(28)9-23(5,6)34-15(4)26/h8,11,13,18,21-22H,7,9-10,12H2,1-6H3 | |
Details | Computed by InChI 1.0.5 (PubChem release 2019.06.18) | |
Source | PubChem | |
URL | https://pubchem.ncbi.nlm.nih.gov | |
Description | Data deposited in or computed by PubChem | |
InChI Key |
FWKBQAVMKVZEOT-UHFFFAOYSA-N | |
Details | Computed by InChI 1.0.5 (PubChem release 2019.06.18) | |
Source | PubChem | |
URL | https://pubchem.ncbi.nlm.nih.gov | |
Description | Data deposited in or computed by PubChem | |
Canonical SMILES |
CC(C)CC(=O)OC1C2C(=CC(C23CO3)OC(=O)CC(C)(C)OC(=O)C)C(=CO1)COC(=O)C | |
Details | Computed by OEChem 2.1.5 (PubChem release 2019.06.18) | |
Source | PubChem | |
URL | https://pubchem.ncbi.nlm.nih.gov | |
Description | Data deposited in or computed by PubChem | |
Molecular Formula |
C24H32O10 | |
Details | Computed by PubChem 2.1 (PubChem release 2019.06.18) | |
Source | PubChem | |
URL | https://pubchem.ncbi.nlm.nih.gov | |
Description | Data deposited in or computed by PubChem | |
DSSTOX Substance ID |
DTXSID30865184 | |
Record name | 4-[(Acetyloxy)methyl]-1-[(3-methylbutanoyl)oxy]-6,7a-dihydro-1H-spiro[cyclopenta[c]pyran-7,2'-oxiran]-6-yl 3-(acetyloxy)-3-methylbutanoate | |
Source | EPA DSSTox | |
URL | https://comptox.epa.gov/dashboard/DTXSID30865184 | |
Description | DSSTox provides a high quality public chemistry resource for supporting improved predictive toxicology. | |
Molecular Weight |
480.5 g/mol | |
Details | Computed by PubChem 2.1 (PubChem release 2021.05.07) | |
Source | PubChem | |
URL | https://pubchem.ncbi.nlm.nih.gov | |
Description | Data deposited in or computed by PubChem | |
Physical Description |
Solid | |
Record name | Acevaltrate | |
Source | Human Metabolome Database (HMDB) | |
URL | http://www.hmdb.ca/metabolites/HMDB0034494 | |
Description | The Human Metabolome Database (HMDB) is a freely available electronic database containing detailed information about small molecule metabolites found in the human body. | |
Explanation | HMDB is offered to the public as a freely available resource. Use and re-distribution of the data, in whole or in part, for commercial purposes requires explicit permission of the authors and explicit acknowledgment of the source material (HMDB) and the original publication (see the HMDB citing page). We ask that users who download significant portions of the database cite the HMDB paper in any resulting publications. | |
CAS No. |
25161-41-5 | |
Record name | Acevaltrate | |
Source | Human Metabolome Database (HMDB) | |
URL | http://www.hmdb.ca/metabolites/HMDB0034494 | |
Description | The Human Metabolome Database (HMDB) is a freely available electronic database containing detailed information about small molecule metabolites found in the human body. | |
Explanation | HMDB is offered to the public as a freely available resource. Use and re-distribution of the data, in whole or in part, for commercial purposes requires explicit permission of the authors and explicit acknowledgment of the source material (HMDB) and the original publication (see the HMDB citing page). We ask that users who download significant portions of the database cite the HMDB paper in any resulting publications. | |
Melting Point |
83 - 84 °C | |
Record name | Acevaltrate | |
Source | Human Metabolome Database (HMDB) | |
URL | http://www.hmdb.ca/metabolites/HMDB0034494 | |
Description | The Human Metabolome Database (HMDB) is a freely available electronic database containing detailed information about small molecule metabolites found in the human body. | |
Explanation | HMDB is offered to the public as a freely available resource. Use and re-distribution of the data, in whole or in part, for commercial purposes requires explicit permission of the authors and explicit acknowledgment of the source material (HMDB) and the original publication (see the HMDB citing page). We ask that users who download significant portions of the database cite the HMDB paper in any resulting publications. | |
Retrosynthesis Analysis
AI-Powered Synthesis Planning: Our tool employs the Template_relevance Pistachio, Template_relevance Bkms_metabolic, Template_relevance Pistachio_ringbreaker, Template_relevance Reaxys, Template_relevance Reaxys_biocatalysis model, leveraging a vast database of chemical reactions to predict feasible synthetic routes.
One-Step Synthesis Focus: Specifically designed for one-step synthesis, it provides concise and direct routes for your target compounds, streamlining the synthesis process.
Accurate Predictions: Utilizing the extensive PISTACHIO, BKMS_METABOLIC, PISTACHIO_RINGBREAKER, REAXYS, REAXYS_BIOCATALYSIS database, our tool offers high-accuracy predictions, reflecting the latest in chemical research and data.
Strategy Settings
Precursor scoring | Relevance Heuristic |
---|---|
Min. plausibility | 0.01 |
Model | Template_relevance |
Template Set | Pistachio/Bkms_metabolic/Pistachio_ringbreaker/Reaxys/Reaxys_biocatalysis |
Top-N result to add to graph | 6 |
Feasible Synthetic Routes
Disclaimer and Information on In-Vitro Research Products
Please be aware that all articles and product information presented on BenchChem are intended solely for informational purposes. The products available for purchase on BenchChem are specifically designed for in-vitro studies, which are conducted outside of living organisms. In-vitro studies, derived from the Latin term "in glass," involve experiments performed in controlled laboratory settings using cells or tissues. It is important to note that these products are not categorized as medicines or drugs, and they have not received approval from the FDA for the prevention, treatment, or cure of any medical condition, ailment, or disease. We must emphasize that any form of bodily introduction of these products into humans or animals is strictly prohibited by law. It is essential to adhere to these guidelines to ensure compliance with legal and ethical standards in research and experimentation.