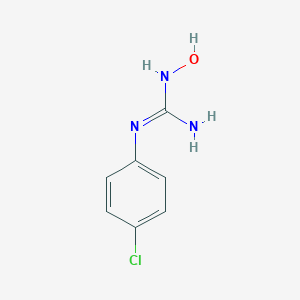
N-(Chlorophenyl)-N'-hydroxyguanidine
- Click on QUICK INQUIRY to receive a quote from our team of experts.
- With the quality product at a COMPETITIVE price, you can focus more on your research.
Overview
Description
N-(Chlorophenyl)-N'-hydroxyguanidine, also known as CHG, is a chemical compound that has gained significant attention in scientific research due to its potential applications in various fields. CHG is a potent inhibitor of nitric oxide synthase (NOS), an enzyme that plays a crucial role in the production of nitric oxide (NO), a signaling molecule involved in various physiological processes.
Mechanism Of Action
N-(Chlorophenyl)-N'-hydroxyguanidine exerts its effects by inhibiting NOS, an enzyme that catalyzes the conversion of L-arginine to NO. By inhibiting NOS, N-(Chlorophenyl)-N'-hydroxyguanidine reduces the production of NO, which is involved in various physiological processes, including vasodilation, neurotransmission, and immune function.
Biochemical And Physiological Effects
N-(Chlorophenyl)-N'-hydroxyguanidine has been shown to have a range of biochemical and physiological effects, including reducing inflammation, improving endothelial function, and inhibiting cancer cell growth. N-(Chlorophenyl)-N'-hydroxyguanidine has also been found to reduce oxidative stress and improve mitochondrial function in animal models of neurodegenerative diseases.
Advantages And Limitations For Lab Experiments
One of the advantages of using N-(Chlorophenyl)-N'-hydroxyguanidine in lab experiments is its potent inhibition of NOS, which allows for the precise modulation of NO levels in cells and tissues. However, N-(Chlorophenyl)-N'-hydroxyguanidine can also have off-target effects, and its use may require careful optimization to avoid unwanted effects on other cellular processes.
Future Directions
There are several future directions for N-(Chlorophenyl)-N'-hydroxyguanidine research, including exploring its potential as a therapeutic agent for various diseases, investigating its effects on different cell types and tissues, and developing more selective NOS inhibitors based on the structure of N-(Chlorophenyl)-N'-hydroxyguanidine. Additionally, further research is needed to elucidate the molecular mechanisms underlying N-(Chlorophenyl)-N'-hydroxyguanidine's effects and to optimize its use in lab experiments and clinical settings.
In conclusion, N-(Chlorophenyl)-N'-hydroxyguanidine is a promising compound with potential applications in various fields of scientific research. Its potent inhibition of NOS and its range of biochemical and physiological effects make it a valuable tool for investigating the role of NO in various physiological processes and diseases. Further research is needed to fully understand the potential of N-(Chlorophenyl)-N'-hydroxyguanidine and to develop more selective NOS inhibitors based on its structure.
Synthesis Methods
N-(Chlorophenyl)-N'-hydroxyguanidine can be synthesized through a multistep process involving the reaction of chlorobenzene with hydroxylamine hydrochloride to form N-(chlorophenyl) hydroxylamine, which is then reacted with cyanamide to produce N-(chlorophenyl) guanidine. Finally, the addition of hydrogen peroxide to N-(chlorophenyl) guanidine yields N-(Chlorophenyl)-N'-hydroxyguanidine.
Scientific Research Applications
N-(Chlorophenyl)-N'-hydroxyguanidine has been extensively studied for its potential applications in various fields, including neuroscience, cardiovascular disease, and cancer research. In neuroscience, N-(Chlorophenyl)-N'-hydroxyguanidine has been shown to improve cognitive function and reduce neuroinflammation in animal models of Alzheimer's disease and traumatic brain injury. In cardiovascular disease, N-(Chlorophenyl)-N'-hydroxyguanidine has been found to reduce blood pressure and improve endothelial function in animal models of hypertension. In cancer research, N-(Chlorophenyl)-N'-hydroxyguanidine has been shown to inhibit the growth and proliferation of various cancer cells, including breast, prostate, and lung cancer cells.
properties
CAS RN |
130974-86-6 |
---|---|
Product Name |
N-(Chlorophenyl)-N'-hydroxyguanidine |
Molecular Formula |
C7H8ClN3O |
Molecular Weight |
185.61 g/mol |
IUPAC Name |
2-(4-chlorophenyl)-1-hydroxyguanidine |
InChI |
InChI=1S/C7H8ClN3O/c8-5-1-3-6(4-2-5)10-7(9)11-12/h1-4,12H,(H3,9,10,11) |
InChI Key |
JYBXKTLYOMPMQY-UHFFFAOYSA-N |
SMILES |
C1=CC(=CC=C1N=C(N)NO)Cl |
Canonical SMILES |
C1=CC(=CC=C1N=C(N)NO)Cl |
Other CAS RN |
130974-86-6 |
synonyms |
2-(4-chlorophenyl)-1-hydroxy-guanidine |
Origin of Product |
United States |
Disclaimer and Information on In-Vitro Research Products
Please be aware that all articles and product information presented on BenchChem are intended solely for informational purposes. The products available for purchase on BenchChem are specifically designed for in-vitro studies, which are conducted outside of living organisms. In-vitro studies, derived from the Latin term "in glass," involve experiments performed in controlled laboratory settings using cells or tissues. It is important to note that these products are not categorized as medicines or drugs, and they have not received approval from the FDA for the prevention, treatment, or cure of any medical condition, ailment, or disease. We must emphasize that any form of bodily introduction of these products into humans or animals is strictly prohibited by law. It is essential to adhere to these guidelines to ensure compliance with legal and ethical standards in research and experimentation.