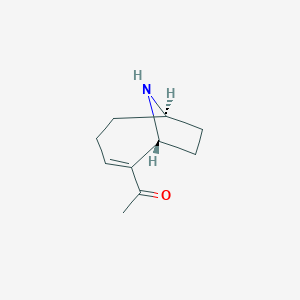
Anatoxin A
Overview
Description
Anatoxin A (C₁₀H₁₅NO), a bicyclic secondary amine alkaloid, is a potent neurotoxin produced by cyanobacteria such as Anabaena, Oscillatoria, and Phormidium . It acts as a full agonist of nicotinic acetylcholine receptors (nAChRs), causing overstimulation of neuromuscular junctions, leading to paralysis, respiratory arrest, and death in vertebrates . Its rigid bicyclic structure mimics acetylcholine but resists enzymatic degradation, resulting in prolonged receptor activation . This compound is detected via liquid chromatography-tandem mass spectrometry (LC-MS/MS) and quantified per cell or dry weight in environmental samples, with concentrations ranging from 3.0–179 µg g⁻¹ dw in benthic mats .
Preparation Methods
Synthetic Routes and Reaction Conditions: Anatoxin-a can be synthesized through various chemical routes. One common method involves the cyclization of a precursor compound, followed by a series of reduction and oxidation reactions to form the bicyclic structure characteristic of anatoxin-a . The reaction conditions typically involve the use of strong acids or bases, high temperatures, and specific catalysts to facilitate the cyclization and subsequent reactions.
Industrial Production Methods: Industrial production of anatoxin-a is primarily focused on its extraction from cyanobacterial cultures. The cyanobacteria are cultivated under controlled conditions to maximize the production of anatoxin-a. The compound is then extracted using solvent extraction methods, followed by purification through techniques such as liquid chromatography .
Chemical Reactions Analysis
Types of Reactions: Anatoxin-a undergoes various chemical reactions, including:
Oxidation: Anatoxin-a can be oxidized to form various derivatives, which may have different toxicological properties.
Reduction: Reduction reactions can convert anatoxin-a into less toxic forms.
Substitution: Substitution reactions can modify the functional groups on anatoxin-a, potentially altering its biological activity.
Common Reagents and Conditions: Common reagents used in these reactions include oxidizing agents like potassium permanganate, reducing agents like sodium borohydride, and various organic solvents. The reaction conditions often involve specific temperatures, pH levels, and catalysts to achieve the desired transformations .
Major Products Formed: The major products formed from these reactions include various anatoxin-a derivatives, such as homoanatoxin-a and dihydroanatoxin-a, which may have different levels of toxicity and biological activity .
Scientific Research Applications
Neurological Research
Anatoxin A serves primarily as a pharmacological probe in neurological studies due to its high affinity for nicotinic acetylcholine receptors (nAChRs). Research indicates that it can mimic acetylcholine and induce depolarizing neuromuscular blockade, making it valuable for studying conditions characterized by low acetylcholine levels such as:
- Myasthenia Gravis
- Alzheimer's Disease
- Parkinson's Disease
- Muscular Dystrophy
Studies have demonstrated that this compound can effectively activate nAChRs in vitro, facilitating investigations into receptor function and potential therapeutic interventions for these diseases .
Immunotoxicity Studies
Recent findings suggest that this compound also exhibits immunotoxic effects , affecting lymphocyte viability and functionality. In vitro studies on fish lymphocytes revealed that exposure to this compound led to:
- Increased reactive oxygen species (ROS) production
- Induction of apoptosis characterized by DNA fragmentation
- Altered expression of pro-inflammatory cytokines such as IL-1β and TNF-α
These findings underscore the potential of this compound as a model compound for studying immune responses and oxidative stress .
Toxicological Assessments
This compound is frequently utilized in toxicological research to assess the risks associated with cyanobacterial blooms. Its acute toxicity has been documented through various studies, including:
- Determining LD50 values across different animal models (e.g., mice, rats)
- Evaluating the neurotoxic effects leading to respiratory paralysis and death within minutes of exposure
Such data are critical for understanding the public health implications of cyanobacterial toxins in freshwater ecosystems .
Detection Methods
This compound is increasingly recognized for its role in environmental monitoring of harmful algal blooms (HABs). Recent advancements include:
- Development of analytical methods for detecting Anatoxin A and its congeners in water samples
- Implementation of monitoring programs aimed at assessing risks associated with cyanotoxins, particularly following incidents like the Toledo water crisis
These efforts are essential for safeguarding public health and informing water management strategies .
Ecotoxicological Impact Studies
Research on the ecological impacts of this compound has highlighted its effects on aquatic organisms. Studies have shown that exposure can lead to significant alterations in community structure and biodiversity within freshwater habitats. This underscores the importance of monitoring this compound levels in assessing ecosystem health .
Case Study 1: Neurotoxic Effects on Aquatic Species
A study conducted on Carassius auratus (goldfish) exposed to varying concentrations of this compound demonstrated significant neurotoxic effects, including:
- Increased rates of apoptosis in lymphocytes
- Morphological changes indicative of mitochondrial damage
These results provide insights into the broader implications of cyanobacterial toxins on aquatic ecosystems .
Case Study 2: Public Health Monitoring Post-Cyanobacterial Blooms
Following a cyanobacterial bloom incident in a major water supply, monitoring efforts revealed elevated levels of this compound. This prompted immediate public health advisories and led to the establishment of enhanced monitoring protocols across multiple states, highlighting the critical need for vigilance regarding cyanotoxins .
Mechanism of Action
Anatoxin-a exerts its effects by acting on nicotinic acetylcholine receptors (nAchR). It mimics the binding of acetylcholine, the natural ligand of these receptors, leading to sustained depolarization of the muscle endplate. This overstimulation results in muscle fatigue, paralysis, and ultimately respiratory failure . Anatoxin-a can cross biological membranes passively, allowing it to act rapidly on its molecular targets .
Comparison with Similar Compounds
Structural Analogues
Homoanatoxin-a
- Structure : A methylated derivative of anatoxin A, with an additional methyl group on the bicyclic backbone .
- Source : Co-produced with this compound in Phormidium autumnale and Oscillatoria spp. .
- Toxicity : Similar mechanism (nAChR agonist) but slightly reduced potency compared to this compound. LC-MS/MS analysis shows co-occurrence in environmental samples, with quotas varying up to 59-fold .
- Detection : Differentiated from this compound via retention time shifts in LC-MS .
Dihydroanatoxin-a and Dihydrohomoanatoxin-a
- Structure : Hydrogenated variants with saturated double bonds in the bicyclic ring .
- Source : Identified in Anabaena flosaquae and Phormidium mats .
- Toxicity : Reduced receptor affinity due to loss of structural rigidity. EC₅₀ values are 10–100× higher than this compound in vitro .
Functional Analogues
This compound(s)
- Structure : A phosphate ester with a guanidine moiety, unrelated to this compound .
- Source : Produced by Anabaena flos-aquae .
- Mechanism: Non-competitive acetylcholinesterase (AChE) inhibitor, causing acetylcholine accumulation .
- Toxicity : In vivo studies in rats show LD₅₀ of 20 µg/kg, compared to 200–250 µg/kg for this compound .
β-N-Methylamino-L-alanine (BMAA)
- Structure: A non-proteinogenic amino acid, distinct from this compound .
- Source: Produced by diverse cyanobacteria (e.g., Microcystis, Anabaena) .
- Mechanism : Misincorporation into proteins and glutamate receptor overactivation, linked to neurodegenerative diseases .
- Detection : Co-occurs with this compound in freshwater systems but requires distinct analytical methods (e.g., HPLC-fluorescence) .
Data Tables
Table 1. Structural and Functional Comparison of this compound and Analogues
Table 2. Environmental Variability of this compound and Analogues
Key Research Findings
Biosynthetic Pathways : this compound production is linked to polyketide synthase genes (osc, ana clusters) in Oscillatoria and Anabaena, while BMAA lacks a defined cluster .
Environmental Sensitivity : this compound quotas in Cylindrospermum vary 42–59× under shifting C/N ratios, whereas BMAA production is stable .
Toxicological Divergence : this compound’s acute neurotoxicity contrasts with BMAA’s chronic effects, necessitating distinct risk assessments .
Analytical Challenges : Co-occurrence of this compound and homoanatoxin-a in benthic mats complicates quantification without isotopic labeling .
Biological Activity
Anatoxin A (ANTX-A) is a potent neurotoxin produced by certain cyanobacteria, particularly species of the genera Anabaena and Aphanizomenon. This compound is known for its severe effects on the nervous system, leading to respiratory paralysis and death in various organisms, including humans. The biological activity of ANTX-A encompasses its mechanisms of action, toxic effects, and potential immunotoxicity, which are critical for understanding its environmental and health impacts.
This compound primarily functions as a postsynaptic depolarizing neuromuscular blocking agent. It mimics acetylcholine, binding to nicotinic acetylcholine receptors (nAChRs) and causing continuous stimulation of these receptors without being broken down by acetylcholinesterase. This results in prolonged muscle contraction followed by paralysis due to receptor desensitization. Studies have shown that ANTX-A acts on various nAChR subtypes, demonstrating its broad neuromuscular impact across different species, including amphibians and mammals .
Neurotoxicity
The neurotoxic effects of ANTX-A have been extensively documented. In laboratory studies, exposure to ANTX-A has resulted in:
- Respiratory Paralysis : This is a well-established consequence of ANTX-A toxicity, leading to death in animal models at doses significantly higher than those considered safe .
- Histopathological Changes : In fish lymphocytes (Carassius auratus), exposure to varying concentrations of ANTX-A resulted in apoptosis characterized by DNA fragmentation and mitochondrial damage. Flow cytometry revealed apoptotic rates of 18.89% to 39.23% at concentrations from 0.01 mg/L to 10 mg/L after 12 hours of exposure .
Concentration (mg/L) | Apoptotic Rate (%) |
---|---|
0.01 | 18.89 |
0.1 | 22.89 |
1 | 39.23 |
10 | 35.58 |
Immunotoxicity
Recent studies have highlighted the immunotoxic potential of ANTX-A, indicating that it can impair immune responses in aquatic organisms:
- Reactive Oxygen Species (ROS) : Exposure to ANTX-A significantly increased ROS levels and malondialdehyde (MDA) concentrations in lymphocytes, suggesting oxidative stress as a mechanism of toxicity .
- Antioxidant Enzyme Activity : The activities of key antioxidant enzymes such as superoxide dismutase (SOD), catalase (CAT), glutathione peroxidase (GPx), and glutathione reductase (GR) were markedly reduced following ANTX-A exposure, indicating compromised cellular defense mechanisms against oxidative damage .
Case Studies
Several case studies have documented the effects of ANTX-A on both wildlife and humans:
- Wildlife Intoxication : Instances of fish kills attributed to cyanobacterial blooms containing ANTX-A have been reported, showcasing its ecological impact.
- Human Exposure : Documented cases of human poisoning have included symptoms such as muscle cramps, respiratory failure, and neurological disturbances shortly after exposure .
Research Findings
Research continues to explore the full scope of ANTX-A's biological activity:
- A study indicated that the acute oral toxicity of ANTX-A is significantly high, with human equivalent doses leading to severe neurotoxic symptoms within minutes post-exposure .
- Further investigations are necessary to understand the long-term effects of chronic exposure and potential cumulative impacts on health and ecosystems.
Q & A
Basic Research Questions
Q. What methodological considerations are critical for detecting Anatoxin A in environmental water samples?
this compound detection requires rigorous validation of analytical techniques such as liquid chromatography-tandem mass spectrometry (LC-MS/MS) or enzyme-linked immunosorbent assays (ELISA). Key considerations include:
- Sample preservation : Immediate acidification (pH ≤3) to prevent degradation .
- Matrix interference : Use of solid-phase extraction (SPE) for purification, especially in eutrophic waters with high organic content .
- Calibration standards : Isotope-labeled internal standards (e.g., deuterated anatoxin-a) to correct for recovery variability .
- Limit of detection (LOD) : Ensure LOD ≤1 ng/L to meet regulatory thresholds for cyanotoxin monitoring .
Q. How can researchers resolve contradictory data on this compound toxicity thresholds across different experimental models?
Discrepancies often arise from variations in:
- Biological models : Differences in acetylcholine receptor subtypes (e.g., rodent vs. zebrafish models) .
- Exposure routes : Intraperitoneal injection vs. oral administration alters bioavailability .
- Data normalization : Use of relative potency factors (RPFs) to standardize toxicity metrics across studies . Cross-validation with in vitro receptor-binding assays (e.g., nicotinic acetylcholine receptor inhibition) is recommended to harmonize findings .
Q. What experimental designs are optimal for studying this compound production under varying environmental conditions?
Controlled mesocosm experiments should include:
- Nutrient gradients : Nitrogen-to-phosphorus (N:P) ratios (e.g., 10:1 to 50:1) to simulate eutrophic conditions .
- Light cycles : 12h light/dark periods with PAR (photosynthetically active radiation) intensity ≥100 µmol photons/m²/s .
- Temperature stratification : Gradients from 15°C to 30°C to mimic seasonal blooms . Quantify toxin production via qPCR targeting the anaC gene (biosynthetic cluster) alongside LC-MS/MS .
Advanced Research Questions
Q. What genomic and metagenomic approaches identify this compound biosynthetic gene clusters (BGCs) in uncultured cyanobacteria?
- Whole-genome sequencing : Use PacBio HiFi or Oxford Nanopore for long-read assembly of Microcoleus spp. genomes .
- BGC prediction : Tools like antiSMASH or PRISM to annotate nonribosomal peptide synthetase (NRPS) and polyketide synthase (PKS) domains .
- Phylogenetic analysis : Compare ana gene clusters with homologs in Cylindrospermum stagnale (e.g., 85% sequence homology) to infer horizontal gene transfer .
Q. How can isotopic labeling elucidate this compound biosynthetic pathways in cyanobacteria?
- Stable isotope probing (SIP) : Feed Cylindrospermum cultures with [U-¹³C]-proline to track incorporation into the toxin’s bicyclic structure .
- High-resolution MS/MS : Fragment labeled anatoxin-a to map isotopic enrichment in specific carbon positions (e.g., C-2 and C-9) .
- Kinetic modeling : Calculate flux rates through proline dehydrogenase and Δ¹-pyrroline-5-carboxylate pathways .
Q. What computational strategies validate this compound’s interaction with nicotinic acetylcholine receptors?
- Molecular docking : Use AutoDock Vina to simulate binding to α4β2 receptor subtypes, focusing on hydrogen bonding with Asp-195 and cation-π interactions .
- Molecular dynamics (MD) simulations : Analyze stability of toxin-receptor complexes over 100-ns trajectories (e.g., RMSD <2 Å) .
- Free energy calculations : MM-PBSA/GBSA to quantify binding affinities (ΔG <−40 kcal/mol indicates strong inhibition) .
Properties
Key on ui mechanism of action |
Anatoxin-a is a nicotinic (cholinergic) agonist that binds to neuronal nicotinic acetylcholine receptors. It has been suggested that the activation of presynaptic nicotinic acetylcholine receptors by anatoxin-a results in an influx of Na+, producing sufficient local depolarization to open voltage sensitive Ca++ and Na+ channels. The latter may then amplify the response, activating further Ca++ channels. As a result of this depolarization there is a block of further electrical transmission, and at sufficiently high doses this can lead to paralysis, asphyxiation and death. Anatoxin-a is more potent than nicotine or acetylcholine in evoking type 1A or type 2 current responses in rat hippocampal neurones, and it is more potent than nicotine in its ability to evoke the secretion of endogenous catecholamines from bovine adrenal chromaffin cells through their neuronal-type nicotinic receptors. Similar to nicotine, anatoxin-a was more potent than noradrenaline in releasing dopamine from striatal nerve terminals from rat superfused hippocampal synaptosomes. In vivo studies in the rat showed that the toxin stimulates the sympathetic system through the release of catecholamines from nerve endings. Anatoxin-a is a nicotinic (cholinergic) agonist that binds to neuronal nicotinic acetylcholine receptors. It has been suggested that the activation of presynaptic nicotinic acetylcholine receptors by anatoxin-a results in an influx of Na+, producing sufficient local depolarisation to open voltage sensitive Ca++ and Na+ channels. The latter may then amplify the response, activating further Ca++ channels. As a result of this depolarization there is a block of further electrical transmission, and at sufficiently high doses this can lead to paralysis, asphyxiation and death. Anatoxin-a (AnTx) is a natural neurotoxin, which acts as a potent and stereoselective agonist at the nicotinic acetylcholine receptors. ... The aim of this study was to determine the neurochemical bases for AnTx-induced striatal DA release, using the brain microdialysis technique, in freely moving rats. Local application of AnTx (3.5 mM) through the microdialysis probe produced an increase in striatal DA levels (701 +/- 51% with respect to basal values). The effect of infusion of AnTx in Ca(2+)-free Ringer medium, in Na(+)-free Ringer medium and with TTX in the medium, was inhibited. Also, reserpine pre-treatment blocked the action of AnTx on striatal DA levels. To investigate the involvement of the DA transporter, the effects of AnTx were observed in the presence of nomifensine. The coadministration of AnTx and nomifensine evoked an additive effect on striatal DA levels. The latter results show that the DA release is not mediated by a decreased DA uptake. Taken as a whole, these results suggest that the effects of AnTx are predominantly mediated by an exocytotic mechanism, Ca(2+)-, Na(+)- and TTX-dependent, and not by a mechanism mediated by the DA transporter. (+)-Anatoxin-a (ANTX) stimulated guinea pig ileum contraction with a potency similar to that of acetylcholine (ACh); the stimulation was blocked by tubocurarine, hexamethonium, or atropine. Although the contraction stimulated by ANTX was blocked by atropine, no specific inhibition of the binding of [3H]N-methylscopolamine to ileum membranes was observed in the presence of ANTX. Furthermore, ANTX failed to stimulate the secretion of alpha-amylase from pancreatic acinar cells, a process that is activated by cholinergic agonists at the muscarinic receptors. When the ileum itself was stimulated by ACh, the contraction was not blocked by either hexamethonium or tubocurarine. Preincubation of the ileum with hemicholinium caused a 50% reduction in the ability of ANTX to stimulate contraction. Based upon these data, it was inferred that ANTX binds to postganglionic synaptic nicotinic receptors in the ileum, thus releasing endogenous ACh, which in turn causes ileum contraction by interacting with the postsynaptic muscarinic receptors. It was also observed that thymopentin (TP-5), a pentapeptide corresponding to positions 32-36 of thymopoietin, blocked the stimulation of ileum contraction by ANTX. |
---|---|
CAS No. |
64285-06-9 |
Molecular Formula |
C10H15NO |
Molecular Weight |
165.23 g/mol |
IUPAC Name |
1-(9-azabicyclo[4.2.1]non-2-en-2-yl)ethanone |
InChI |
InChI=1S/C10H15NO/c1-7(12)9-4-2-3-8-5-6-10(9)11-8/h4,8,10-11H,2-3,5-6H2,1H3 |
InChI Key |
SGNXVBOIDPPRJJ-UHFFFAOYSA-N |
SMILES |
CC(=O)C1=CCCC2CCC1N2 |
Isomeric SMILES |
CC(=O)C1=CCC[C@@H]2CC[C@H]1N2 |
Canonical SMILES |
CC(=O)C1=CCCC2CCC1N2 |
Color/Form |
Oil |
Key on ui other cas no. |
64285-06-9 |
Pictograms |
Irritant; Health Hazard |
solubility |
In water, 7.2X10+4 mg/L at 25 °C (est) |
Synonyms |
1-(1R,6R)-9-Azabicyclo[4.2.1]non-2-en-2-ylethanone; (1R)-1-(9-Azabicyclo[4.2.1]non-2-en-2-yl)ethanone; (+)-Anatoxin a; (+)-Anatoxin α; Anatoxin I; Anatoxin a; Anatoxin a; |
vapor_pressure |
5.8X10-3 mm Hg at 25 °C (est) |
Origin of Product |
United States |
Retrosynthesis Analysis
AI-Powered Synthesis Planning: Our tool employs the Template_relevance Pistachio, Template_relevance Bkms_metabolic, Template_relevance Pistachio_ringbreaker, Template_relevance Reaxys, Template_relevance Reaxys_biocatalysis model, leveraging a vast database of chemical reactions to predict feasible synthetic routes.
One-Step Synthesis Focus: Specifically designed for one-step synthesis, it provides concise and direct routes for your target compounds, streamlining the synthesis process.
Accurate Predictions: Utilizing the extensive PISTACHIO, BKMS_METABOLIC, PISTACHIO_RINGBREAKER, REAXYS, REAXYS_BIOCATALYSIS database, our tool offers high-accuracy predictions, reflecting the latest in chemical research and data.
Strategy Settings
Precursor scoring | Relevance Heuristic |
---|---|
Min. plausibility | 0.01 |
Model | Template_relevance |
Template Set | Pistachio/Bkms_metabolic/Pistachio_ringbreaker/Reaxys/Reaxys_biocatalysis |
Top-N result to add to graph | 6 |
Feasible Synthetic Routes
Disclaimer and Information on In-Vitro Research Products
Please be aware that all articles and product information presented on BenchChem are intended solely for informational purposes. The products available for purchase on BenchChem are specifically designed for in-vitro studies, which are conducted outside of living organisms. In-vitro studies, derived from the Latin term "in glass," involve experiments performed in controlled laboratory settings using cells or tissues. It is important to note that these products are not categorized as medicines or drugs, and they have not received approval from the FDA for the prevention, treatment, or cure of any medical condition, ailment, or disease. We must emphasize that any form of bodily introduction of these products into humans or animals is strictly prohibited by law. It is essential to adhere to these guidelines to ensure compliance with legal and ethical standards in research and experimentation.