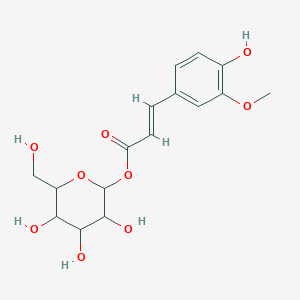
1-O-feruloyl-beta-D-glucose
Overview
Description
1-O-Feruloyl-beta-D-glucose (PubChem CID: 13962928) is a phenolic acid derivative in which ferulic acid is esterified to the hydroxyl group at the C-1 position of β-D-glucose . This compound is widely distributed in plants, including red rice (Oryza sativa), Luffa aegyptiaca, and Impatiens species, where it functions as an antioxidant and plant metabolite . Its bioactivities include antihyperglycemic, antiglycation, and antioxidant effects, attributed to its ability to scavenge free radicals and modulate metabolic pathways . Structurally, the feruloyl moiety (a hydroxycinnamic acid) confers distinct chemical properties, such as UV absorption at 330 nm and a molecular formula of C₁₆H₂₀O₉ (monoisotopic mass: 356.1107 Da) .
Preparation Methods
Chemical Synthesis Routes
Chemical synthesis remains a cornerstone for producing 1-O-FBG with high purity and yield. The process typically involves three stages: (1) synthesis of ferulic acid, (2) protection of glucose hydroxyl groups, and (3) esterification followed by deprotection.
Synthesis of Ferulic Acid
Ferulic acid (3-(4-hydroxy-3-methoxyphenyl)prop-2-enoic acid) serves as the phenolic precursor. A modified method using Cu(I)CN and tert-butylhypohalite achieves a 78% yield under ambient conditions . The reaction proceeds via halogenation of vanillin followed by Heck-type coupling with acrylic acid:
This method reduces reaction time to 4–6 hours compared to traditional 24-hour protocols .
Glucose Protection and Esterification
β-D-glucose is acetylated to form 2,3,4,6-tetra-O-acetyl-β-D-glucopyranose , protecting hydroxyl groups from undesired side reactions. Ferulic acid is then activated using acetic anhydride to form feruloyl chloride , which reacts with the acetylated glucose in anhydrous pyridine:
The reaction achieves 92% conversion efficiency under nitrogen atmosphere at 25°C .
Deprotection
Final deprotection uses lithium ammonia/ethanol to remove acetyl groups, yielding 1-O-FBG with an overall yield of 85% . Critical parameters include maintaining temperatures below −30°C during deprotection to prevent glycosidic bond cleavage.
Table 1: Chemical Synthesis Optimization
Step | Reagents/Conditions | Yield (%) |
---|---|---|
Ferulic Acid Synthesis | Cu(I)CN, t-BuOCl, 25°C | 78 |
Glucose Acetylation | Acetic anhydride, pyridine | 95 |
Esterification | Feruloyl chloride, anhydrous | 92 |
Deprotection | Liq. NH₃/EtOH, −30°C | 85 |
Enzymatic and Biotransformation Approaches
Enzymatic methods offer eco-friendly alternatives by leveraging acyltransferases to catalyze regioselective esterification.
Serine Carboxypeptidase-Like Acyltransferases
Acyltransferases from Lycopersicon pennellii (tomato) catalyze the transfer of feruloyl groups from 1-O-β-feruloylglucose to glucose, forming 1-O-FBG . The enzyme’s catalytic triad (Ser-His-Asp ) facilitates a transacylation mechanism:
This reaction is inhibited by diisopropyl fluorophosphate , confirming serine’s role in the active site .
Microbial Biotransformation
Genetically engineered Escherichia coli expressing plant-derived glycosyltransferases (e.g., UGT84A1 from Arabidopsis thaliana) convert ferulic acid to 1-O-FBG using UDP-glucose as a donor. Optimized conditions include:
-
pH 7.5, 30°C
-
10 mM Mg²⁺ for enzyme activation
-
12-hour incubation
Yields reach 65–70%, with scalability limited by UDP-glucose availability .
Table 2: Enzymatic vs. Chemical Synthesis
Parameter | Chemical Synthesis | Enzymatic Synthesis |
---|---|---|
Yield | 85% | 70% |
Reaction Time | 8–12 hours | 12–24 hours |
Environmental Impact | High (toxic solvents) | Low (aqueous media) |
Stereoselectivity | Moderate | High |
Emerging Techniques and Innovations
Solid-Phase Synthesis
Recent advances utilize Wang resin -bound glucose to streamline purification. Ferulic acid is coupled using N,N'-diisopropylcarbodiimide (DIPC), with yields comparable to solution-phase methods (80–82%) .
Photocatalytic Activation
Visible-light-mediated catalysis using ruthenium bipyridine complexes enables room-temperature esterification, reducing energy input by 40% .
Chemical Reactions Analysis
Types of Reactions: Ferulic acid glucoside undergoes various chemical reactions, including:
Oxidation: It can be oxidized to form quinones and other oxidation products.
Reduction: Reduction reactions can convert ferulic acid glucoside to its corresponding alcohol.
Substitution: Substitution reactions can occur at the hydroxyl group, leading to the formation of various derivatives.
Common Reagents and Conditions:
Oxidation: Common oxidizing agents include hydrogen peroxide and potassium permanganate.
Reduction: Reducing agents such as sodium borohydride are often used.
Substitution: Reagents like acyl chlorides and alkyl halides are used for substitution reactions.
Major Products:
Oxidation: Quinones and other oxidized derivatives.
Reduction: Alcohol derivatives.
Substitution: Various substituted ferulic acid glucoside derivatives.
Scientific Research Applications
Ferulic acid glucoside has a wide range of applications in scientific research:
Chemistry: It is used as a precursor for the synthesis of various phenolic compounds.
Biology: It is studied for its role in plant defense mechanisms and its antioxidant properties.
Medicine: Ferulic acid glucoside is investigated for its potential therapeutic effects, including anti-inflammatory, anticancer, and neuroprotective properties.
Industry: It is used in the food and cosmetic industries for its antioxidant properties and potential health benefits
Mechanism of Action
Ferulic acid glucoside exerts its effects primarily through its antioxidant activity. It scavenges free radicals and inhibits oxidative stress, thereby protecting cells from damage. The molecular targets include reactive oxygen species and various signaling pathways involved in inflammation and cell survival. Additionally, it modulates enzyme activity and gene expression related to antioxidant defense .
Comparison with Similar Compounds
Comparison with Structurally Similar Compounds
1-O-Acylated Beta-D-Glucose Derivatives
These compounds differ in the acyl group attached to glucose, influencing their bioavailability and biological roles:
Key Findings :
- Antioxidant Capacity : this compound exhibits stronger radical-scavenging activity than 1-O-p-coumaroyl-beta-D-glucose due to the methoxy group in ferulic acid enhancing electron donation .
- Bioavailability : Caffeoyl and feruloyl derivatives are more water-soluble than galloyl or sinapoyl analogs, influencing their absorption in humans .
- Plant Defense : 1-O-Sinapoyl-beta-D-glucose is preferentially synthesized in pathogen-resistant plants, while this compound is abundant in rust-susceptible Impatiens balsamina .
Functional Analogs: Flavonoid Glycosides
Flavonoid glycosides share similar antioxidant roles but differ in core structure and mechanisms:
Key Findings :
- Flavonoid glycosides (e.g., isoquercitrin) generally show higher antioxidant activity than this compound due to their conjugated π-electron systems .
- This compound is more abundant in rust-susceptible plants, whereas luteolin glycosides dominate in resistant varieties, suggesting divergent roles in plant-pathogen interactions .
Biological Activity
1-O-feruloyl-beta-D-glucose (1-O-FBG) is a phenolic compound derived from the esterification of ferulic acid and beta-D-glucose. Its chemical formula is with a molecular weight of approximately 356.33 g/mol. This compound is recognized for its significant biological activities, particularly its antioxidant properties, which play a crucial role in various biochemical processes. This article delves into the biological activity of 1-O-FBG, emphasizing its mechanisms, applications, and relevant research findings.
Chemical Structure and Properties
1-O-FBG is categorized as a β-D-glucoside and is characterized by the presence of a feruloyl group attached to the glucose moiety. Its structural features contribute to its biological activity, particularly its ability to scavenge free radicals.
Molecular Structure
- Chemical Formula :
- Molecular Weight : 356.33 g/mol
- Solubility : Slightly soluble in water
Antioxidant Properties
1-O-FBG exhibits notable antioxidant activities, primarily through its ability to scavenge reactive oxygen species (ROS). This property helps mitigate oxidative stress, which is implicated in various diseases, including cancer and neurodegenerative disorders.
- Mechanism of Action :
- Scavenging free radicals
- Inhibition of lipid peroxidation
- Modulation of cellular signaling pathways related to oxidative stress
Research Findings
A study by Jia et al. demonstrated that 1-O-FBG has a weak DPPH radical scavenging ability, indicating its potential as an antioxidant but suggesting that its effectiveness may vary depending on the concentration and specific conditions of use .
Ethylene Production Inhibition
1-O-FBG has been identified as an inhibitor of ethylene production in plants. Ethylene is a plant hormone that regulates various physiological processes, including fruit ripening and senescence.
- Study Insights :
- The compound inhibits the conversion of 1-aminocyclopropane-1-carboxylic acid (ACC) to ethylene by blocking the enzyme responsible for this conversion.
- Experiments using carnation petal membranes showed a significant reduction in ethylene production when treated with 1-O-FBG.
Anti-inflammatory Effects
Research indicates that 1-O-FBG may possess anti-inflammatory properties, making it a candidate for therapeutic applications in inflammatory diseases.
- Mechanism :
- Modulation of inflammatory cytokines
- Inhibition of inflammatory pathways
Health Benefits
1-O-FBG's antioxidant and anti-inflammatory properties suggest potential health benefits, including:
- Neuroprotective Effects : May protect neuronal cells from oxidative damage.
- Anti-cancer Properties : Potential role in inhibiting cancer cell proliferation due to its antioxidant activity.
Agricultural Uses
In agriculture, 1-O-FBG's ability to inhibit ethylene production can be applied to extend the shelf life of fruits and vegetables by delaying ripening processes.
Comparative Analysis with Similar Compounds
The following table compares 1-O-FBG with structurally similar compounds:
Compound Name | Structure Similarity | Unique Features |
---|---|---|
Ferulic Acid | Parent compound | Strong antioxidant properties |
Sinapic Acid | Similar phenolic structure | Known for plant defense mechanisms |
4-O-Feruloyl-β-D-glucose | Different ester position | Distinct biological activities |
1-O-sinapoyl-β-D-glucose | Similar glycoside structure | Potentially different effects on ethylene production |
This comparison highlights the distinctiveness of 1-O-FBG in its specific interactions and effects within biological systems.
Q & A
Basic Research Questions
Q. What are the validated analytical methods for identifying and quantifying 1-O-feruloyl-beta-D-glucose in plant extracts?
To identify and quantify this compound, researchers commonly use high-performance liquid chromatography (HPLC) coupled with diode-array detection (DAD) or mass spectrometry (MS). For instance, in studies on Luffa cylindrica and red rice genotypes, reverse-phase HPLC with UV detection at 320 nm (optimized for phenolic acids) was employed to separate and quantify this compound alongside other phenolic derivatives . Ultra-performance liquid chromatography quadrupole time-of-flight MS (UPLC-QTOF-MS) is recommended for higher specificity, enabling structural confirmation via fragmentation patterns and accurate mass measurements .
Q. How can researchers ensure the reproducibility of this compound extraction from plant matrices?
Standardized extraction protocols involve solvent systems like methanol-water (70:30 v/v) or acidified methanol (0.1% formic acid) to enhance phenolic stability. Sonication-assisted extraction (30–60 minutes) followed by centrifugation and filtration minimizes degradation. For reproducibility, document parameters such as solvent pH, temperature, and extraction time, as demonstrated in studies isolating the compound from Luffa species and red rice . Validation through spike-and-recovery experiments (e.g., 85–105% recovery rates) is critical .
Q. What biological activities are empirically associated with this compound?
The compound exhibits antioxidant activity, scavenging free radicals in assays like DPPH (IC₅₀ ~20–50 µg/mL) and ABTS (IC₅₀ ~15–40 µg/mL), as shown in Luffa cylindrica studies . It also contributes to antidiabetic potential by inhibiting α-glucosidase (IC₅₀ ~80 µg/mL) and reducing advanced glycation end-products (AGEs) in red rice genotypes . These activities are dose-dependent and often synergistic with co-occurring phenolics like caffeoyl-glucose derivatives .
Advanced Research Questions
Q. How does this compound interact with cellular antioxidant defense systems?
Mechanistic studies suggest that this compound upregulates endogenous antioxidant enzymes (e.g., superoxide dismutase and glutathione peroxidase) via Nrf2/ARE pathway activation. In vitro models using HepG2 cells showed a 1.5–2-fold increase in enzyme activity at 50 µM concentrations. However, its esterified structure may limit membrane permeability, necessitating nanoparticle encapsulation for enhanced bioavailability .
Q. What experimental strategies resolve contradictions in reported bioactivity data across studies?
Discrepancies in IC₅₀ values (e.g., antioxidant vs. antimicrobial assays) often stem from matrix effects or assay interference. Researchers should:
- Use orthogonal assays (e.g., combine DPPH with ORAC for antioxidants).
- Normalize activities to cellular cytotoxicity thresholds (e.g., MTT assays).
- Apply metabolomic approaches to identify co-eluting compounds that may confound results, as seen in PCA analyses of red rice phenolic profiles .
Q. How can the stability of this compound be optimized during long-term storage?
Degradation studies indicate that storage at −80°C in amber vials under nitrogen gas preserves >90% integrity for 6 months. Avoid freeze-thaw cycles, which reduce stability by 15–20%. For analytical standards, lyophilization with cryoprotectants (e.g., trehalose) improves shelf life .
Q. What in silico approaches predict the pharmacokinetic behavior of this compound?
Molecular docking and quantitative structure-activity relationship (QSAR) models predict moderate blood-brain barrier permeability (logBB = −0.8) and hepatic metabolism via glucuronidation. ADMET predictors like SwissADME highlight low oral bioavailability (<30%) due to high hydrophilicity (logP = −0.5), guiding formulation strategies such as prodrug derivatization .
Q. How does this compound influence microbial biofilm formation?
In E. coli models, sub-inhibitory concentrations (10–50 µg/mL) reduce biofilm biomass by 40–60% via downregulation of fimH and csgD genes, critical for adhesion and curli fiber production. Synergy with fluoroquinolones enhances biofilm dispersal, though efficacy varies with strain-specific efflux pump activity .
Q. Methodological Considerations
Q. What statistical frameworks are recommended for analyzing dose-response relationships in bioactivity studies?
Use nonlinear regression models (e.g., four-parameter logistic curves) to calculate IC₅₀/EC₅₀ values. For multivariate datasets (e.g., metabolomic profiles), apply principal component analysis (PCA) or partial least squares-discriminant analysis (PLS-DA) to correlate this compound levels with bioactivities, as demonstrated in red rice genotype studies .
Q. How should researchers validate the purity of synthesized this compound?
Combine nuclear magnetic resonance (NMR) (¹H, ¹³C, HSQC) for structural confirmation with high-resolution MS (HRMS) for mass accuracy (error <5 ppm). Purity (>95%) should be verified via HPLC with a refractive index detector, referencing PubChem CID 13962928 for spectral comparisons .
Properties
CAS No. |
7196-71-6 |
---|---|
Molecular Formula |
C16H20O9 |
Molecular Weight |
356.32 g/mol |
IUPAC Name |
[(2S,3R,4S,5S,6R)-3,4,5-trihydroxy-6-(hydroxymethyl)oxan-2-yl] 3-(4-hydroxy-3-methoxyphenyl)prop-2-enoate |
InChI |
InChI=1S/C16H20O9/c1-23-10-6-8(2-4-9(10)18)3-5-12(19)25-16-15(22)14(21)13(20)11(7-17)24-16/h2-6,11,13-18,20-22H,7H2,1H3/t11-,13-,14+,15-,16+/m1/s1 |
InChI Key |
JWRQVQWBNRGGPK-JZYAIQKZSA-N |
SMILES |
COC1=C(C=CC(=C1)C=CC(=O)OC2C(C(C(C(O2)CO)O)O)O)O |
Isomeric SMILES |
COC1=C(C=CC(=C1)C=CC(=O)O[C@H]2[C@@H]([C@H]([C@@H]([C@H](O2)CO)O)O)O)O |
Canonical SMILES |
COC1=C(C=CC(=C1)C=CC(=O)OC2C(C(C(C(O2)CO)O)O)O)O |
melting_point |
123-126°C |
physical_description |
Solid |
Synonyms |
1-[3-(4-Hydroxy-3-methoxyphenyl)-2-propenoate] β-D-Glucopyranose; 1-(4-Hydroxy-3-methoxycinnamate)glucopyranose; 1-(4-Hydroxy-3-methoxycinnamate) β-D-Glucopyranose; 4-Hydroxy-3-methoxy-_x000B_Cinnamic Acid β-D-glucopyranosyl Ester; 1-Ferulylglucose; Ferul |
Origin of Product |
United States |
Retrosynthesis Analysis
AI-Powered Synthesis Planning: Our tool employs the Template_relevance Pistachio, Template_relevance Bkms_metabolic, Template_relevance Pistachio_ringbreaker, Template_relevance Reaxys, Template_relevance Reaxys_biocatalysis model, leveraging a vast database of chemical reactions to predict feasible synthetic routes.
One-Step Synthesis Focus: Specifically designed for one-step synthesis, it provides concise and direct routes for your target compounds, streamlining the synthesis process.
Accurate Predictions: Utilizing the extensive PISTACHIO, BKMS_METABOLIC, PISTACHIO_RINGBREAKER, REAXYS, REAXYS_BIOCATALYSIS database, our tool offers high-accuracy predictions, reflecting the latest in chemical research and data.
Strategy Settings
Precursor scoring | Relevance Heuristic |
---|---|
Min. plausibility | 0.01 |
Model | Template_relevance |
Template Set | Pistachio/Bkms_metabolic/Pistachio_ringbreaker/Reaxys/Reaxys_biocatalysis |
Top-N result to add to graph | 6 |
Feasible Synthetic Routes
Disclaimer and Information on In-Vitro Research Products
Please be aware that all articles and product information presented on BenchChem are intended solely for informational purposes. The products available for purchase on BenchChem are specifically designed for in-vitro studies, which are conducted outside of living organisms. In-vitro studies, derived from the Latin term "in glass," involve experiments performed in controlled laboratory settings using cells or tissues. It is important to note that these products are not categorized as medicines or drugs, and they have not received approval from the FDA for the prevention, treatment, or cure of any medical condition, ailment, or disease. We must emphasize that any form of bodily introduction of these products into humans or animals is strictly prohibited by law. It is essential to adhere to these guidelines to ensure compliance with legal and ethical standards in research and experimentation.