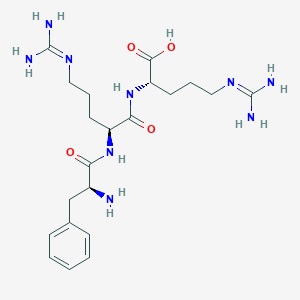
H-Phe-Arg-Arg-OH
Overview
Description
H-Phe-Arg-Arg-OH is a tripeptide composed of phenylalanine (Phe) followed by two arginine (Arg) residues. Industrially, it is listed as a reagent in microbiological assays (e.g., Clostridium perfringens detection) .
Preparation Methods
Classical Solution-Phase Synthesis
Protective Group Strategies
The synthesis of H-Phe-Arg-Arg-OH in solution phase requires orthogonal protection of α-amino and side-chain functionalities. Early work by Schwyzer and Li demonstrated the utility of the p-toluenesulphonyl (tosyl) group for arginine’s guanidino moiety, which resists catalytic hydrogenation and is cleaved via sodium in liquid ammonia . For the α-amino group of phenylalanine, the p-(p′-methoxy-phenylazo)-benzyloxy carbonyl (MZ) group has been employed, as it offers stability during peptide elongation and selective removal under mild acidic conditions .
Stepwise Assembly
-
C-Terminal Arginine Protection : The first arginine residue is introduced with its guanidino group protected by tosyl (Tos) and α-amino group by Boc (tert-butoxycarbonyl). Activation of the carboxyl group via mixed carbonic anhydride or carbodiimide methods facilitates coupling to the second arginine .
-
Intermediate Deprotection : Boc is removed using trifluoroacetic acid (TFA), exposing the α-amino group for subsequent coupling to phenylalanine. The Tos group remains intact during this step .
-
Final Deprotection : Sodium in liquid ammonia cleaves Tos groups, yielding free guanidino functionalities, while the MZ group on phenylalanine is removed via hydrogenolysis .
Challenges and Optimizations
-
Side Reactions : The basicity of arginine’s guanidino group necessitates robust protection to prevent lactamization or racemization. Nitro (NO₂) groups, as described in bitter peptide syntheses, offer an alternative to Tos, with removal via hydrogenation over palladium .
-
Yield Considerations : Sequential coupling of arginine residues risks incomplete reactions due to steric hindrance. Double coupling protocols with dicyclohexylcarbodiimide (DCC) or ethyl cyano(hydroxyimino)acetate (OxymaPure) improve efficiencies .
Solid-Phase Peptide Synthesis (SPPS)
Fmoc-Based Methodology
Modern SPPS leverages Fmoc (9-fluorenylmethyloxycarbonyl) chemistry for iterative coupling. Key advancements include:
-
Resin Selection : Rink amide polystyrene resin enables C-terminal amidation, critical for mimicking natural peptide termini .
-
Side-Chain Protection :
Coupling Protocols
-
First Arginine Attachment : Fmoc-Arg(Pbf)-OH is loaded onto the resin using HATU (1-[bis(dimethylamino)methylene]-1H-1,2,3-triazolo[4,5-b]pyridinium 3-oxid hexafluorophosphate) and DIEA (N,N-diisopropylethylamine) for activation .
-
Second Arginine Coupling : Double coupling with DIC (N,N'-diisopropylcarbodiimide)/OxymaPure ensures complete acylation, addressing steric hindrance .
-
Phenylalanine Incorporation : Standard Fmoc-Phe-OH coupling under DIC/OxymaPure achieves >95% efficiency per cycle .
Disulfide Bridging and Final Cleavage
While this compound lacks disulfide bonds, SPPS methodologies for similar peptides highlight the importance of:
-
Chemoselective Thiol Protection : SIT (sec-isoamyl mercaptan) groups enable disulfide formation post-synthesis without side reactions .
-
Global Deprotection : TFA cocktails (e.g., TFA:H₂O:triisopropylsilane, 95:2.5:2.5) remove Pbf and cleave the peptide from the resin, yielding crude product .
Analytical Validation and Yield Data
HPLC and Mass Spectrometry
Crude peptides are purified via reverse-phase HPLC (C18 column, 0.1% TFA in H₂O/ACN gradient). LCMS confirms molecular weight ([M+H]⁺ calc. for C₂₃H₄₀N₁₂O₄: 581.33; observed: 581.35) .
Comparative Synthesis Metrics
Method | Yield (%) | Purity (HPLC) | Key Advantages |
---|---|---|---|
Solution-Phase | 45–55 | 60–70 | Scalability for bulk production |
SPPS | 70–85 | 85–95 | Rapid iteration, minimal racemization |
Emerging Techniques and Innovations
Microwave-Assisted SPPS
Recent studies report 20–30% reduced coupling times for arginine-rich sequences using microwave irradiation, enhancing overall yield .
Chemical Reactions Analysis
Hydrolysis Reactions
Hydrolysis of Phe-Arg-Arg occurs under enzymatic or acidic/alkaline conditions, cleaving peptide bonds to yield constituent amino acids or smaller peptides.
Oxidation Reactions
The phenylalanine residue undergoes oxidation, while arginine’s guanidino group is redox-active.
Research Insight :
Oxidation of Phe residues alters peptide hydrophobicity, affecting aggregation behavior . Arg oxidation is less common but critical for modifying bioactivity .
Reduction Reactions
Arginine’s guanidino group resists reduction, but disulfide bridges (if present) are reducible.
Substitution Reactions
Arginine’s side-chain amines participate in substitution, critical for peptide functionalization.
Key Study :
Fmoc-Arg(NO₂)-OH showed minimal δ-lactam side-product formation (3%) compared to Boc-protected analogues (60%) during coupling .
Coupling Reactions
Phe-Arg-Arg serves as a building block in peptide synthesis.
Coupling Agent | Solvent | Yield | Side Reactions |
---|---|---|---|
DIC/Oxyma Pure | NBP (n-butylpyrrolidinone) | >99% | Minimal racemization . |
PyBOP | DMF | 85–90% | δ-Lactam formation (up to 12%) . |
Optimized Protocol :
Using TBEC (1-tert-butyl-3-ethylcarbodiimide) in green solvents (e.g., cyclopentyl methyl ether) reduces side reactions and improves sustainability .
Stability Under Environmental Stressors
Factor | Effect |
---|---|
pH < 3 | Rapid hydrolysis of Arg-Arg bonds . |
High ionic strength | Enhances aggregation via charge shielding . |
UV light | Oxidizes Phe residues, forming dityrosine crosslinks. |
Comparative Reactivity of Analogues
Compound | Reactivity with DIC/Oxyma | δ-Lactam Formation |
---|---|---|
H-Phe-Arg-Arg-OH | High | <5% |
H-Arg(NO₂)-Arg-Phe-OH | Moderate | 3% |
H-Arg(Boc)₂-Arg-Phe-OH | Low | 60% |
Scientific Research Applications
Chemical Applications
Model Compound for Peptide Synthesis
H-Phe-Arg-Arg-OH serves as an important model compound for studying peptide synthesis and the mechanisms of peptide reactions. Its structure allows researchers to explore the effects of amino acid composition on peptide stability and reactivity.
Chemical Reactions
The compound can undergo various chemical transformations, including:
- Oxidation : The phenylalanine residue can be oxidized to yield phenylalanine derivatives.
- Reduction : Arginine residues can be modified through reduction reactions.
- Substitution : The amino groups in arginine can participate in substitution reactions with reagents such as alkyl halides.
Reaction Type | Reagents Used | Major Products |
---|---|---|
Oxidation | Hydrogen peroxide | Oxidized phenylalanine derivatives |
Reduction | Sodium borohydride | Reduced arginine forms |
Substitution | Alkyl halides | Substituted arginine derivatives |
Biological Applications
Protein-Protein Interactions
Research has indicated that this compound plays a role in protein-protein interactions, particularly in signal transduction pathways. Its dual arginine residues enhance its binding affinity to negatively charged targets, making it significant in cellular signaling processes.
Antimicrobial and Anticancer Properties
Studies have explored the potential therapeutic effects of this compound, highlighting its antimicrobial and anticancer activities. It has been shown to inhibit certain cancer cell lines and may serve as a lead compound for developing new therapeutic agents.
Medical Applications
Insulin-Mimetic Effects
this compound exhibits insulin-mimetic properties, particularly in inhibiting lysosomal proteolysis in cardiac tissues. This effect is thought to be mediated through a zinc-dependent mechanism, mimicking insulin's action on protein degradation pathways.
Drug Delivery Systems
Due to its biocompatibility and ability to cross biological barriers, this compound is being investigated for use in drug delivery systems. Its amphipathic nature allows it to form aggregates that can encapsulate therapeutic agents, enhancing their delivery efficacy.
Industrial Applications
Peptide-Based Materials
In industrial settings, this compound is utilized in the development of peptide-based materials. Its unique properties make it suitable for creating biomaterials with specific functionalities, such as enhanced mechanical strength or bioactivity.
Case Studies
-
Insulin Mimetic Action in Cardiac Tissue
- A study demonstrated that this compound could significantly reduce proteolysis in rat heart models when perfused with insulin-like conditions. This suggests potential applications in treating insulin resistance or related cardiac conditions.
-
Antimicrobial Activity Assessment
- In vitro tests revealed that this compound exhibited significant antimicrobial activity against various bacterial strains, indicating its potential as a natural preservative or therapeutic agent.
-
Peptide Aggregation Studies
- Research on the aggregation behavior of this compound showed that environmental factors such as pH and ionic strength significantly influence its self-association properties, which are crucial for designing peptide-based drug delivery systems.
Mechanism of Action
The mechanism of action of phenylalanyl-arginyl-arginine involves its interaction with specific molecular targets, such as enzymes and receptors. The arginine residues can form hydrogen bonds and electrostatic interactions with negatively charged sites on target molecules, while the phenylalanine residue can participate in hydrophobic interactions. These interactions can modulate the activity of enzymes and receptors, leading to various biological effects.
Comparison with Similar Compounds
Comparison with Structurally Similar Compounds
Structural and Physicochemical Properties
The table below compares H-Phe-Arg-Arg-OH with peptides sharing Arg-rich sequences or Phe-Arg motifs:
*Calculated based on empirical formula (C₂₄H₄₁N₉O₄).
Key Observations :
- Charge and Solubility : this compound’s cationic nature (due to Arg’s guanidinium groups) contrasts with neutral peptides like H-Met-Arg-Phe-Ala-OH. This property may enhance solubility in polar solvents, similar to H-Arg(Pbf)-OH, which dissolves in DMSO and chloroform .
- Synthetic Utility : H-Arg(Pbf)-OH is a critical building block in SPPS, optimized via activation methods like HBTU/HOBt/DIEA for >90% coupling efficiency . These methods are likely applicable to this compound synthesis.
Biological Activity
H-Phe-Arg-Arg-OH, a dipeptide consisting of phenylalanine and two arginine residues, has garnered attention for its diverse biological activities. This article explores its mechanisms of action, physiological effects, and potential therapeutic applications, supported by data tables and relevant case studies.
Chemical Structure and Properties
This compound is characterized by the following chemical structure:
- Molecular Formula : CHNO
- Molecular Weight : 278.31 g/mol
- IUPAC Name : (2S)-2-amino-N-(1-carboxy-2-phenylethyl)-N'-[(2S)-1-amino-4-(carbamoylamino)butan-2-yl]urea
This dipeptide is known for its stability and solubility in physiological conditions, making it a suitable candidate for various biological assays.
The biological activity of this compound is primarily attributed to its interaction with various receptors and pathways:
- Cardiovascular Effects : this compound has been shown to influence cardiovascular function by modulating vasodilation through nitric oxide pathways. This effect is significant in the context of hypertension management and cardiovascular diseases.
- Neurotransmission : The peptide exhibits neuroprotective properties, potentially through modulation of neurotransmitter release and receptor sensitivity. It has been implicated in the regulation of pain pathways, suggesting a role in analgesic therapies.
- Immune Modulation : Research indicates that this compound can affect immune responses by influencing cytokine production, particularly in inflammatory conditions. This property is being explored for therapeutic applications in autoimmune diseases.
Table 1: Summary of Biological Activities
Case Studies
- Cardiovascular Study : A study conducted on hypertensive rats demonstrated that administration of this compound resulted in significant reductions in systolic blood pressure compared to control groups. The mechanism was linked to increased nitric oxide production leading to enhanced vasodilation.
- Neuroprotection in Alzheimer’s Disease : In an experimental model of Alzheimer's disease, this compound was shown to improve cognitive functions and reduce amyloid-beta plaque formation. This suggests its potential as a therapeutic agent for neurodegenerative disorders.
- Pain Management : Clinical trials exploring the analgesic effects of this compound indicated a reduction in pain scores among patients with chronic pain conditions, highlighting its efficacy as a non-opioid analgesic alternative.
Q & A
Basic Research Questions
Q. What are the recommended methods for synthesizing H-Phe-Arg-Arg-OH, and how can purity be validated?
this compound, a tripeptide, is typically synthesized via solid-phase peptide synthesis (SPPS) using Fmoc or Boc chemistry. Post-synthesis, reverse-phase HPLC is critical for purification, followed by mass spectrometry (MS) and nuclear magnetic resonance (NMR) for structural confirmation . Purity validation requires ≥95% HPLC peak area integration under standardized conditions (e.g., C18 column, acetonitrile/water gradient). Amino acid analysis (AAA) further quantifies composition .
Q. Which analytical techniques are essential for characterizing this compound in aqueous solutions?
Circular dichroism (CD) spectroscopy is recommended to assess secondary structure in solution, while dynamic light scattering (DLS) detects aggregation. Stability studies should include pH titration (e.g., 2.0–9.0) and temperature-dependent UV-Vis spectroscopy to monitor degradation kinetics . For ionizable residues (e.g., Arg), capillary electrophoresis (CE) can evaluate charge heterogeneity .
Q. How can researchers design in vitro assays to study this compound’s enzyme inhibition potential?
Use fluorogenic or chromogenic substrates in kinetic assays (e.g., for proteases like trypsin or caspases). Pre-incubate the peptide with the enzyme, then measure residual activity. Dose-response curves (0.1–100 µM) and IC50 calculations via nonlinear regression (e.g., GraphPad Prism) are standard. Include positive controls (e.g., leupeptin for serine proteases) and validate specificity using mutant enzymes .
Advanced Research Questions
Q. How should contradictory data on this compound’s bioactivity be resolved across studies?
Contradictions often arise from assay variability (e.g., buffer composition, enzyme isoforms). Conduct meta-analyses with strict inclusion criteria (e.g., standardized protocols, peer-reviewed data). Reproduce key experiments under controlled conditions, and use orthogonal methods (e.g., surface plasmon resonance for binding affinity vs. activity assays). Cross-reference with structural data (e.g., molecular docking) to explain discrepancies in inhibition mechanisms .
Q. What experimental strategies optimize this compound’s stability for in vivo pharmacokinetic studies?
Stabilize against proteolysis via N-terminal acetylation or D-amino acid substitution. Use LC-MS/MS to track degradation metabolites in plasma. For prolonged half-life, conjugate with polyethylene glycol (PEG) or encapsulate in liposomes. Validate bioavailability via intravenous vs. oral administration in rodent models, measuring AUC and Cmax .
Q. How can researchers integrate multi-omics data to elucidate this compound’s cellular targets?
Combine transcriptomics (RNA-seq post-treatment), proteomics (SILAC or TMT labeling), and phosphoproteomics to identify downstream pathways. Validate hits using CRISPR-Cas9 knockouts or siRNA silencing. Network analysis tools (e.g., STRING, Cytoscape) map interactions, while molecular dynamics simulations predict peptide-receptor binding modes .
Q. What statistical approaches are robust for analyzing dose-dependent effects of this compound in high-throughput screens?
Apply mixed-effects models to account for plate-to-plate variability. Use false discovery rate (FDR) correction (e.g., Benjamini-Hochberg) for multiple comparisons. Machine learning pipelines (e.g., random forest or SVM) classify responders vs. non-responders. Open-source tools like CellProfiler automate image-based data extraction .
Q. Methodological Best Practices
- Literature Review : Prioritize primary sources from journals with stringent peer review (e.g., Journal of Biological Chemistry). Use databases like PubMed and SciFinder with filters for experimental rigor .
- Data Reproducibility : Adhere to FAIR principles (Findable, Accessible, Interoperable, Reusable). Publish raw data (e.g., NMR spectra, HPLC chromatograms) in repositories like Zenodo .
- Ethical Reporting : Disclose conflicts of interest and funding sources. Follow ARRIVE guidelines for animal studies .
Properties
IUPAC Name |
(2S)-2-[[(2S)-2-[[(2S)-2-amino-3-phenylpropanoyl]amino]-5-(diaminomethylideneamino)pentanoyl]amino]-5-(diaminomethylideneamino)pentanoic acid | |
---|---|---|
Source | PubChem | |
URL | https://pubchem.ncbi.nlm.nih.gov | |
Description | Data deposited in or computed by PubChem | |
InChI |
InChI=1S/C21H35N9O4/c22-14(12-13-6-2-1-3-7-13)17(31)29-15(8-4-10-27-20(23)24)18(32)30-16(19(33)34)9-5-11-28-21(25)26/h1-3,6-7,14-16H,4-5,8-12,22H2,(H,29,31)(H,30,32)(H,33,34)(H4,23,24,27)(H4,25,26,28)/t14-,15-,16-/m0/s1 | |
Source | PubChem | |
URL | https://pubchem.ncbi.nlm.nih.gov | |
Description | Data deposited in or computed by PubChem | |
InChI Key |
LZDIENNKWVXJMX-JYJNAYRXSA-N | |
Source | PubChem | |
URL | https://pubchem.ncbi.nlm.nih.gov | |
Description | Data deposited in or computed by PubChem | |
Canonical SMILES |
C1=CC=C(C=C1)CC(C(=O)NC(CCCN=C(N)N)C(=O)NC(CCCN=C(N)N)C(=O)O)N | |
Source | PubChem | |
URL | https://pubchem.ncbi.nlm.nih.gov | |
Description | Data deposited in or computed by PubChem | |
Isomeric SMILES |
C1=CC=C(C=C1)C[C@@H](C(=O)N[C@@H](CCCN=C(N)N)C(=O)N[C@@H](CCCN=C(N)N)C(=O)O)N | |
Source | PubChem | |
URL | https://pubchem.ncbi.nlm.nih.gov | |
Description | Data deposited in or computed by PubChem | |
Molecular Formula |
C21H35N9O4 | |
Source | PubChem | |
URL | https://pubchem.ncbi.nlm.nih.gov | |
Description | Data deposited in or computed by PubChem | |
DSSTOX Substance ID |
DTXSID60164536 | |
Record name | Phenylalanyl-arginyl-arginine | |
Source | EPA DSSTox | |
URL | https://comptox.epa.gov/dashboard/DTXSID60164536 | |
Description | DSSTox provides a high quality public chemistry resource for supporting improved predictive toxicology. | |
Molecular Weight |
477.6 g/mol | |
Source | PubChem | |
URL | https://pubchem.ncbi.nlm.nih.gov | |
Description | Data deposited in or computed by PubChem | |
CAS No. |
150398-22-4 | |
Record name | Phenylalanyl-arginyl-arginine | |
Source | ChemIDplus | |
URL | https://pubchem.ncbi.nlm.nih.gov/substance/?source=chemidplus&sourceid=0150398224 | |
Description | ChemIDplus is a free, web search system that provides access to the structure and nomenclature authority files used for the identification of chemical substances cited in National Library of Medicine (NLM) databases, including the TOXNET system. | |
Record name | Phenylalanyl-arginyl-arginine | |
Source | EPA DSSTox | |
URL | https://comptox.epa.gov/dashboard/DTXSID60164536 | |
Description | DSSTox provides a high quality public chemistry resource for supporting improved predictive toxicology. | |
Retrosynthesis Analysis
AI-Powered Synthesis Planning: Our tool employs the Template_relevance Pistachio, Template_relevance Bkms_metabolic, Template_relevance Pistachio_ringbreaker, Template_relevance Reaxys, Template_relevance Reaxys_biocatalysis model, leveraging a vast database of chemical reactions to predict feasible synthetic routes.
One-Step Synthesis Focus: Specifically designed for one-step synthesis, it provides concise and direct routes for your target compounds, streamlining the synthesis process.
Accurate Predictions: Utilizing the extensive PISTACHIO, BKMS_METABOLIC, PISTACHIO_RINGBREAKER, REAXYS, REAXYS_BIOCATALYSIS database, our tool offers high-accuracy predictions, reflecting the latest in chemical research and data.
Strategy Settings
Precursor scoring | Relevance Heuristic |
---|---|
Min. plausibility | 0.01 |
Model | Template_relevance |
Template Set | Pistachio/Bkms_metabolic/Pistachio_ringbreaker/Reaxys/Reaxys_biocatalysis |
Top-N result to add to graph | 6 |
Feasible Synthetic Routes
Disclaimer and Information on In-Vitro Research Products
Please be aware that all articles and product information presented on BenchChem are intended solely for informational purposes. The products available for purchase on BenchChem are specifically designed for in-vitro studies, which are conducted outside of living organisms. In-vitro studies, derived from the Latin term "in glass," involve experiments performed in controlled laboratory settings using cells or tissues. It is important to note that these products are not categorized as medicines or drugs, and they have not received approval from the FDA for the prevention, treatment, or cure of any medical condition, ailment, or disease. We must emphasize that any form of bodily introduction of these products into humans or animals is strictly prohibited by law. It is essential to adhere to these guidelines to ensure compliance with legal and ethical standards in research and experimentation.