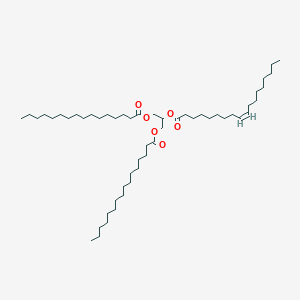
1,3-Dipalmitoyl-2-oleoylglycerol
Overview
Description
1,3-Dipalmitoyl-2-oleoylglycerol (POP) is a structured triacylglycerol (TAG) with two saturated palmitic acid chains esterified at the sn-1 and sn-3 positions of the glycerol backbone and an unsaturated oleic acid chain at the sn-2 position. This unique configuration confers distinct physicochemical and biological properties, making POP a molecule of interest in lipid metabolism, food science, and neuroprotective therapeutics .
Natural Sources and Synthesis:
POP is abundant in natural oils such as rice bran oil (RBO), palm oil, and cocoa butter. For instance, palm mid-fraction (PMF) contains ~67.6% POP by weight . Industrially, POP is synthesized via enzymatic transesterification using lipases (e.g., NS40086) in solvent-free systems, optimizing conditions like temperature (55–60°C) and substrate molar ratios to minimize acyl migration and maximize yield .
Preparation Methods
Chemical Synthesis via Regioselective Acylation
sn-Glycerol-3-Protective Group Strategy
The most widely employed method for POP synthesis involves selective protection of the sn-2 hydroxyl group on glycerol, enabling sequential acylation of the sn-1 and sn-3 positions. As demonstrated in the synthesis of PLP , this process begins with the preparation of 1,3-diacylglycerol intermediates.
Step 1: Protection of sn-2 Hydroxyl Group
Glycerol is treated with trityl chloride or tert-butyldimethylsilyl (TBDMS) chloride in anhydrous dichloromethane under inert conditions. The reaction, catalyzed by 4-dimethylaminopyridine (DMAP), proceeds at room temperature for 6–8 hours, yielding a protected glycerol derivative with free sn-1 and sn-3 hydroxyl groups.
Step 2: Acylation of sn-1 and sn-3 Positions
The protected glycerol undergoes acylation with palmitoyl chloride (2.2 equivalents) in the presence of pyridine as an acid scavenger. Reaction conditions include refluxing in chloroform for 12–16 hours, achieving >95% conversion to 1,3-dipalmitoyl-sn-glycerol . Excess acylating agent ensures complete esterification.
Step 3: Deprotection and sn-2 Acylation
Deprotection is achieved via hydrogenolysis (for trityl groups) or tetrabutylammonium fluoride (TBAF) treatment (for TBDMS groups). The resulting 1,3-dipalmitoyl-sn-glycerol is then acylated at the sn-2 position with oleoyl chloride (1.5 equivalents) under similar conditions, yielding crude POP.
Reaction Summary
Step | Reagents | Conditions | Yield (%) |
---|---|---|---|
Protection | Trityl chloride, DMAP | 25°C, 8 h | 92 |
sn-1/3 Acylation | Palmitoyl chloride, pyridine | Reflux, 16 h | 95 |
Deprotection | H₂/Pd-C | 25°C, 4 h | 89 |
sn-2 Acylation | Oleoyl chloride, pyridine | Reflux, 12 h | 88 |
Enzymatic Synthesis Using Lipase Catalysts
Regioselective Transesterification
Lipases such as Rhizomucor miehei (RM IM) or Thermomyces lanuginosus exhibit strong selectivity for the sn-1 and sn-3 positions, enabling solvent-free POP synthesis. In a typical protocol , glycerol is reacted with methyl palmitate (2.2 equivalents) and methyl oleate (1.0 equivalent) at 60°C for 24–48 hours. The lipase (5–10% w/w) is immobilized on acrylic resin to enhance stability.
Key Parameters
-
Temperature: 60–65°C (optimizes enzyme activity and substrate miscibility)
-
Solvent: Solvent-free systems reduce downstream purification complexity.
-
Yield: 70–75% POP, with <5% 1,2-dipalmitoyl-3-oleoylglycerol byproduct.
Purification and Isolation
Column Chromatography
Crude POP is purified using silica gel chromatography with a hexane:diethyl ether:formic acid (80:20:2 v/v/v) mobile phase. Fractions are analyzed by thin-layer chromatography (TLC), with POP exhibiting an Rₕ value of 0.41 . High-performance liquid chromatography (HPLC) with evaporative light scattering detection (ELSD) confirms purity ≥99% using a C18 column and acetone/acetonitrile (70:30 v/v) isocratic elution .
Recrystallization
Further purification involves recrystallization from chloroform:ethyl acetate (1:3 v/v) at –20°C. This step removes residual diacylglycerols and unreacted fatty acids, achieving >99.5% purity.
Analytical Validation
Mass Spectrometry
Electrospray ionization mass spectrometry (ESI-MS) of POP shows a sodium adduct [M + Na]⁺ at m/z 855.7 (calculated for C₅₃H₁₀₀O₆Na: 855.7) . Isotopic patterns and fragmentation profiles confirm molecular integrity.
Elementary Analysis
Elemental analysis validates stoichiometry:
Element | Theoretical (%) | Observed (%) |
---|---|---|
C | 74.48 | 74.45 ± 0.3 |
H | 11.89 | 11.92 ± 0.2 |
Challenges and Optimization Strategies
Byproduct Formation
Partial acyl migration during sn-2 acylation generates 1,2-dipalmitoyl-3-oleoylglycerol (≤5%). This is mitigated by:
-
Low-Temperature Acylation: Conducting sn-2 acylation at 0–4°C reduces migration.
-
Catalytic DMAP: Limiting DMAP to 0.1 equivalents minimizes base-induced isomerization.
Scalability Considerations
Industrial-scale production employs continuous-flow reactors for enzymatic synthesis, reducing batch variability. Solvent recovery systems (e.g., falling-film evaporators) enhance sustainability.
Chemical Reactions Analysis
Hydrolysis and Stability
POP demonstrates resistance to enzymatic hydrolysis due to its stereospecific structure. Lipases preferentially hydrolyze the sn-1 and sn-3 ester bonds, releasing palmitic acid and leaving a 2-oleoylglycerol intermediate . This property is critical in lipid metabolism studies.
Key Data:
-
Hydrolysis Rate: Slower compared to asymmetrical triglycerides due to steric hindrance from the sn-2 oleate .
-
pH Stability: Stable under neutral conditions but undergoes partial hydrolysis in alkaline environments (pH > 10) .
Oxidation Behavior
-
Oxidative Stress Protection: POP administration in rats reduced lipid peroxidation by 42% in brain tissues post-ischemia, as measured by malondialdehyde (MDA) levels .
-
Antioxidant Mechanism: Enhances glutathione (GSH) levels by 35% in vivo, suggesting indirect antioxidant activity .
Crystallization Dynamics
POP’s crystallization from solvents like acetone is influenced by cooling rates and molecular packing.
Experimental Data:
Parameter | POP (Pure) | POP-PPP Mixtures |
---|---|---|
Metastable Zone Width | 6–8°C (at 1°C/min) | 4–6°C (at 1°C/min) |
Crystal Morphology | Spherulitic | Plate-like aggregates |
Cooling Rate Effect | Low sensitivity | Higher sensitivity |
-
Solvent Interaction: Crystallizes in open spherulitic structures at slower cooling rates, favoring solvent inclusion .
Synthetic and Purification Reactions
POP is synthesized via stereoselective esterification and purified using chromatographic methods:
-
Esterification: Glycerol reacts with palmitoyl chloride (stepwise at sn-1/3) and oleoyl chloride (sn-2) under controlled catalysis.
-
Purification:
Yield Optimization:
-
Temperature: 45–50°C maximizes esterification efficiency.
-
Catalyst: Lipase B from Candida antarctica shows 88% regioselectivity .
Biochemical Interactions
POP modulates signaling pathways in biological systems:
-
Neuroprotection: Inhibits p38 MAPK phosphorylation by 60% and activates PI3K/Akt/CREB pathways, reducing cerebral infarct volume by 55% in MCAO/R rats .
-
Metabolic Fate: Primarily absorbed as 2-oleoylglycerol post-hydrolysis, influencing lipid trafficking and storage .
Comparative Reactivity Table
Reaction Type | POP Reactivity | Comparison to PPP |
---|---|---|
Hydrolysis | Moderate (sn-1/3) | High (all sn positions) |
Oxidation | Higher (C18:1 site) | Low (saturated only) |
Crystallization Rate | Slower | Faster |
Scientific Research Applications
Food Science and Lipid Stability
DPOG plays a significant role in understanding the oxidative stability of triglycerides in food products. Research has demonstrated that DPOG can be used to elucidate the decomposition products of triglycerides under high-temperature conditions, such as frying. A study highlighted its importance in oxidative lipidomics, showing that DPOG helps assess the stability of vegetable oils during frying, which is crucial for food safety and quality control (Hu et al., 2023) .
Case Study: Oxidative Stability Assessment
- Objective : To evaluate the oxidative stability of various triglycerides during frying.
- Method : DPOG was subjected to simulated frying conditions to analyze its decomposition products.
- Findings : The study concluded that DPOG's structure influences its oxidative stability, making it a valuable compound for improving the shelf life of fried foods.
Lipid Metabolism Research
DPOG has been investigated for its effects on lipid metabolism and fat accumulation. A study involving C57BL/6 mice explored how specific triglyceride configurations, including DPOG, affect fat deposition. The results indicated that certain configurations could limit fat accumulation more effectively than others, providing insights into potential therapeutic strategies for obesity and related metabolic disorders (Gouk et al., 2014) .
Case Study: Lipid Metabolism in Mice
- Objective : To assess the impact of triglyceride configurations on lipid metabolism.
- Method : Mice were fed diets containing different triglyceride forms, including DPOG.
- Findings : DPOG exhibited a significant effect on reducing fat deposition compared to other triglycerides.
Neuroprotective Effects
DPOG has shown promise in neuroprotection, particularly against cerebral ischemia-reperfusion injury. A study conducted on rats demonstrated that DPOG derived from rice bran oil could mitigate neurological deficits and reduce infarct size following induced ischemia (Zhang et al., 2022) . This suggests potential applications in treating neurodegenerative diseases.
Case Study: Neuroprotection in Rats
- Objective : To evaluate the neuroprotective effects of DPOG in ischemic conditions.
- Method : Rats underwent middle cerebral artery occlusion followed by treatment with DPOG.
- Findings : Treatment with DPOG significantly reduced infarct size and improved neurological function.
Digestion and Absorption Studies
Recent research has focused on the digestion and absorption properties of DPOG-rich lipids using in vitro gastrointestinal models. This investigation is vital for understanding how different lipid structures affect nutrient absorption and metabolism (Kim et al., 2024) .
Case Study: In Vitro Digestion Analysis
- Objective : To assess the digestion and absorption characteristics of DPOG-rich lipids.
- Method : An in vitro gastrointestinal digestion model was employed alongside Caco-2 cell assays to mimic human digestion.
- Findings : Results indicated that DPOG enhances nutrient absorption efficiency compared to other triglycerides.
Crystallization Behavior
The crystallization behavior of DPOG is critical for applications in food technology and pharmaceuticals. Understanding how this compound crystallizes can inform its use in creating stable emulsions and solid fats (Huang et al., 2021) .
Case Study: Crystallization Kinetics
- Objective : To analyze the crystallization kinetics of DPOG under varying temperature conditions.
- Method : Synchrotron radiation time-resolved X-ray diffraction was used to observe crystallization patterns.
- Findings : The study revealed distinct crystallization behaviors that could be manipulated for desired physical properties in food products.
Mechanism of Action
The mechanism by which 1,3-Dipalmitoyl-2-oleoylglycerol exerts its effects involves several molecular targets and pathways:
Neuroprotection: It has been shown to reduce oxidative stress and inflammation in the brain by modulating pathways such as the p38 MAPK and PI3K/Akt/CREB pathways.
Lipid Metabolism: The compound influences lipid metabolism by affecting the activity of enzymes involved in fat deposition and mobilization.
Comparison with Similar Compounds
Key Physicochemical Properties :
- Molecular formula : C53H100O6 (average mass: 833.36 g/mol) .
- Melting point : ~47°C, influenced by its mixed saturated-unsaturated fatty acid profile .
- Crystallization behavior: Non-isothermal differential scanning calorimetry (DSC) reveals distinct phase transitions, critical for food applications like cocoa butter substitutes .
Biological Significance: POP demonstrates neuroprotective effects in rat models of middle cerebral artery occlusion/reperfusion (MCAO/R) injury. At doses of 1–5 mg/kg, POP reduces cerebral infarction volume by 30–50%, mitigates oxidative stress (e.g., restores glutathione levels), and suppresses pro-apoptotic proteins (Bax, caspase-3) while enhancing anti-apoptotic Bcl-2 expression .
POP belongs to a broader class of structured TAGs. Below, we compare its structural, functional, and biological attributes with analogous compounds:
Structural and Physicochemical Comparisons
Table 1: Structural and Thermal Properties of POP and Analogous TAGs
Key Observations :
- Saturation vs. Unsaturation : POP’s sn-2 oleic acid enhances membrane fluidity compared to fully saturated TAGs like SOS. Conversely, SOS’s stearic acid chains confer higher melting points, critical for chocolate tempering .
- Crystallization: POP and OPO mixtures form a molecular compound (βC) with monotectic phases, influencing lipid matrices in food and pharmaceutical formulations .
- Oxidative Stability: SLS, with linoleic acid at sn-2, is more prone to oxidation than POP, limiting its shelf-life in lipid-based products .
Key Observations :
- Neuroprotection Uniqueness : POP’s neuroprotective efficacy is unmatched among compared TAGs, likely due to its synergistic modulation of p38 MAPK (anti-apoptotic) and PI3K/Akt/CREB (pro-survival) pathways .
- Nutritional Applications : OPO and SOS are prioritized in food science for texture and digestibility, whereas POP bridges nutrition and therapeutics .
- Antioxidant Mechanisms : POP’s restoration of glutathione parallels phytosterols like NMN, but its TAG structure enhances bioavailability compared to free fatty acids .
Q & A
Basic Research Questions
Q. What enzymatic methods are optimal for synthesizing POP in solvent-free systems?
POP is synthesized via enzymatic transesterification using lipases such as NS40086 (from Rhizomucor miehei) or Thermomyces lanuginosus. Key parameters include:
- Substrate ratio : A 1:2 molar ratio of high-oleic peanut oil to ethyl palmitate maximizes POP yield by reducing acyl migration .
- Temperature : 60–70°C balances enzyme activity and stability.
- Lipase loading : 8–12% (w/w) of total substrates ensures efficient catalysis without excessive cost .
- Reaction time : 6–8 hours under vacuum minimizes side reactions. Optimization via response surface methodology (RSM) is recommended to account for interactions between variables .
Optimal Conditions (NS40086 Lipase) |
---|
Temperature: 65°C |
Substrate ratio (oil:ethyl palmitate): 1:2 |
Enzyme loading: 10% (w/w) |
Reaction time: 7 hours |
Q. How can acyl migration during POP synthesis be minimized?
Acyl migration (undesired positional rearrangement of fatty acids) is reduced by:
- Using 1,3-regioselective lipases (e.g., NS40086) .
- Limiting reaction time to <8 hours and maintaining temperatures below 70°C .
- Avoiding polar solvents, which increase migration rates. Solvent-free systems are preferred .
Q. What analytical techniques validate POP purity and regioisomeric structure?
- HPLC-MS : Quantifies POP content and detects sn-2 oleic acid positional specificity .
- X-ray diffraction (XRD) : Confirms crystalline structure and polymorphism (e.g., β vs. β' phases) .
- Differential Scanning Calorimetry (DSC) : Measures melting profiles to identify molecular compounds in mixtures (e.g., POP/PPO systems) .
Advanced Research Questions
Q. How does POP’s phase behavior in binary mixtures influence crystallization kinetics?
POP forms molecular compounds with regioisomers like PPO (1,2-dipalmitoyl-3-oleoylglycerol) at a 1:1 ratio. Key findings:
- Monotectic phase separation occurs in POP/PPO systems, with βC (a double-chain-length structure) exhibiting a melting point of 31.2°C .
- Metastable αC and β'C phases form during rapid cooling, impacting nucleation rates .
- Time-resolved synchrotron XRD is critical for tracking transient polymorphic transitions .
Q. What kinetic models describe nonisothermal crystallization of POP?
Nonisothermal DSC data are best fitted by the Johnson-Mehl-Avrami-Erofeyev-Kolmogorov (JMAEK) model. Key parameters:
- Activation energy (Ea) : Derived via isoconversional methods (e.g., Starink analysis) .
- Vogel-Fulcher equation : Describes temperature dependence of crystallization rate constants, where (reference temperature) correlates with POP’s melting point . Example workflow:
- Cool melted POP at 2°C/min to induce crystallization.
- Analyze DSC peaks using software (e.g., Netzsch Proteus) to extract Ea and nucleation order.
Q. How can POP be used as a model compound in irradiation studies?
POP serves as a substrate to detect 2-alkylcyclobutanones (2-ACBs) , markers of food irradiation. Method:
- Irradiate POP (10–50 kGy) and extract 2-ACBs via solid-phase microextraction (SPME).
- Analyze using GC-MS/MS with derivatization (e.g., BSTFA) for enhanced sensitivity (LOD: 0.1 µg/kg) .
- Compare with non-irradiated controls to validate irradiation-specific byproducts.
Q. What are the challenges in resolving POP-containing TAG mixtures via chromatography?
Co-elution of regioisomers (e.g., POP vs. PPO) requires:
- Silver-ion HPLC : Separates TAGs based on double-bond interactions .
- Electrospray ionization tandem MS : Differentiates regioisomers via fragmentation patterns (e.g., sn-2 vs. sn-1/3 cleavage) .
- Supercritical fluid chromatography (SFC) : Provides high-resolution separation with CO₂-based mobile phases .
Q. Methodological Considerations
- Contradictions in phase studies : While reports βC as a stable POP/PPO compound, highlights metastable αC/β'C phases under non-equilibrium conditions. Researchers should specify thermal history when comparing results.
- Synthesis scalability : Solvent-free enzymatic methods ( ) are lab-efficient but may require pressurized reactors for industrial translation.
Properties
IUPAC Name |
1,3-di(hexadecanoyloxy)propan-2-yl octadec-9-enoate | |
---|---|---|
Details | Computed by Lexichem TK 2.7.0 (PubChem release 2021.05.07) | |
Source | PubChem | |
URL | https://pubchem.ncbi.nlm.nih.gov | |
Description | Data deposited in or computed by PubChem | |
InChI |
InChI=1S/C53H100O6/c1-4-7-10-13-16-19-22-25-26-29-32-35-38-41-44-47-53(56)59-50(48-57-51(54)45-42-39-36-33-30-27-23-20-17-14-11-8-5-2)49-58-52(55)46-43-40-37-34-31-28-24-21-18-15-12-9-6-3/h25-26,50H,4-24,27-49H2,1-3H3 | |
Details | Computed by InChI 1.0.6 (PubChem release 2021.05.07) | |
Source | PubChem | |
URL | https://pubchem.ncbi.nlm.nih.gov | |
Description | Data deposited in or computed by PubChem | |
InChI Key |
FDCOHGHEADZEGF-UHFFFAOYSA-N | |
Details | Computed by InChI 1.0.6 (PubChem release 2021.05.07) | |
Source | PubChem | |
URL | https://pubchem.ncbi.nlm.nih.gov | |
Description | Data deposited in or computed by PubChem | |
Canonical SMILES |
CCCCCCCCCCCCCCCC(=O)OCC(COC(=O)CCCCCCCCCCCCCCC)OC(=O)CCCCCCCC=CCCCCCCCC | |
Details | Computed by OEChem 2.3.0 (PubChem release 2021.05.07) | |
Source | PubChem | |
URL | https://pubchem.ncbi.nlm.nih.gov | |
Description | Data deposited in or computed by PubChem | |
Molecular Formula |
C53H100O6 | |
Details | Computed by PubChem 2.1 (PubChem release 2021.05.07) | |
Source | PubChem | |
URL | https://pubchem.ncbi.nlm.nih.gov | |
Description | Data deposited in or computed by PubChem | |
DSSTOX Substance ID |
DTXSID40392618 | |
Record name | 1,3-Dipalmitoyl-2-oleoylglycerol | |
Source | EPA DSSTox | |
URL | https://comptox.epa.gov/dashboard/DTXSID40392618 | |
Description | DSSTox provides a high quality public chemistry resource for supporting improved predictive toxicology. | |
Molecular Weight |
833.4 g/mol | |
Details | Computed by PubChem 2.1 (PubChem release 2021.05.07) | |
Source | PubChem | |
URL | https://pubchem.ncbi.nlm.nih.gov | |
Description | Data deposited in or computed by PubChem | |
CAS No. |
2190-25-2 | |
Record name | 1,3-Dipalmitoyl-2-oleoylglycerol | |
Source | EPA DSSTox | |
URL | https://comptox.epa.gov/dashboard/DTXSID40392618 | |
Description | DSSTox provides a high quality public chemistry resource for supporting improved predictive toxicology. | |
Retrosynthesis Analysis
AI-Powered Synthesis Planning: Our tool employs the Template_relevance Pistachio, Template_relevance Bkms_metabolic, Template_relevance Pistachio_ringbreaker, Template_relevance Reaxys, Template_relevance Reaxys_biocatalysis model, leveraging a vast database of chemical reactions to predict feasible synthetic routes.
One-Step Synthesis Focus: Specifically designed for one-step synthesis, it provides concise and direct routes for your target compounds, streamlining the synthesis process.
Accurate Predictions: Utilizing the extensive PISTACHIO, BKMS_METABOLIC, PISTACHIO_RINGBREAKER, REAXYS, REAXYS_BIOCATALYSIS database, our tool offers high-accuracy predictions, reflecting the latest in chemical research and data.
Strategy Settings
Precursor scoring | Relevance Heuristic |
---|---|
Min. plausibility | 0.01 |
Model | Template_relevance |
Template Set | Pistachio/Bkms_metabolic/Pistachio_ringbreaker/Reaxys/Reaxys_biocatalysis |
Top-N result to add to graph | 6 |
Feasible Synthetic Routes
Disclaimer and Information on In-Vitro Research Products
Please be aware that all articles and product information presented on BenchChem are intended solely for informational purposes. The products available for purchase on BenchChem are specifically designed for in-vitro studies, which are conducted outside of living organisms. In-vitro studies, derived from the Latin term "in glass," involve experiments performed in controlled laboratory settings using cells or tissues. It is important to note that these products are not categorized as medicines or drugs, and they have not received approval from the FDA for the prevention, treatment, or cure of any medical condition, ailment, or disease. We must emphasize that any form of bodily introduction of these products into humans or animals is strictly prohibited by law. It is essential to adhere to these guidelines to ensure compliance with legal and ethical standards in research and experimentation.