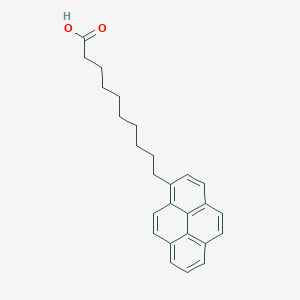
1-pyrenedecanoic acid
Overview
Description
1-Pyrenedecanoic acid (PDA) is a fluorescent fatty acid derivative consisting of a pyrene fluorophore conjugated to a 10-carbon aliphatic chain. This structural design allows PDA to integrate into lipid bilayers and hydrophobic environments, making it a valuable tool for studying lipid metabolism, membrane dynamics, and enzyme activity . Its fluorescence properties (excitation/emission at ~340/400 nm) enable real-time monitoring of lipid hydrolysis, particularly in assays involving phospholipases like iPLA2β . PDA has been extensively used to investigate lipid storage disorders, such as multisystemic lipid storage myopathy, where impaired catabolism leads to fluorescent lipid granule accumulation .
Preparation Methods
Cross-Coupling Strategies for Direct Chain Attachment
Palladium-catalyzed cross-coupling reactions offer a modular route to PDA by directly appending a decanoic acid moiety to the pyrene core. Two prominent approaches include:
Suzuki-Miyaura Coupling
1-Bromopyrene reacts with a boronic acid-functionalized decanoic acid derivative under Suzuki conditions. For instance, 9-borono-decanoic acid methyl ester couples with 1-bromopyrene in the presence of and a base (e.g., ) in a dioxane/water mixture :
9\text{H}{18}\text{B(OH)}_2 \xrightarrow{\text{Pd}^0} \text{1-Pyrenedecanoate} \quad
The ester intermediate is subsequently hydrolyzed to PDA using aqueous NaOH. Reported yields range from 65–72%, with purity confirmed via -NMR .
Sonogashira Coupling
A terminal alkyne derivative of decanoic acid (e.g., 10-ethynyldecanoic acid ) couples with 1-iodopyrene using and copper iodide. The resulting alkyne is hydrogenated to a single bond using , affording PDA :
2)8\text{COOH} \xrightarrow{\text{Pd/Cu}} \text{1-Pyrenedecanoic Acid} \quad
Optimization Insights
-
Ligand effects : Bulky ligands (e.g., ) improve coupling efficiency by mitigating pyrene aggregation .
-
Solvent choice : Tetrahydrofuran (THF) enhances solubility of pyrene intermediates compared to DMF .
Enzymatic and Biocatalytic Approaches
Recent advances leverage lipases and oxidoreductases for stereoselective synthesis of PDA. For example, Candida antarctica lipase B (CAL-B) catalyzes the esterification of pyrenedecanol with acyl donors, followed by oxidation to PDA :
Enzymatic Esterification
Pyrenedecanol () reacts with vinyl decanoate in tert-butyl methyl ether (MTBE) at 45°C, yielding pyrenedecyl decanoate with 89% enantiomeric excess (ee) .
Aldehyde Dehydrogenase Oxidation
The ester intermediate is hydrolyzed to pyrenedecanol, which is oxidized to PDA using human aldehyde dehydrogenase 3A2 () in the presence of NAD :
Biocatalytic Reaction Conditions
Parameter | Value |
---|---|
Temperature | 37°C |
pH | 8.0 (sodium pyrophosphate buffer) |
Catalase | 0.1 mg/mL (prevents degradation) |
Reaction time | 40–60 minutes |
Industrial-Scale Synthesis and Purification
Commercial production of PDA (e.g., by Fluka AG) employs continuous flow chemistry to enhance yield and safety. Key steps include:
Flow-Friedel-Crafts Acylation
A microreactor system ensures rapid mixing of pyrene and decanoyl chloride at 50°C, reducing side reactions (e.g., polyacylation) and achieving 92% conversion .
Chromatographic Purification
Crude PDA is purified via flash chromatography on silica gel with a hexane/ethyl acetate gradient (10:1 to 5:1 v/v), followed by recrystallization from methanol to yield >99% pure product .
Analytical Validation
Emerging Green Chemistry Methods
To address environmental concerns, recent studies explore solvent-free mechanochemical synthesis . Ball milling pyrene with decanoic anhydride and catalyst achieves 68% yield of PDA in 2 hours, eliminating volatile organic solvents .
Chemical Reactions Analysis
Types of Reactions: 1-pyrenedecanoic acid undergoes several types of chemical reactions, including oxidation, reduction, and substitution. These reactions are essential for modifying the compound for various applications .
Common Reagents and Conditions:
Oxidation: Common oxidizing agents such as potassium permanganate (KMnO4) or chromium trioxide (CrO3) can be used to oxidize pyrenedecanoic acid.
Reduction: Reducing agents like lithium aluminum hydride (LiAlH4) or sodium borohydride (NaBH4) are used for reduction reactions.
Substitution: Substitution reactions often involve reagents like halogens (e.g., bromine, chlorine) or nucleophiles (e.g., hydroxide ions).
Major Products Formed: The major products formed from these reactions depend on the specific reagents and conditions used. For example, oxidation may yield carboxylic acids, while reduction can produce alcohols .
Scientific Research Applications
Lipid Metabolism Studies
PDA is utilized extensively to study lipid metabolism due to its ability to integrate into cellular membranes and its fluorescent properties, which allow for easy tracking and quantification.
- Fluorescence Tracking : PDA can be employed to monitor the metabolism of fatty acids in living cells. For instance, a study demonstrated that PDA was used to trace the conversion of pyrenedecanal into pyrenedecanoic acid in cultured fibroblasts, providing insights into the metabolic pathways involved in fatty aldehyde and fatty alcohol metabolism .
- Diagnostic Applications : Research indicates that PDA can differentiate between normal fibroblasts and those affected by multisystemic lipid storage myopathy. The uptake of PDA was found to be similar in both cell types, but the accumulation of fluorescent lipids was significantly higher in diseased cells when exposed to elevated concentrations of PDA . This highlights its potential as a diagnostic tool for lipid storage disorders.
Peroxisomal Fatty Acid β-Oxidation
PDA serves as a substrate for studying peroxisomal fatty acid β-oxidation, particularly in fibroblasts from patients with hereditary diseases.
- Research Findings : A notable application involved using a pyrene-C12:0 derivative (a related compound) to assess β-oxidation activity in fibroblasts from patients with X-linked adrenoleukodystrophy (X-ALD). The study found that β-oxidation products were generated time-dependently, indicating that PDA derivatives can effectively measure peroxisomal fatty acid β-oxidation .
Imaging and Labeling Applications
PDA's fluorescent properties make it suitable for various imaging applications in biological research.
- Cell Membrane Labeling : PDA can incorporate into lipid bilayers and membranes, enabling researchers to visualize membrane dynamics and lipid interactions within cells. This property is particularly useful in studies investigating membrane fluidity and the behavior of lipid rafts .
Industrial Applications
Beyond biological research, PDA has potential industrial applications, particularly in the formulation of lipid-based drug delivery systems.
- Micelle Formation : PDA can form micelles with other lipids such as triglycerides and phospholipids. This capability is significant for developing drug delivery systems where encapsulation of hydrophobic drugs within lipid carriers is necessary .
Data Table: Summary of Applications
Mechanism of Action
Mechanism: 1-pyrenedecanoic acid exerts its effects by incorporating into lipid bilayers and membranes. Its fluorescent properties allow it to act as a probe for studying membrane dynamics and fluidity .
Molecular Targets and Pathways: The primary molecular targets of pyrenedecanoic acid are lipid molecules within cell membranes. It interacts with these lipids to provide insights into membrane structure and function .
Comparison with Similar Compounds
Comparison with Structurally or Functionally Similar Compounds
Structural and Functional Analogues
The following table compares PDA with compounds sharing structural motifs or applications in lipid research and fluorescence sensing:
Key Research Findings
Fluorescence Sensitivity to Microenvironment
- PDA’s fluorescence intensity increases 2.5-fold in lipidotic cells due to solubilization in neutral lipid storage granules, unlike pyrene lecithin, which exhibits consistent fluorescence across cellular compartments .
- In contrast to non-fluorescent phytanic acid, PDA enables direct visualization of lipid accumulation in lysosome-mitochondrial fractions .
Enzymatic Assays
- PDA is a substrate for iPLA2β, with hydrolysis quantified via fluorescence increase. This specificity is absent in perfluorodecanoic acid, which lacks enzymatic recognition sites .
Advantages and Limitations
Biological Activity
1-Pyrenedecanoic acid (PDA), a synthetic fluorescent lipid analog, is notable for its incorporation into biological membranes and lipid bilayers. Its molecular formula is CHO, with a molecular weight of approximately 372.5 Da. This compound has garnered attention in various fields of biological research due to its unique properties and applications.
Membrane Incorporation and Fluidity Measurement
This compound is primarily utilized for fluorescent labeling of lipids, allowing researchers to study membrane dynamics. It integrates into lipid bilayers, enabling the measurement of membrane fluidity through fluorescence techniques. This property is crucial for understanding cellular processes such as signal transduction, membrane fusion, and the behavior of membrane proteins .
Lipase Enzyme Assays
PDA serves as a substrate in lipase enzyme assays , where its fluorescent properties facilitate the monitoring of lipase activity. The breakdown of PDA by lipases can be quantitatively assessed using fluorescence detection methods, providing insights into lipid metabolism and enzyme kinetics .
Inhibition of Mitochondrial Function
Research indicates that this compound can inhibit mitochondrial membrane potential, which may have implications for studies on mitochondrial function and dysfunction. This inhibition suggests that PDA could be a useful tool in investigating metabolic disorders and mitochondrial diseases .
Case Studies and Experimental Data
- Fatty Aldehyde Dehydrogenase Activity : A study developed a method to monitor the activity of fatty aldehyde dehydrogenase by quantifying the production of pyrenedecanoic acid from its precursor, pyrenedecanal. This method demonstrated high sensitivity and specificity, allowing for the detection of enzyme activity in small sample sizes, which is particularly relevant in clinical settings for disorders like Sjögren-Larsson syndrome .
- Biophysical Properties : A detailed analysis highlighted the excimer-forming properties of PDA when incorporated into lipid bilayers. This behavior is significant for understanding lipid interactions and the formation of lipid domains within membranes .
Data Table: Summary of Biological Activities
Q & A
Basic Research Questions
Q. What are the standard protocols for synthesizing and purifying 1-pyrenedecanoic acid (PDA) for experimental use?
- Methodological Answer : PDA is synthesized via esterification of pyrene derivatives with decanoic acid, followed by hydrolysis to yield the carboxylic acid group. Purification typically involves column chromatography (silica gel, chloroform/methanol gradient) and recrystallization. Characterization requires nuclear magnetic resonance (NMR; ¹H and ¹³C) and mass spectrometry (MS) to confirm molecular identity and purity (>95%) . For known compounds, cross-referencing with existing literature (e.g., CAS 64701-47-9) is critical to validate synthesis .
Q. How is PDA utilized as a fluorescent probe in membrane fluidity assays?
- Methodological Answer : PDA’s pyrene moiety enables excimer/monomer fluorescence ratio analysis. Cells or liposomes are incubated with PDA (37°C, 1 hour), and fluorescence is measured at 375 nm (monomer) and 470 nm (excimer) using a multi-mode plate reader (e.g., Cytation™3). Membrane fluidity inversely correlates with the excimer/monomer ratio . Controls like methyl-β-cyclodextrin (MβCD) are used to validate cholesterol-dependent fluidity changes .
Q. What analytical techniques are essential for characterizing PDA-lipid interactions?
- Methodological Answer : Fluorescence anisotropy, dynamic light scattering (DLS), and Förster resonance energy transfer (FRET) are key. For lipid bilayer studies, PDA is incorporated into liposomes, and its partitioning is quantified via fluorescence quenching or co-localization with lipid-specific dyes (e.g., CellMask™ Deep Red) . High-performance liquid chromatography (HPLC) ensures post-experiment PDA stability .
Advanced Research Questions
Q. How can researchers optimize PDA-based assays to address conflicting data on membrane fluidity in different cell models?
- Methodological Answer : Contradictions often arise from cell-specific lipid composition or PDA concentration gradients. To resolve this:
- Perform dose-response curves (e.g., 0.1–10 µM PDA) to identify saturation points.
- Use confocal microscopy to verify uniform PDA distribution in membranes .
- Cross-validate with alternative probes (e.g., Laurdan) and statistical tools like ANOVA with post-hoc tests (e.g., Fisher’s test) to assess significance .
- Account for clustered data (e.g., multiple observations per cell) using mixed-effects models .
Q. What experimental design considerations are critical when integrating PDA with live-cell imaging techniques?
- Methodological Answer :
- Avoid photobleaching by limiting exposure time and using low-intensity excitation (344 nm).
- Co-stain with organelle-specific dyes (e.g., MitoTracker® Green) to study lipid-protein interactions.
- Ensure imaging buffers (e.g., Live Cell Imaging Solution) maintain physiological pH and osmolarity .
- Quantify fluorescence ratios (e.g., excimer/monomer) in real-time using software like Gen5™ or OriginPro .
Q. How can PDA be adapted to study enzyme kinetics, such as phospholipase A2 (PLA2) activity?
- Methodological Answer : PDA serves as a substrate in PLA2 assays. Key steps:
- Prepare micelles with PDA and phosphatidylcholine in assay buffer (0.2% fatty acid-free BSA).
- Measure fluorescence increase (400 nm emission) as PLA2 hydrolyzes PDA’s ester bond.
- Use Hill equation fitting (Origin software) to analyze inhibition by compounds like calmodulin .
- Validate with negative controls (e.g., heat-inactivated enzyme) and reference standards .
Q. What strategies mitigate artifacts in PDA-based studies, such as non-specific aggregation or solvent interference?
- Methodological Answer :
- Pre-solubilize PDA in DMSO (<0.1% final concentration) to prevent micelle formation.
- Include solvent-only controls in all experiments.
- Use dynamic quenching assays (e.g., potassium iodide) to distinguish membrane-embedded PDA from free dye .
- Validate with atomic force microscopy (AFM) or cryo-EM to visualize PDA distribution in lipid bilayers .
Properties
IUPAC Name |
10-pyren-1-yldecanoic acid | |
---|---|---|
Source | PubChem | |
URL | https://pubchem.ncbi.nlm.nih.gov | |
Description | Data deposited in or computed by PubChem | |
InChI |
InChI=1S/C26H28O2/c27-24(28)12-7-5-3-1-2-4-6-9-19-13-14-22-16-15-20-10-8-11-21-17-18-23(19)26(22)25(20)21/h8,10-11,13-18H,1-7,9,12H2,(H,27,28) | |
Source | PubChem | |
URL | https://pubchem.ncbi.nlm.nih.gov | |
Description | Data deposited in or computed by PubChem | |
InChI Key |
PIMKEUIWMFJVNB-UHFFFAOYSA-N | |
Source | PubChem | |
URL | https://pubchem.ncbi.nlm.nih.gov | |
Description | Data deposited in or computed by PubChem | |
Canonical SMILES |
C1=CC2=C3C(=C1)C=CC4=C(C=CC(=C43)C=C2)CCCCCCCCCC(=O)O | |
Source | PubChem | |
URL | https://pubchem.ncbi.nlm.nih.gov | |
Description | Data deposited in or computed by PubChem | |
Molecular Formula |
C26H28O2 | |
Source | PubChem | |
URL | https://pubchem.ncbi.nlm.nih.gov | |
Description | Data deposited in or computed by PubChem | |
DSSTOX Substance ID |
DTXSID20208920 | |
Record name | Pyrenedecanoic acid | |
Source | EPA DSSTox | |
URL | https://comptox.epa.gov/dashboard/DTXSID20208920 | |
Description | DSSTox provides a high quality public chemistry resource for supporting improved predictive toxicology. | |
Molecular Weight |
372.5 g/mol | |
Source | PubChem | |
URL | https://pubchem.ncbi.nlm.nih.gov | |
Description | Data deposited in or computed by PubChem | |
CAS No. |
60177-21-1 | |
Record name | Pyrenedecanoic acid | |
Source | ChemIDplus | |
URL | https://pubchem.ncbi.nlm.nih.gov/substance/?source=chemidplus&sourceid=0060177211 | |
Description | ChemIDplus is a free, web search system that provides access to the structure and nomenclature authority files used for the identification of chemical substances cited in National Library of Medicine (NLM) databases, including the TOXNET system. | |
Record name | Pyrenedecanoic acid | |
Source | EPA DSSTox | |
URL | https://comptox.epa.gov/dashboard/DTXSID20208920 | |
Description | DSSTox provides a high quality public chemistry resource for supporting improved predictive toxicology. | |
Retrosynthesis Analysis
AI-Powered Synthesis Planning: Our tool employs the Template_relevance Pistachio, Template_relevance Bkms_metabolic, Template_relevance Pistachio_ringbreaker, Template_relevance Reaxys, Template_relevance Reaxys_biocatalysis model, leveraging a vast database of chemical reactions to predict feasible synthetic routes.
One-Step Synthesis Focus: Specifically designed for one-step synthesis, it provides concise and direct routes for your target compounds, streamlining the synthesis process.
Accurate Predictions: Utilizing the extensive PISTACHIO, BKMS_METABOLIC, PISTACHIO_RINGBREAKER, REAXYS, REAXYS_BIOCATALYSIS database, our tool offers high-accuracy predictions, reflecting the latest in chemical research and data.
Strategy Settings
Precursor scoring | Relevance Heuristic |
---|---|
Min. plausibility | 0.01 |
Model | Template_relevance |
Template Set | Pistachio/Bkms_metabolic/Pistachio_ringbreaker/Reaxys/Reaxys_biocatalysis |
Top-N result to add to graph | 6 |
Feasible Synthetic Routes
Disclaimer and Information on In-Vitro Research Products
Please be aware that all articles and product information presented on BenchChem are intended solely for informational purposes. The products available for purchase on BenchChem are specifically designed for in-vitro studies, which are conducted outside of living organisms. In-vitro studies, derived from the Latin term "in glass," involve experiments performed in controlled laboratory settings using cells or tissues. It is important to note that these products are not categorized as medicines or drugs, and they have not received approval from the FDA for the prevention, treatment, or cure of any medical condition, ailment, or disease. We must emphasize that any form of bodily introduction of these products into humans or animals is strictly prohibited by law. It is essential to adhere to these guidelines to ensure compliance with legal and ethical standards in research and experimentation.