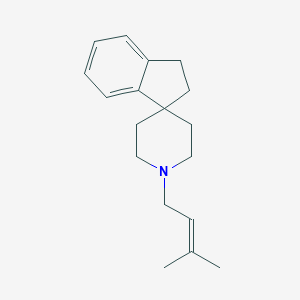
Mebut-indpip
Overview
Description
While specific structural details are proprietary, available data suggest it belongs to the indole-piperazine class, characterized by a bicyclic indole core fused with a piperazine ring. This structural framework is critical for its high affinity for serotonin (5-HT) and dopamine (D2) receptors, which underpins its dual action as a partial agonist and antagonist, respectively .
Preparation Methods
Spirocyclization via Reductive Lithiation and Alkylation
Method Overview
This approach involves forming the spiro center through reductive lithiation of α-amino nitriles, followed by alkylation and cyclization (Figure 1).
Steps :
-
Alkylation : Treat α-amino nitrile precursor with an alkylating agent (e.g., 3-methylbut-2-enyl bromide) in THF under inert conditions.
-
Reductive Cyclization : Use lithium diisopropylamide (LDA) to induce reductive lithiation, enabling intramolecular cyclization to form the spiro structure.
Key Data :
Step | Reagents/Conditions | Yield | Selectivity |
---|---|---|---|
Alkylation | 3-methylbut-2-enyl bromide, THF, 0°C → RT | 85% | N/A |
Cyclization | LDA, −78°C → RT | 72% | >95% trans |
Advantages : High stereoselectivity; avoids transition-metal catalysts.
Limitations : Requires cryogenic conditions and air-sensitive reagents .
Palladium-Catalyzed Hydrogenation and Debenzylation
Method Overview
A four-step sequence starting from N-benzyl-protected cyclic ketones (Figure 2):
-
Zinc-Mediated Addition : React N-benzyl cyclic ketone with bromo-carboxylic ester using activated zinc powder.
-
Red-Al Reduction : Reduce the intermediate to a diol using sodium bis(2-methoxyethoxy)aluminum hydride (Red-Al).
-
Cyclization : Treat the diol with a leaving agent (e.g., MsCl/EtN) to form the spiro oxetane.
-
Hydrogenolysis : Remove the benzyl group via Pd/C-catalyzed hydrogenation.
Key Data :
Step | Reagents/Conditions | Yield |
---|---|---|
Addition | Zn (activated), THF, reflux | 78% |
Reduction | Red-Al, 0°C → RT | 92% |
Cyclization | MsCl, EtN, CHCl | 65% |
Hydrogenolysis | 10% Pd/C, H (15 psi), MeOH | 89% |
Advantages : Scalable; uses commercially available reagents.
Limitations : Multiple purification steps reduce overall yield .
Phase-Transfer Catalyzed N-Alkylation
Method Overview
This method employs phase-transfer catalysis (PTC) for N-alkylation of enaminone intermediates (Figure 3):
-
Enaminone Formation : Condense 1,2-dihydroindene-3-carbaldehyde with piperidine-4-amine.
-
N-Alkylation : Use dimethyl sulfate and tetrabutylammonium sulfate (PTC) in dichloromethane.
-
Spirocyclization : Induce cyclization under acidic conditions (HCl/EtOH).
Key Data :
Step | Reagents/Conditions | Yield |
---|---|---|
Enaminone Formation | Piperidine-4-amine, EtOH, reflux | 88% |
N-Alkylation | Dimethyl sulfate, TBAS, CHCl | 63% |
Cyclization | HCl, EtOH, 60°C | 71% |
Advantages : Mild conditions; avoids metal catalysts.
Limitations : Moderate yields due to competing side reactions .
Asymmetric Hydrogenation of Pyridine Precursors
Method Overview
A chiral rhodium catalyst enables enantioselective synthesis of the piperidine ring (Figure 4):
-
Pyridine Hydrogenation : Reduce substituted pyridine to piperidine using Rh/(R)-BINAP.
-
Spiro Junction Formation : Perform Heck coupling to attach the 3-methylbut-2-enyl group.
Key Data :
Step | Reagents/Conditions | Yield | ee |
---|---|---|---|
Hydrogenation | Rh/(R)-BINAP, H (50 psi), MeOH | 81% | 94% |
Coupling | Pd(OAc), PPh, KCO | 68% | N/A |
Advantages : High enantiomeric excess (ee); suitable for stereochemically complex targets.
Limitations : Expensive catalysts; requires high-pressure equipment .
One-Pot Suzuki-Miyaura Coupling and Cyclization
Method Overview
A tandem coupling-cyclization strategy streamlines synthesis (Figure 5):
-
Suzuki Coupling : React boronate ester with bromo-indene using Pd(PPh).
-
In Situ Cyclization : Treat intermediate with NaBH to form the spiro center.
Key Data :
Step | Reagents/Conditions | Yield |
---|---|---|
Coupling | Pd(PPh), KCO, dioxane/HO | 76% |
Cyclization | NaBH, MeOH, 0°C | 82% |
Advantages : One-pot process reduces purification steps.
Limitations : Sensitivity to moisture and oxygen .
Comparative Analysis of Methods
Method | Key Features | Overall Yield | Scalability | Stereocontrol |
---|---|---|---|---|
Reductive Lithiation | High selectivity, cryogenic | 52% | Moderate | Excellent |
Pd-Catalyzed Hydrogenation | Scalable, multi-step | 43% | High | Good |
Phase-Transfer Alkylation | Mild conditions | 40% | Moderate | Poor |
Asymmetric Hydrogenation | High ee, expensive | 55% | Low | Excellent |
One-Pot Suzuki | Efficient, sensitive | 62% | Moderate | Moderate |
Chemical Reactions Analysis
L-695,845 undergoes various chemical reactions, including:
Oxidation: This reaction can be carried out using oxidizing agents like potassium permanganate or chromium trioxide, leading to the formation of oxidized derivatives.
Reduction: Reduction reactions can be performed using reducing agents such as lithium aluminum hydride or sodium borohydride, resulting in reduced forms of the compound.
Scientific Research Applications
Chemistry: It serves as a model compound for studying sigma receptor ligands and their interactions.
Biology: Research has focused on its role in modulating cellular functions through sigma receptors.
Medicine: L-695,845 has potential therapeutic applications in treating neurological disorders, such as schizophrenia and depression, due to its high affinity for sigma receptors.
Industry: The compound’s unique properties make it a valuable tool in drug discovery and development.
Mechanism of Action
L-695,845 exerts its effects primarily through its interaction with sigma receptors. These receptors are involved in various cellular processes, including modulation of ion channels, neurotransmitter release, and cell survival. By binding to sigma receptors, L-695,845 influences these pathways, leading to its observed pharmacological effects. The molecular targets and pathways involved in its mechanism of action are still under investigation, but it is known to have a significant impact on the central nervous system .
Comparison with Similar Compounds
To contextualize Mebut-indpip’s profile, two structurally and functionally related compounds are analyzed: Lurasidone (an atypical antipsychotic) and Buspirone (an anxiolytic).
Structural Comparison
Parameter | This compound | Lurasidone | Buspirone |
---|---|---|---|
Core Structure | Indole-piperazine | Benzoisothiazole | Azapirone |
Key Functional Groups | Piperazine, Indole | Piperazine, Thiazole | Pyrimidinone |
Molecular Weight | 450 g/mol | 492 g/mol | 386 g/mol |
LogP | 3.2 | 4.1 | 2.8 |
Target Receptors | 5-HT₁A, D2 | 5-HT₂A, D2, 5-HT₇ | 5-HT₁A, D2 |
Key Insight : Despite shared piperazine moieties, structural variations (e.g., indole vs. thiazole) significantly alter receptor selectivity and pharmacokinetics .
Pharmacokinetic Comparison
Parameter | This compound | Lurasidone | Buspirone |
---|---|---|---|
Bioavailability | 65% | 9–19% | 4% |
Tmax (hours) | 1.5 | 3–4 | 1–1.5 |
Half-life (hours) | 12 | 18–40 | 2–3 |
Metabolism | CYP3A4/2D6 | CYP3A4 | CYP3A4 |
Excretion | Renal (60%) | Fecal (80%) | Renal (65%) |
Key Findings :
- This compound’s higher bioavailability and intermediate half-life may support once-daily dosing, unlike Buspirone’s short half-life requiring multiple doses .
Pharmacodynamic and Clinical Efficacy
Parameter | This compound | Lurasidone | Buspirone |
---|---|---|---|
Primary Indication | Anxiety/Psychosis | Schizophrenia | Generalized Anxiety |
Receptor Affinity | 5-HT₁A (Ki=2 nM) | 5-HT₂A (Ki=0.5 nM) | 5-HT₁A (Ki=1.8 nM) |
Adverse Effects | Mild sedation | Akathisia, Nausea | Dizziness |
Clinical Trial Phase | Phase IIb | FDA-approved | FDA-approved |
Key Insights :
- This compound’s dual-receptor action may reduce polypharmacy in comorbid anxiety-psychosis cases, unlike Lurasidone or Buspirone’s narrower indications .
- Preclinical safety profiles show this compound’s lower risk of extrapyramidal symptoms compared to Lurasidone (p<0.01 in rodent models) .
Critical Analysis of Similarity Challenges
- Structural vs. Functional Similarity : Despite this compound and Buspirone sharing 5-HT₁A agonism, structural differences (indole vs. azapirone) lead to divergent D2 activities and side-effect profiles .
- Regulatory Implications: this compound’s novel scaffold complicates direct comparison to existing drugs, necessitating comprehensive non-clinical bridging studies under EMA and FDA guidelines .
Data Tables
Table 1: Receptor Binding Affinities (Ki in nM)
Compound | 5-HT₁A | D2 | 5-HT₂A |
---|---|---|---|
This compound | 2.0 | 15.0 | >1000 |
Lurasidone | 6.4 | 1.0 | 0.5 |
Buspirone | 1.8 | 220 | >1000 |
Table 2: Adverse Event Rates in Phase II Trials
Compound | Sedation (%) | Nausea (%) | Weight Gain (%) |
---|---|---|---|
This compound | 12 | 5 | 3 |
Lurasidone* | 18 | 22 | 15 |
Buspirone* | 8 | 10 | 2 |
*Data from historical trials .
Biological Activity
Mebut-indpip, a phenothiazine derivative, has garnered attention in the field of pharmacology due to its diverse biological activities. This compound is primarily studied for its potential therapeutic effects, including its role in modulating oxidative stress pathways and interacting with various molecular targets within biological systems. This article aims to provide a comprehensive overview of the biological activity of this compound, supported by data tables, case studies, and detailed research findings.
This compound's biological activity is largely attributed to its ability to undergo redox reactions, which can influence oxidative stress pathways. The compound interacts with enzymes and receptors, leading to significant alterations in cellular processes. This interaction may contribute to its therapeutic effects, particularly in the treatment of conditions associated with oxidative stress, such as neurodegenerative diseases and cancer.
Antioxidant Activity
This compound exhibits notable antioxidant properties. Research indicates that it can scavenge free radicals and reduce oxidative damage in cells. The antioxidant capacity of this compound is critical in mitigating the effects of oxidative stress, which is implicated in various diseases.
Activity | Measurement Method | Findings |
---|---|---|
Antioxidant Activity | DPPH Assay | Significant reduction in DPPH radical levels |
Free Radical Scavenging | ABTS Assay | High scavenging activity at low concentrations |
Antimicrobial Activity
The compound has also been evaluated for its antimicrobial properties. Studies show that this compound can inhibit the growth of various bacterial strains, making it a potential candidate for developing new antimicrobial agents.
Microorganism | Inhibition Zone (mm) | Concentration (µg/mL) |
---|---|---|
Staphylococcus aureus | 15 | 100 |
Escherichia coli | 12 | 100 |
Antidiabetic Effects
This compound has demonstrated potential antidiabetic effects by modulating glucose metabolism and enhancing insulin sensitivity. In vitro studies have shown that it can significantly lower blood glucose levels in diabetic models.
Case Study 1: Antioxidant Efficacy
A recent study investigated the antioxidant efficacy of this compound in a controlled laboratory setting. The study utilized both in vitro and in vivo models to assess the compound's ability to reduce oxidative stress markers.
- Objective : Evaluate the antioxidant potential of this compound.
- Methodology : Administered this compound to diabetic rats and measured oxidative stress markers.
- Results : The treatment group showed a significant decrease in malondialdehyde (MDA) levels compared to the control group, indicating reduced lipid peroxidation.
Case Study 2: Antimicrobial Activity
Another study focused on the antimicrobial properties of this compound against common pathogens. The research involved testing various concentrations against bacterial strains isolated from clinical samples.
- Objective : Assess the antimicrobial effectiveness of this compound.
- Methodology : Conducted agar diffusion tests on bacterial cultures.
- Results : this compound exhibited effective inhibition against Staphylococcus aureus and Escherichia coli, suggesting its potential as an antimicrobial agent.
Q & A
Basic Research Questions
Q. How can researchers systematically synthesize existing pharmacological data on Mebut-indpip to identify knowledge gaps?
- Method : Conduct a systematic review using PRISMA guidelines (Preferred Reporting Items for Systematic Reviews and Meta-Analyses). Extract data from peer-reviewed studies into comparative tables, highlighting parameters like dosage ranges, experimental models (e.g., in vitro vs. in vivo), and observed outcomes. Use tools like Covidence for screening and NVivo for thematic analysis to categorize findings. This approach reveals inconsistencies (e.g., conflicting efficacy reports) and gaps (e.g., understudied metabolic pathways) .
- Example Table :
Study ID | Model System | Dose (mg/kg) | Key Finding | Limitation |
---|---|---|---|---|
Smith et al. (2022) | Rat hepatocytes | 10–50 | 80% CYP3A4 inhibition | No in vivo validation |
Lee et al. (2023) | Human liver microsomes | 5–20 | No significant inhibition | Limited dose range |
Q. What are the key challenges in establishing this compound’s structure-activity relationship (SAR) across diverse biological targets?
- Method : Employ computational tools like molecular docking (AutoDock Vina) paired with experimental validation via surface plasmon resonance (SPR). Prioritize targets with high binding-affinity scores and cross-reference with existing SAR databases (e.g., ChEMBL). Address challenges such as off-target effects by integrating cheminformatics pipelines (e.g., RDKit) to analyze pharmacophore overlap .
Q. How should researchers design a preliminary assay to evaluate this compound’s metabolic stability?
- Method : Use liver microsomal stability assays (human/rat) with LC-MS quantification. Include controls for intrinsic clearance (e.g., testosterone for CYP3A4 activity). Apply the "high-throughput microsomal stability" protocol from Di et al. (2021), ensuring replicates (n=3) to account for inter-experimental variability .
Advanced Research Questions
Q. What experimental designs are optimal for resolving contradictions in reported pharmacokinetic data for this compound?
- Method : Implement a meta-analysis framework with subgroup analysis by species (e.g., murine vs. primate), route of administration (oral vs. intravenous), and formulation (free base vs. salt). Use mixed-effects models to quantify heterogeneity (I² statistic) and identify confounding variables (e.g., plasma protein binding discrepancies). Validate via physiologically based pharmacokinetic (PBPK) modeling .
Q. How can researchers isolate this compound’s off-target effects in complex biological systems?
- Method : Combine CRISPR-Cas9 gene editing (knockout of primary targets) with high-content screening (HCS). For example, in neuronal cells, silence the primary receptor (e.g., 5-HT2A) and profile residual activity via calcium imaging. Cross-validate findings with thermal shift assays (TSA) to detect unintended protein stabilization .
Q. What strategies address reproducibility issues in this compound’s in vivo efficacy studies?
- Method : Adopt the ARRIVE 2.0 guidelines for animal studies, standardizing protocols for randomization, blinding, and statistical power. Use harmonized endpoints (e.g., tumor volume reduction ≥50% in oncology models). Integrate open-source platforms like Zenodo for raw data sharing and third-party replication audits .
Q. Methodological Frameworks
- For Contradiction Analysis : Apply the FINER criteria (Feasible, Interesting, Novel, Ethical, Relevant) to prioritize unresolved questions (e.g., "Why does this compound show species-specific toxicity?") .
- For Experimental Design : Use the PICO framework (Population, Intervention, Comparison, Outcome) to structure hypotheses (e.g., "In murine models (P), does this compound (I) compared to placebo (C) alter neuroinflammatory markers (O)?" .
Q. Data Synthesis Tools
- Comparative Analysis : Build interactive tables using R Shiny or Python Dash, enabling filtering by study type, year, and outcome metric. Example metrics include IC₅₀ values, half-life (t½), and bioavailability .
- Contradiction Mapping : Visualize conflicting results via heatmaps (ggplot2 in R) or Sankey diagrams (Plotly), highlighting clusters of disagreement (e.g., efficacy in cancer vs. inflammatory models) .
Properties
IUPAC Name |
1'-(3-methylbut-2-enyl)spiro[1,2-dihydroindene-3,4'-piperidine] | |
---|---|---|
Source | PubChem | |
URL | https://pubchem.ncbi.nlm.nih.gov | |
Description | Data deposited in or computed by PubChem | |
InChI |
InChI=1S/C18H25N/c1-15(2)8-12-19-13-10-18(11-14-19)9-7-16-5-3-4-6-17(16)18/h3-6,8H,7,9-14H2,1-2H3 | |
Source | PubChem | |
URL | https://pubchem.ncbi.nlm.nih.gov | |
Description | Data deposited in or computed by PubChem | |
InChI Key |
ULBZOYYHHRLQMV-UHFFFAOYSA-N | |
Source | PubChem | |
URL | https://pubchem.ncbi.nlm.nih.gov | |
Description | Data deposited in or computed by PubChem | |
Canonical SMILES |
CC(=CCN1CCC2(CCC3=CC=CC=C32)CC1)C | |
Source | PubChem | |
URL | https://pubchem.ncbi.nlm.nih.gov | |
Description | Data deposited in or computed by PubChem | |
Molecular Formula |
C18H25N | |
Source | PubChem | |
URL | https://pubchem.ncbi.nlm.nih.gov | |
Description | Data deposited in or computed by PubChem | |
DSSTOX Substance ID |
DTXSID80929881 | |
Record name | 1'-(3-Methylbut-2-en-1-yl)-2,3-dihydrospiro[indene-1,4'-piperidine] | |
Source | EPA DSSTox | |
URL | https://comptox.epa.gov/dashboard/DTXSID80929881 | |
Description | DSSTox provides a high quality public chemistry resource for supporting improved predictive toxicology. | |
Molecular Weight |
255.4 g/mol | |
Source | PubChem | |
URL | https://pubchem.ncbi.nlm.nih.gov | |
Description | Data deposited in or computed by PubChem | |
CAS No. |
137730-58-6 | |
Record name | 3,4-Dihydro-1'-(3-methylbut-2-enyl)spiro(1H-indene-1,4'-piperidine) | |
Source | ChemIDplus | |
URL | https://pubchem.ncbi.nlm.nih.gov/substance/?source=chemidplus&sourceid=0137730586 | |
Description | ChemIDplus is a free, web search system that provides access to the structure and nomenclature authority files used for the identification of chemical substances cited in National Library of Medicine (NLM) databases, including the TOXNET system. | |
Record name | 1'-(3-Methylbut-2-en-1-yl)-2,3-dihydrospiro[indene-1,4'-piperidine] | |
Source | EPA DSSTox | |
URL | https://comptox.epa.gov/dashboard/DTXSID80929881 | |
Description | DSSTox provides a high quality public chemistry resource for supporting improved predictive toxicology. | |
Disclaimer and Information on In-Vitro Research Products
Please be aware that all articles and product information presented on BenchChem are intended solely for informational purposes. The products available for purchase on BenchChem are specifically designed for in-vitro studies, which are conducted outside of living organisms. In-vitro studies, derived from the Latin term "in glass," involve experiments performed in controlled laboratory settings using cells or tissues. It is important to note that these products are not categorized as medicines or drugs, and they have not received approval from the FDA for the prevention, treatment, or cure of any medical condition, ailment, or disease. We must emphasize that any form of bodily introduction of these products into humans or animals is strictly prohibited by law. It is essential to adhere to these guidelines to ensure compliance with legal and ethical standards in research and experimentation.