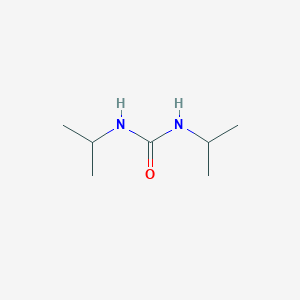
1,3-Diisopropylurea
Overview
Description
1,3-Diisopropylurea (CAS: 4128-37-4) is a urea derivative with the molecular formula C₇H₁₆N₂O and a molecular weight of 144.21 g/mol . Its structure features two isopropyl groups attached to the urea backbone, as depicted by the SMILES notation CC(C)NC(=O)NC(C)C . This compound is utilized in biochemical research to study glycolysis, gluconeogenesis, and lipid metabolism inhibition . Additionally, it is a degradation product of the herbicide Bromacil and a genotoxic impurity in active pharmaceutical ingredients (APIs), necessitating stringent removal during drug manufacturing .
Preparation Methods
Laboratory-Scale Synthesis Methods
Isocyanate-Amine Coupling
The reaction of isopropyl isocyanate with isopropylamine in tetrahydrofuran (THF) using sodium hydride (NaH) as a base represents a high-yield route. This method proceeds via nucleophilic attack of the deprotonated amine on the electrophilic carbon of the isocyanate, forming the urea bond .
Procedure :
-
Dissolve 1 mmol isopropylamine in 5 mL THF.
-
Add 1 mmol NaH and stir for 5 minutes.
-
Introduce 1 mmol isopropyl isocyanate dropwise.
-
Monitor reaction completion via TLC (hexane/ethyl acetate, 3:1).
-
Quench with ice water, extract with ethyl acetate, and purify via column chromatography.
Outcome :
-
Purity : >95% (confirmed by NMR)
-
Advantages : Mild conditions (room temperature), short reaction time (<24 hours).
Urea-Amine Condensation
Adapting methodologies from thiourea synthesis , this route substitutes thiourea with urea, leveraging similar catalytic systems. While urea’s lower reactivity necessitates elevated temperatures, PEG-400 enhances reaction efficiency by stabilizing intermediates.
Procedure :
-
Combine urea and diisopropylamine in a 0.5:1 molar ratio.
-
Add PEG-400 (4% molar equivalent of urea) in water.
-
Reflux at 100°C for 24–30 hours.
-
Filter and wash the precipitate with cold water.
Outcome :
-
Challenges : Requires rigorous temperature control to prevent urea decomposition.
Industrial Production Techniques
Catalytic Methods Using PEG-400
Industrial-scale synthesis prioritizes cost-effectiveness and environmental sustainability. The PEG-400-catalyzed reaction in water achieves these goals by enabling catalyst and solvent recycling .
Optimized Parameters :
-
Molar Ratio : Urea:diisopropylamine = 0.5:1
-
Catalyst Loading : 4% PEG-400 (relative to urea)
-
Temperature : 100°C (reflux)
-
Time : 24–30 hours
Economic Impact :
-
Waste Reduction : Aqueous solvent minimizes volatile organic compound (VOC) emissions.
Solvent and Catalyst Recycling
Post-reaction filtrates from the PEG-400 system retain catalytic activity, allowing reuse without significant yield loss. For example, recycling the filtrate in subsequent batches maintained yields at 78–82% over three cycles .
Reaction Optimization and Parameters
Temperature and Time Effects
Elevated temperatures (80–100°C) are critical for overcoming urea’s kinetic barriers, while prolonged reaction times (>24 hours) ensure complete conversion.
Table 1: Temperature vs. Yield
Temperature (°C) | Time (hours) | Yield (%) |
---|---|---|
80 | 36 | 65 |
100 | 24 | 80 |
120 | 12 | 72* |
*Decomposition byproducts observed at >110°C.
Catalysts and Their Impact
PEG-400 outperforms traditional bases (e.g., NaOH) by reducing side reactions and enhancing solubility.
Table 2: Catalyst Performance Comparison
Catalyst | Yield (%) | Byproducts (%) |
---|---|---|
PEG-400 | 80 | <5 |
NaOH | 60 | 15–20 |
None | 30 | 30–40 |
Comparative Analysis of Synthesis Routes
Table 3: Method Comparison
Method | Yield (%) | Scalability | Environmental Impact |
---|---|---|---|
Isocyanate-Amine | 88 | Moderate | Medium (THF solvent) |
PEG-400 Catalyzed | 80 | High | Low (aqueous) |
Carbodiimide Hydrolysis | 65* | Low | High (multi-step) |
*Theoretical yield based on carbodiimide intermediate synthesis .
Chemical Reactions Analysis
Hydrolysis
The urea bond undergoes hydrolysis under acidic or alkaline conditions:
Acidic Hydrolysis (HCl, 80°C):
Alkaline Hydrolysis (NaOH, 60°C):
Table 1: Hydrolysis Reaction Parameters
Condition | Reagent | Temperature | Time | Yield | Byproducts |
---|---|---|---|---|---|
Acidic | 6M HCl | 80°C | 4 hrs | 85% | Isopropylamine·HCl, CO₂ |
Alkaline | 4M NaOH | 60°C | 3 hrs | 78% | Sodium carbonate |
Methacrylic Acid Complexes
1,3-Diisopropylurea forms 1:1 and 1:2 complexes with methacrylic acid (MAA) via hydrogen bonding, as confirmed by NMR and DFT calculations (B3LYP/6-311++G(d,p)) . The 1:2 complex exhibits a binding constant , critical for designing molecularly imprinted polymers (MIPs) .
Key Interactions:
-
N–H···O Hydrogen Bonds : Between urea NH and MAA carbonyl.
-
Steric Effects : Isopropyl groups direct MAA orientation.
Dynamic Covalent Networks
This compound participates in reversible urea bond exchange with isocyanates, enabling self-healing polymers. The dissociation rate () of its urea bonds at 37°C is 0.21 h⁻¹ , with a half-life () of 3.3 hours .
Table 2: Polymerization Parameters
Halogenation
This compound reacts with halogens (Cl₂, Br₂) under controlled conditions, yielding halogenated derivatives. For example, bromination produces 1-(2-bromo-5-methyl-3-oxocyclohex-1-en-1-yl)-1,3-diisopropylurea with 81% yield .
Condensation with Carbodiimides
In Rh₂(OAc)₄-catalyzed reactions, it forms N-acyl ureas via insertion into carbodiimides :
Genotoxic Impurity Removal
Molecularly imprinted polymers (MIPs) functionalized with methacrylic acid selectively adsorb this compound from API solutions, achieving 80% binding efficiency even at 1:10,000 impurity-to-API ratios .
Scientific Research Applications
Scientific Research Applications
-
Peptide Synthesis
DIPU is widely used as a coupling reagent in solid-phase peptide synthesis (SPPS). It facilitates the formation of peptide bonds while minimizing solvent usage, thus enhancing efficiency and reducing waste by up to 95% during amino acid addition cycles . This method has been demonstrated to maintain high purity and scalability for complex peptides. -
Molecularly Imprinted Polymers (MIPs)
In the pharmaceutical industry, DIPU serves as a template for designing MIPs that selectively bind and remove impurities from active pharmaceutical ingredients (APIs). The use of DIPU in MIPs allows for the targeted extraction of potentially genotoxic impurities from reaction streams . -
Catalysis
DIPU forms complexes with metal ions, which are utilized as catalysts in various organic reactions. These metal complexes enhance reaction rates and selectivity, making them valuable in synthetic chemistry. -
Material Science
The properties of DIPU allow it to be used in developing new materials with unique characteristics. For instance, its ability to form dynamic covalent bonds contributes to self-healing and environmentally adaptive materials . -
Biological Activity
Research indicates that DIPU may exhibit anticancer properties by inhibiting fatty acid synthesis, which is crucial for cell division in cancer cells. This mechanism suggests potential therapeutic applications in treating conditions like prostate cancer .
Peptide Synthesis Efficiency
A study demonstrated that using DIPU in SPPS significantly reduced the time and resources needed for peptide synthesis without compromising quality. The process was scalable for sequences up to 89 amino acids long, showcasing its practical application in pharmaceutical development.
MIPs for API Purification
Research focused on creating MIPs using DIPU as a template showed successful removal of genotoxic impurities from API solutions. The study highlighted the effectiveness of these polymers under industrial conditions, emphasizing their potential for improving drug safety standards .
Dynamic Bonding in Materials
Investigations into polymers containing DIPU revealed their ability to self-heal and adapt to environmental changes. This property was tested through mechanical stress experiments where the polymer exhibited recovery capabilities after being cut, demonstrating its practical applications in smart materials .
Mechanism of Action
The mechanism of action of 1,3-Diisopropylurea involves its ability to form hydrogen bonds and interact with other molecules through its urea moiety. This interaction is crucial in peptide synthesis, where it facilitates the formation of peptide bonds by activating carboxyl groups. In molecularly imprinted polymers, it acts as a template molecule, allowing for the selective binding of target molecules .
Comparison with Similar Compounds
Comparison with Structurally Similar Urea Derivatives
Substituent Effects on Physical Properties
Urea derivatives vary significantly in physical properties based on substituent groups:
*Calculated based on molecular formulas.
- Melting Points : Bulky substituents like cyclohexyl (e.g., 1,3-dicyclohexylurea) increase melting points due to enhanced crystal packing, whereas branched isopropyl groups (e.g., this compound derivatives) result in lower melting points (158–160°C for compound 4i ) .
- Solubility : this compound is soluble in organic solvents, while dicyclohexylurea derivatives exhibit lower solubility due to hydrophobicity .
Genotoxicity and Regulatory Considerations
Removal from Pharmaceuticals
Studies demonstrate that OSN at a diafiltration ratio of 3 achieves 90% removal of this compound from APIs, with MIPs further reducing residual levels to meet regulatory standards .
Biological Activity
1,3-Diisopropylurea (DIPU) is a compound that has garnered interest in various fields, particularly in medicinal chemistry and polymer science. This article explores its biological activity, focusing on its interactions, potential therapeutic applications, and mechanisms of action.
This compound is a derivative of urea, characterized by two isopropyl groups attached to the nitrogen atoms. Its chemical formula is and it has a molecular weight of 142.20 g/mol. The structure can be represented as follows:
- Enzyme Inhibition : DIPU has been shown to act as an inhibitor of certain enzymes, which can be pivotal in drug design. For instance, it interacts with enzymes involved in steroid metabolism, specifically the inhibition of 5α-reductase, which converts testosterone to dihydrotestosterone (DHT). This inhibition is significant in the context of treating conditions like benign prostatic hyperplasia and prostate cancer .
- Host-Guest Interactions : Recent studies have demonstrated that DIPU can form complexes with various molecules through host-guest interactions. For example, its complexation with methacrylic acid has been investigated using NMR and IR spectroscopy. These studies revealed the formation of higher-order complexes that may have implications for drug delivery systems and molecular imprinting technologies .
- Genotoxic Potential : Some research indicates that DIPU may exhibit genotoxic properties under certain conditions. The potential for DNA damage through oxidative stress mechanisms has been noted, suggesting a need for careful evaluation in therapeutic contexts .
Case Study 1: Inhibition of 5α-Reductase
A study investigated the effects of various urea derivatives on 5α-reductase activity. It was found that DIPU significantly inhibited this enzyme, leading to reduced levels of DHT in cellular models. This finding supports its potential use in treating androgen-dependent conditions .
Case Study 2: Molecular Imprinting
In another study focusing on molecular imprinting techniques, DIPU was used as a template molecule to create selective binding sites within polymer matrices. The binding properties were analyzed using computational methods alongside experimental techniques, indicating that DIPU's structural characteristics enhance the specificity of the resulting polymers for target analytes .
Table 1: Summary of Biological Activities of this compound
Q & A
Basic Research Questions
Q. What are the common synthetic pathways leading to the formation of 1,3-Diisopropylurea (DIU) as a by-product?
DIU frequently forms during peptide synthesis when diisopropylcarbodiimide (DIC) is used as a coupling reagent. The reaction between DIC and carboxylic acids generates DIU as a side product, which can persist even after standard aqueous workups and column chromatography. Residual DIU often requires advanced purification methods like preparative HPLC for complete removal .
Q. Which analytical techniques are most effective for detecting and quantifying DIU in reaction mixtures?
Thin-layer chromatography (TLC) and elemental analysis are initial screening methods, but they lack sensitivity for trace DIU detection. High-performance liquid chromatography (HPLC) is preferred for precise quantification, particularly in pharmaceutical impurity profiling. Mass spectrometry (MS) can further confirm DIU identity in complex matrices .
Q. How do solubility properties of DIU influence purification strategies in synthetic workflows?
DIU exhibits poor solubility in polar solvents like water and methanol, complicating traditional liquid-liquid extraction. This necessitates solvent optimization (e.g., using dichloromethane or ethyl acetate) or hybrid approaches like organic solvent nanofiltration (OSN) to isolate DIU from active pharmaceutical ingredients (APIs) .
Advanced Research Questions
Q. What strategies are effective for removing trace DIU impurities from APIs while maintaining yield and purity?
A hybrid method combining molecularly imprinted polymers (MIPs) and OSN has shown success. MIPs tailored for DIU (using methacrylic acid as a functional monomer) selectively bind the impurity, while OSN separates it based on molecular size. This dual approach achieves >99% DIU removal without compromising API integrity .
Q. How does residual DIU impact the safety and efficacy of pharmaceutical compounds?
DIU is classified as a potential genotoxic impurity (GTI) due to structural alerts. Regulatory guidelines (e.g., ICH M7) mandate its concentration to ≤1 ppm in APIs. Validated HPLC-MS methods are critical for ensuring compliance, with detection limits ≤0.1 ppm .
Q. What role does DIU play in the design of molecularly imprinted polymers for pharmaceutical impurity removal?
DIU serves as a template in MIP synthesis, where functional monomers (e.g., methacrylic acid) form hydrogen bonds with its urea group. After polymerization and template removal, the MIPs exhibit high selectivity for DIU, achieving binding capacities up to 5.03 mg/g in optimized conditions .
Q. How can researchers address discrepancies in solubility data for DIU across different solvent systems?
Systematic solubility studies under controlled temperatures and solvent polarities are essential. For instance, DIU’s insolubility in water but moderate solubility in acetonitrile (Table 3, ) informs solvent selection for recrystallization or nanofiltration. Computational models (e.g., COSMO-RS) can predict solubility trends to guide experimental design .
Q. Methodological Considerations
- Experimental Design : Prioritize orthogonal analytical methods (e.g., HPLC + MS) to resolve DIU co-elution with APIs .
- Data Contradictions : Reconcile solubility discrepancies by validating solvent polarity indexes and temperature effects .
- Regulatory Alignment : Align detection limits with ICH M7 guidelines using spike/recovery studies in API matrices .
Properties
IUPAC Name |
1,3-di(propan-2-yl)urea | |
---|---|---|
Source | PubChem | |
URL | https://pubchem.ncbi.nlm.nih.gov | |
Description | Data deposited in or computed by PubChem | |
InChI |
InChI=1S/C7H16N2O/c1-5(2)8-7(10)9-6(3)4/h5-6H,1-4H3,(H2,8,9,10) | |
Source | PubChem | |
URL | https://pubchem.ncbi.nlm.nih.gov | |
Description | Data deposited in or computed by PubChem | |
InChI Key |
BGRWYRAHAFMIBJ-UHFFFAOYSA-N | |
Source | PubChem | |
URL | https://pubchem.ncbi.nlm.nih.gov | |
Description | Data deposited in or computed by PubChem | |
Canonical SMILES |
CC(C)NC(=O)NC(C)C | |
Source | PubChem | |
URL | https://pubchem.ncbi.nlm.nih.gov | |
Description | Data deposited in or computed by PubChem | |
Molecular Formula |
C7H16N2O | |
Source | PubChem | |
URL | https://pubchem.ncbi.nlm.nih.gov | |
Description | Data deposited in or computed by PubChem | |
DSSTOX Substance ID |
DTXSID6044486 | |
Record name | 1,3-Dipropan-2-ylurea | |
Source | EPA DSSTox | |
URL | https://comptox.epa.gov/dashboard/DTXSID6044486 | |
Description | DSSTox provides a high quality public chemistry resource for supporting improved predictive toxicology. | |
Molecular Weight |
144.21 g/mol | |
Source | PubChem | |
URL | https://pubchem.ncbi.nlm.nih.gov | |
Description | Data deposited in or computed by PubChem | |
CAS No. |
4128-37-4 | |
Record name | N,N′-Bis(1-methylethyl)urea | |
Source | CAS Common Chemistry | |
URL | https://commonchemistry.cas.org/detail?cas_rn=4128-37-4 | |
Description | CAS Common Chemistry is an open community resource for accessing chemical information. Nearly 500,000 chemical substances from CAS REGISTRY cover areas of community interest, including common and frequently regulated chemicals, and those relevant to high school and undergraduate chemistry classes. This chemical information, curated by our expert scientists, is provided in alignment with our mission as a division of the American Chemical Society. | |
Explanation | The data from CAS Common Chemistry is provided under a CC-BY-NC 4.0 license, unless otherwise stated. | |
Record name | N,N'-Diisopropylurea | |
Source | ChemIDplus | |
URL | https://pubchem.ncbi.nlm.nih.gov/substance/?source=chemidplus&sourceid=0004128374 | |
Description | ChemIDplus is a free, web search system that provides access to the structure and nomenclature authority files used for the identification of chemical substances cited in National Library of Medicine (NLM) databases, including the TOXNET system. | |
Record name | 1,3-Diisopropylurea | |
Source | DTP/NCI | |
URL | https://dtp.cancer.gov/dtpstandard/servlet/dwindex?searchtype=NSC&outputformat=html&searchlist=112719 | |
Description | The NCI Development Therapeutics Program (DTP) provides services and resources to the academic and private-sector research communities worldwide to facilitate the discovery and development of new cancer therapeutic agents. | |
Explanation | Unless otherwise indicated, all text within NCI products is free of copyright and may be reused without our permission. Credit the National Cancer Institute as the source. | |
Record name | Urea, N,N'-bis(1-methylethyl)- | |
Source | EPA Chemicals under the TSCA | |
URL | https://www.epa.gov/chemicals-under-tsca | |
Description | EPA Chemicals under the Toxic Substances Control Act (TSCA) collection contains information on chemicals and their regulations under TSCA, including non-confidential content from the TSCA Chemical Substance Inventory and Chemical Data Reporting. | |
Record name | 1,3-Dipropan-2-ylurea | |
Source | EPA DSSTox | |
URL | https://comptox.epa.gov/dashboard/DTXSID6044486 | |
Description | DSSTox provides a high quality public chemistry resource for supporting improved predictive toxicology. | |
Record name | 1,3-diisopropylurea | |
Source | European Chemicals Agency (ECHA) | |
URL | https://echa.europa.eu/substance-information/-/substanceinfo/100.021.764 | |
Description | The European Chemicals Agency (ECHA) is an agency of the European Union which is the driving force among regulatory authorities in implementing the EU's groundbreaking chemicals legislation for the benefit of human health and the environment as well as for innovation and competitiveness. | |
Explanation | Use of the information, documents and data from the ECHA website is subject to the terms and conditions of this Legal Notice, and subject to other binding limitations provided for under applicable law, the information, documents and data made available on the ECHA website may be reproduced, distributed and/or used, totally or in part, for non-commercial purposes provided that ECHA is acknowledged as the source: "Source: European Chemicals Agency, http://echa.europa.eu/". Such acknowledgement must be included in each copy of the material. ECHA permits and encourages organisations and individuals to create links to the ECHA website under the following cumulative conditions: Links can only be made to webpages that provide a link to the Legal Notice page. | |
Record name | N,N'-DIISOPROPYLUREA | |
Source | FDA Global Substance Registration System (GSRS) | |
URL | https://gsrs.ncats.nih.gov/ginas/app/beta/substances/KT3MU4Y10Z | |
Description | The FDA Global Substance Registration System (GSRS) enables the efficient and accurate exchange of information on what substances are in regulated products. Instead of relying on names, which vary across regulatory domains, countries, and regions, the GSRS knowledge base makes it possible for substances to be defined by standardized, scientific descriptions. | |
Explanation | Unless otherwise noted, the contents of the FDA website (www.fda.gov), both text and graphics, are not copyrighted. They are in the public domain and may be republished, reprinted and otherwise used freely by anyone without the need to obtain permission from FDA. Credit to the U.S. Food and Drug Administration as the source is appreciated but not required. | |
Synthesis routes and methods I
Procedure details
Synthesis routes and methods II
Procedure details
Synthesis routes and methods III
Procedure details
Synthesis routes and methods IV
Procedure details
Synthesis routes and methods V
Procedure details
Retrosynthesis Analysis
AI-Powered Synthesis Planning: Our tool employs the Template_relevance Pistachio, Template_relevance Bkms_metabolic, Template_relevance Pistachio_ringbreaker, Template_relevance Reaxys, Template_relevance Reaxys_biocatalysis model, leveraging a vast database of chemical reactions to predict feasible synthetic routes.
One-Step Synthesis Focus: Specifically designed for one-step synthesis, it provides concise and direct routes for your target compounds, streamlining the synthesis process.
Accurate Predictions: Utilizing the extensive PISTACHIO, BKMS_METABOLIC, PISTACHIO_RINGBREAKER, REAXYS, REAXYS_BIOCATALYSIS database, our tool offers high-accuracy predictions, reflecting the latest in chemical research and data.
Strategy Settings
Precursor scoring | Relevance Heuristic |
---|---|
Min. plausibility | 0.01 |
Model | Template_relevance |
Template Set | Pistachio/Bkms_metabolic/Pistachio_ringbreaker/Reaxys/Reaxys_biocatalysis |
Top-N result to add to graph | 6 |
Feasible Synthetic Routes
Disclaimer and Information on In-Vitro Research Products
Please be aware that all articles and product information presented on BenchChem are intended solely for informational purposes. The products available for purchase on BenchChem are specifically designed for in-vitro studies, which are conducted outside of living organisms. In-vitro studies, derived from the Latin term "in glass," involve experiments performed in controlled laboratory settings using cells or tissues. It is important to note that these products are not categorized as medicines or drugs, and they have not received approval from the FDA for the prevention, treatment, or cure of any medical condition, ailment, or disease. We must emphasize that any form of bodily introduction of these products into humans or animals is strictly prohibited by law. It is essential to adhere to these guidelines to ensure compliance with legal and ethical standards in research and experimentation.