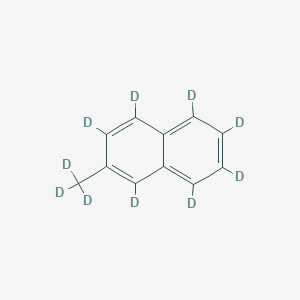
2-Methylnaphthalene-d10
Overview
Description
2-(Trifluoromethyl)quinoxaline is a heterocyclic compound featuring a quinoxaline core substituted with a trifluoromethyl (-CF₃) group at the 2-position. Quinoxalines are nitrogen-containing bicyclic structures known for their diverse pharmacological and material applications . The trifluoromethyl group enhances lipophilicity, metabolic stability, and binding affinity, making this derivative particularly valuable in drug discovery .
Preparation Methods
Direct Deuteration Using Supercritical Deuterium Oxide
Reaction Mechanism and Conditions
The most efficient method for synthesizing 2-methylnaphthalene-d10 involves exposing 2-methylnaphthalene to supercritical DO at temperatures between 200°C and 450°C . Under these conditions, the physicochemical properties of DO—including reduced dielectric constant and enhanced ion product—facilitate rapid hydrogen-deuterium (H/D) exchange. At 450°C, deuteration efficiencies reach 100%, with deuterium preferentially incorporating into aromatic C–H bonds due to increased electrophilic substitution rates .
Catalytic Enhancements
The addition of acid (DCl), base (NaOD), or salt catalysts (NaCO) improves reaction reproducibility and reduces side products. For instance, NaOD-catalyzed reactions yield this compound with a relative standard deviation (RSD) below 5%, minimizing undesired byproducts such as dimethylnaphthalenes . Metal catalysts (e.g., platinum) are less effective, often leading to hydrogenolysis or ring saturation.
Table 1: Supercritical DO Deuteration Performance
Temperature (°C) | Catalyst | Deuteration Efficiency (%) | Byproducts Detected |
---|---|---|---|
450 | None | 100 | Trace naphthalene-d8 |
400 | NaOD (0.1M) | 98 ± 2 | Dimethylnaphthalene (<1%) |
350 | DCl (0.05M) | 85 ± 5 | Undeuterated residue (5%) |
Isomerization of Deuterated 1-Methylnaphthalene
Catalytic Isomerization Framework
1-Methylnaphthalene-d10 can be isomerized to this compound using dealuminated Y zeolites with unit cell constants ≤24.37 Å . Steam-treated Y zeolites (Si/Al ratio >5) exhibit enhanced stability at high temperatures (350–600°C), preventing rapid deactivation from carbonaceous deposits. The reaction proceeds via a carbocation intermediate, where deuterium retention depends on the absence of proton donors in the reaction environment .
Temperature Optimization
At 450°C, the isomerization achieves a 72% yield of this compound with a catalyst lifetime exceeding 100 hours . Lower temperatures (350°C) favor thermodynamic control but reduce reaction rates, while temperatures above 500°C accelerate catalyst degradation.
Table 2: Isomerization Performance with Y Zeolites
Unit Cell Constant (Å) | Temperature (°C) | This compound Yield (%) | Catalyst Lifetime (hr) |
---|---|---|---|
24.29 | 450 | 72 | 120 |
24.37 | 480 | 68 | 90 |
24.45 | 420 | 55 | 60 |
Methylation of Deuterated Naphthalene Precursors
Vapor-Phase Methylation
Naphthalene-d8 reacts with deuterated methanol (CDOD) over activated alumina catalysts at 500–650°C to produce this compound . The reaction mechanism involves Friedel-Crafts alkylation, where the deuterated methyl group (CD) electrophilically substitutes the naphthalene ring. Elevated temperatures (>550°C) favor 2-methyl selectivity due to reduced steric hindrance at the β-position .
Pressure and Feedstock Effects
Operating at 10–40 atmospheres enhances methanol conversion but requires careful control to avoid polyalkylation. A molar ratio of naphthalene-d8 to CDOD of 1:2 optimizes monoalkylation, achieving 50% selectivity for this compound .
Table 3: Methylation Reaction Parameters
Pressure (atm) | Temperature (°C) | Selectivity this compound (%) | Methanol Conversion (%) |
---|---|---|---|
10 | 570 | 50 | 85 |
20 | 600 | 48 | 92 |
40 | 650 | 45 | 95 |
Catalytic H/D Exchange Reactions
Acid- and Base-Mediated Exchange
Deuterium incorporation into pre-synthesized 2-methylnaphthalene can occur via reversible H/D exchange using DCl or NaOD in DO . Acidic conditions (pH <2) protonate the aromatic ring, facilitating electrophilic deuteration, while basic conditions (pH >12) deprotonate C–H bonds, enabling nucleophilic substitution. However, these methods are less efficient than supercritical DO, typically achieving 70–85% deuteration .
Solvent Isotope Effects
The use of fully deuterated solvents (e.g., DO, CDOD) suppresses protium recontamination. Kinetic studies show a ratio of 3.2 for aromatic deuteration, indicating significant isotope effects during exchange .
Comparative Analysis of Preparation Methods
Efficiency and Scalability
Supercritical DO deuteration offers the highest efficiency (100%) but requires specialized high-pressure reactors . Isomerization and methylation routes are more scalable for industrial production but depend on the availability of deuterated precursors .
Cost Considerations
Deuterated reagents (e.g., CDOD, naphthalene-d8) increase synthesis costs by 20–50x compared to non-deuterated analogs. Catalytic isomerization minimizes reagent expenses but demands frequent catalyst regeneration .
Table 4: Method Comparison
Method | Deuterium Source | Yield (%) | Cost (Relative) | Scalability |
---|---|---|---|---|
Supercritical DO | DO | 100 | High | Moderate |
Isomerization | 1-Methylnaphthalene-d10 | 72 | Medium | High |
Methylation | CDOD | 50 | Very High | High |
Chemical Reactions Analysis
Atmospheric OH Radical-Initiated Oxidation
2-Methylnaphthalene-d10 undergoes oxidation via hydroxyl (OH) radical addition, forming deuterated adducts. Key steps include:
-
Adduct Formation : OH radicals add to α- and β-positions of the naphthalene ring, producing intermediates such as 2-MN-n-OH-D10 (n = 1–8) .
-
Fate of Adducts : α-adducts (R1) predominantly react with O₂ to form peroxy radicals, while β-adducts (R3) undergo ring-opening reactions .
Table 1: Key intermediates in OH-initiated oxidation
Intermediate | Pathway | Product Type |
---|---|---|
R1 (α-adduct) | O₂ addition → Peroxy radical | Bicyclic peroxides |
R3 (β-adduct) | Ring cleavage → Epoxides | Carbonyl compounds |
Microbial Oxidation in Anaerobic Environments
Under sulfate-reducing conditions, this compound is mineralized via:
-
Methyl Group Oxidation : The deuterated methyl group (-CD₃) is oxidized to a carboxyl group (-COOH), forming 2-naphthoic acid-d7 (retains seven deuterium atoms on the ring) .
-
Carboxylation : Three carboxylated derivatives of this compound are detected, though their exact structures remain unconfirmed .
Table 2: Biodegradation metrics
Parameter | Value | Conditions |
---|---|---|
Degradation Rate | 25 μM/day | 20 mM sulfate, anaerobic |
Mineralization to CO₂ | 87% | 14C-labeled compound |
Gas-Phase NO₃ Radical Reactions
Nitration occurs via electrophilic attack on the aromatic ring:
-
Primary Products : Dimethylnitronaphthalenes (DMNNs) and ethylnitronaphthalenes (ENNs) form with yields of 4–21% and 12–19%, respectively .
-
Mechanism : NO₃ addition followed by NO₂ substitution and HNO₃ elimination .
Table 3: Nitro-derivative yields
Reactant | Product | Yield (%) |
---|---|---|
This compound | 2-Nitromethylnaphthalene | 19 |
This compound | Dimethylnitronaphthalene | 21 |
Hazardous Interactions
This compound exhibits reactivity with:
-
Strong Oxidizers : Reactions with peroxides/O₂ may produce explosive intermediates .
-
Thermal Decomposition : Forms carbon oxides under high temperatures (>260°C) .
Photochemical Degradation
In atmospheric conditions, deuterated analogs follow pathways similar to non-deuterated forms but with altered kinetics due to isotope effects .
Hydrolysis and Solubility
Scientific Research Applications
Environmental Monitoring and Analysis
Use in PAH Analysis
2-Methylnaphthalene-d10 is primarily utilized as an internal standard in gas chromatography-mass spectrometry (GC-MS) for the analysis of PAHs in environmental samples. Its deuterated form allows for accurate quantification due to its distinct mass, which aids in differentiating it from non-deuterated compounds during analysis. This is particularly important in studies examining the presence of PAHs in soil and water samples.
- Case Study: GC-MS Optimization
A study optimized a GC-MS method using this compound to analyze PAHs across various matrices, achieving high sensitivity and a wide dynamic range (2.5 ppb to 20 ppm). The use of this deuterated compound improved calibration accuracy and reduced errors in quantification, demonstrating its effectiveness as an internal standard in complex environmental samples .
Parameter | Value |
---|---|
Dynamic Range | 2.5 ppb to 20 ppm |
Sensitivity | Low picogram range |
Calibration Method | Single curve for multiple matrices |
Toxicological Research
Toxicity Studies
This compound is also used in toxicological studies to assess the effects of 2-methylnaphthalene on biological systems. Its deuterated form allows researchers to trace metabolic pathways without interference from naturally occurring compounds.
- Case Study: Toxicological Review
A comprehensive review by the U.S. Environmental Protection Agency (EPA) highlighted the use of deuterated compounds like this compound in evaluating chronic toxicity and cancer bioassays. The study provided insights into the metabolic pathways and potential health risks associated with exposure to methylnaphthalenes .
Study Type | Findings |
---|---|
Chronic Toxicity | Identified pulmonary effects in mice |
Metabolism | Traced using deuterated standards |
Chemical Synthesis and Industrial Applications
Synthesis of Derivatives
In chemical synthesis, this compound serves as a precursor for synthesizing various derivatives used in industrial applications, including dyes, fragrances, and pharmaceuticals.
- Case Study: Synthesis Pathways
Research has demonstrated that this compound can be nitrated to produce nitro derivatives with specific yields. This process is essential for developing compounds with desired chemical properties for industrial use .
Synthesis Reaction | Product | Yield (%) |
---|---|---|
Nitration | Nitro-derivatives | Varies (4-21% based on isomer) |
Analytical Chemistry Applications
Standardization in Analytical Methods
The compound is widely used as a standard in analytical chemistry due to its stable isotopic composition, which enhances the reliability of analytical results.
- Case Study: Standardization Protocols
Analytical methods involving solid-phase microextraction (SPME) have employed this compound to standardize measurements of volatile organic compounds (VOCs) in food products. This application underscores its role in ensuring accuracy across various analytical techniques .
Application Area | Method Used | Outcome |
---|---|---|
Food Safety | SPME GC-MS | Accurate VOC profiling |
Mechanism of Action
The mechanism of action of 2-Methylnaphthalene-d10 involves its interaction with molecular targets through various pathways:
Oxidation Mechanism: The compound undergoes oxidation initiated by hydroxyl radicals (OH) in the atmosphere, forming various intermediates and products.
Molecular Targets: The primary targets are the aromatic ring and the methyl group, which undergo various transformations during the reactions.
Comparison with Similar Compounds
Key Properties :
Structure-Activity Relationships (SAR)
- Electronic Effects: The -CF₃ group’s electron-withdrawing nature reduces electron density on the quinoxaline ring, weakening π-stacking with catalytic residues (e.g., His57 in HCV protease) . In contrast, ethyl (-C₂H₅) groups, being electron-neutral, maintain stronger interactions .
Steric Effects :
Pharmacological Performance
- Antimicrobial Activity: 2-Acyl-3-CF₃-quinoxaline 1,4-dioxides show 4–8× higher activity against Mycobacterium tuberculosis than non-fluorinated analogs due to improved membrane penetration . -CF₃ derivatives also inhibit Gram-positive bacteria (MIC: 2–8 µg/mL) .
- Anticancer Activity: 6-Bromo-2-chloro-N-[4-(trifluoromethyl)phenyl]-3-aminoquinoxaline (compound 4 ) exhibits dual anticancer (IC₅₀: 1.2 µM) and antimicrobial effects. Phenyl-substituted analogs (e.g., 2-phenylquinoxaline) require additional functional groups (e.g., sulfonamides) for comparable efficacy .
Antiviral Activity :
- -CF₃-substituted HCV protease inhibitors (e.g., compound 18d ) show 2–4× lower potency than ethyl analogs but retain activity against drug-resistant variants (EC₅₀: 0.8–3 µM).
Biological Activity
2-Methylnaphthalene-d10 (CAS Number: 7297-45-2) is a deuterated derivative of 2-methylnaphthalene, primarily used in research to study the biological effects and environmental behavior of polycyclic aromatic hydrocarbons (PAHs). This compound is particularly significant due to its implications in human health risk assessments and its role in environmental contamination. This article explores various aspects of its biological activity, including metabolism, toxicity, and bioaccumulation.
Property | Value |
---|---|
Molecular Formula | C₁₁H₈D₁₀ |
Molecular Weight | 152.3 g/mol |
Boiling Point | 239.9 °C |
Melting Point | 34-36 °C |
Density | 1.0 g/cm³ |
Water Solubility | 0.00246 g/100 mL |
Flash Point | 97.8 °C |
Metabolism and Toxicity
Research indicates that this compound is metabolized by cytochrome P450 enzymes, which convert PAHs into hydroxylated metabolites that can be conjugated for easier excretion . The compound exhibits potential toxicity, especially in aquatic organisms, where it has been shown to be highly toxic to aquatic life with long-lasting effects .
Case Study: Human Exposure Risk Assessment
A study conducted in Ibeno, Nigeria, assessed the risk of human exposure to 2-methylnaphthalene through the consumption of contaminated shrimp (Macrobrachium vollenhovenii). The findings indicated that bioaccumulation factors were influenced by various parameters, including the shrimp's size and lipid content. The study found higher concentrations of 2-methylnaphthalene in sediment compared to fresh shrimp samples, indicating significant environmental accumulation .
Bioaccumulation and Environmental Impact
The bioaccumulation of this compound has been linked to its physicochemical properties, which affect its uptake in marine organisms. For instance, a higher lipid content in organisms correlates with increased accumulation of PAHs . The sediment-dwelling biota, such as crabs and shrimp, serve as indicators for monitoring environmental contamination levels.
Table: Bioaccumulation Factors
Organism | Bioaccumulation Factor (BSAF) |
---|---|
Macrobrachium vollenhovenii | High |
Crabs | Moderate |
Research Findings on Degradation
A significant aspect of understanding the biological activity of this compound is its degradation under anaerobic conditions. A study demonstrated that a sulfate-reducing bacterial consortium could degrade this compound at rates up to 25 μM per day, highlighting its potential for bioremediation strategies . This finding is crucial for developing methods to mitigate the environmental impact of PAHs.
Q & A
Basic Research Questions
Q. What are the standard analytical methods for characterizing 2-Methylnaphthalene-d10 in environmental samples?
Q. How is this compound validated as an internal standard in toxicokinetic studies?
- Methodological Answer : Deuterated analogs are spiked into biological matrices (e.g., plasma, tissues) to correct for extraction efficiency and ionization suppression in LC-MS/MS. Validation includes testing linearity (0.1–100 ng/mL), precision (CV <15%), and stability under storage conditions. Cross-reactivity with non-deuterated analogs must be excluded via high-resolution mass spectrometry .
Advanced Research Questions
Q. How can researchers resolve contradictions in reported toxicological outcomes for this compound?
- Methodological Answer : Apply risk-of-bias (RoB) assessment frameworks to evaluate study quality. For example:
- Animal Studies : Check randomization of dose administration and allocation concealment (Table C-7, ).
- Human Studies : Verify completeness of outcome reporting (Table C-6, ).
- Use the ATSDR confidence-rating system (Step 6–8, ) to weight evidence, prioritizing studies with low RoB and reproducible endpoints (e.g., hepatic enzyme induction or oxidative stress markers).
Q. What criteria should guide the design of inhalation exposure studies for this compound?
- Exposure Routes : Inhalation (vapor or aerosol), oral (gavage), or dermal.
- Health Outcomes : Respiratory effects (e.g., alveolar hyperplasia), hepatic CYP450 induction, or DNA adduct formation.
- Dosimetry : Use physiologically based pharmacokinetic (PBPK) models to extrapolate interspecies differences in metabolism .
Q. How can systematic reviews optimize literature searches for this compound-related mechanisms of action?
- Methodological Answer : Use multi-database query strings targeting:
- MeSH Terms : "Polycyclic Aromatic Hydrocarbons/pharmacology," "Gene Expression," "DNA Adducts."
- Keywords : "Deuterated naphthalene," "isotope tracing," "toxicodynamics."
Example search string from :
("this compound" OR "Deuterated PAHs") AND ("Toxicokinetics" OR "Metabolomics" OR "RNA Interference")
Prioritize PubMed, TOXCENTER, and NIH RePORTER for peer-reviewed studies, excluding non-English papers without translated abstracts .
Q. What methodologies are recommended for biomonitoring occupational exposure to this compound?
- Methodological Answer : Combine air sampling (NIOSH Method 5515) with urinary metabolite profiling (e.g., 2-hydroxynaphthalene-d10 glucuronide). Use isotope dilution to correct for matrix effects. Longitudinal studies should account for inter-individual variability in CYP1A2 activity, a key metabolic enzyme .
Q. Data Contradiction Analysis Framework
For conflicting results (e.g., variable LD50 values):
Assay Comparability : Ensure consistent exposure durations (acute vs. chronic) and species/strain selection.
Analytical Sensitivity : Compare detection limits of methods (e.g., GC-MS vs. ELISA).
Confounding Factors : Control for co-exposure to PAHs in environmental studies .
Properties
IUPAC Name |
1,2,3,4,5,6,8-heptadeuterio-7-(trideuteriomethyl)naphthalene | |
---|---|---|
Source | PubChem | |
URL | https://pubchem.ncbi.nlm.nih.gov | |
Description | Data deposited in or computed by PubChem | |
InChI |
InChI=1S/C11H10/c1-9-6-7-10-4-2-3-5-11(10)8-9/h2-8H,1H3/i1D3,2D,3D,4D,5D,6D,7D,8D | |
Source | PubChem | |
URL | https://pubchem.ncbi.nlm.nih.gov | |
Description | Data deposited in or computed by PubChem | |
InChI Key |
QIMMUPPBPVKWKM-UZHHFJDZSA-N | |
Source | PubChem | |
URL | https://pubchem.ncbi.nlm.nih.gov | |
Description | Data deposited in or computed by PubChem | |
Canonical SMILES |
CC1=CC2=CC=CC=C2C=C1 | |
Source | PubChem | |
URL | https://pubchem.ncbi.nlm.nih.gov | |
Description | Data deposited in or computed by PubChem | |
Isomeric SMILES |
[2H]C1=C(C(=C2C(=C(C(=C(C2=C1[2H])[2H])[2H])C([2H])([2H])[2H])[2H])[2H])[2H] | |
Source | PubChem | |
URL | https://pubchem.ncbi.nlm.nih.gov | |
Description | Data deposited in or computed by PubChem | |
Molecular Formula |
C11H10 | |
Source | PubChem | |
URL | https://pubchem.ncbi.nlm.nih.gov | |
Description | Data deposited in or computed by PubChem | |
DSSTOX Substance ID |
DTXSID10453745 | |
Record name | 2-Methylnaphthalene-d10 | |
Source | EPA DSSTox | |
URL | https://comptox.epa.gov/dashboard/DTXSID10453745 | |
Description | DSSTox provides a high quality public chemistry resource for supporting improved predictive toxicology. | |
Molecular Weight |
152.26 g/mol | |
Source | PubChem | |
URL | https://pubchem.ncbi.nlm.nih.gov | |
Description | Data deposited in or computed by PubChem | |
CAS No. |
7297-45-2 | |
Record name | 2-Methylnaphthalene-d10 | |
Source | EPA DSSTox | |
URL | https://comptox.epa.gov/dashboard/DTXSID10453745 | |
Description | DSSTox provides a high quality public chemistry resource for supporting improved predictive toxicology. | |
Record name | 7297-45-2 | |
Source | European Chemicals Agency (ECHA) | |
URL | https://echa.europa.eu/information-on-chemicals | |
Description | The European Chemicals Agency (ECHA) is an agency of the European Union which is the driving force among regulatory authorities in implementing the EU's groundbreaking chemicals legislation for the benefit of human health and the environment as well as for innovation and competitiveness. | |
Explanation | Use of the information, documents and data from the ECHA website is subject to the terms and conditions of this Legal Notice, and subject to other binding limitations provided for under applicable law, the information, documents and data made available on the ECHA website may be reproduced, distributed and/or used, totally or in part, for non-commercial purposes provided that ECHA is acknowledged as the source: "Source: European Chemicals Agency, http://echa.europa.eu/". Such acknowledgement must be included in each copy of the material. ECHA permits and encourages organisations and individuals to create links to the ECHA website under the following cumulative conditions: Links can only be made to webpages that provide a link to the Legal Notice page. | |
Synthesis routes and methods I
Procedure details
Synthesis routes and methods II
Procedure details
Synthesis routes and methods III
Procedure details
Synthesis routes and methods IV
Procedure details
Synthesis routes and methods V
Procedure details
Retrosynthesis Analysis
AI-Powered Synthesis Planning: Our tool employs the Template_relevance Pistachio, Template_relevance Bkms_metabolic, Template_relevance Pistachio_ringbreaker, Template_relevance Reaxys, Template_relevance Reaxys_biocatalysis model, leveraging a vast database of chemical reactions to predict feasible synthetic routes.
One-Step Synthesis Focus: Specifically designed for one-step synthesis, it provides concise and direct routes for your target compounds, streamlining the synthesis process.
Accurate Predictions: Utilizing the extensive PISTACHIO, BKMS_METABOLIC, PISTACHIO_RINGBREAKER, REAXYS, REAXYS_BIOCATALYSIS database, our tool offers high-accuracy predictions, reflecting the latest in chemical research and data.
Strategy Settings
Precursor scoring | Relevance Heuristic |
---|---|
Min. plausibility | 0.01 |
Model | Template_relevance |
Template Set | Pistachio/Bkms_metabolic/Pistachio_ringbreaker/Reaxys/Reaxys_biocatalysis |
Top-N result to add to graph | 6 |
Feasible Synthetic Routes
Disclaimer and Information on In-Vitro Research Products
Please be aware that all articles and product information presented on BenchChem are intended solely for informational purposes. The products available for purchase on BenchChem are specifically designed for in-vitro studies, which are conducted outside of living organisms. In-vitro studies, derived from the Latin term "in glass," involve experiments performed in controlled laboratory settings using cells or tissues. It is important to note that these products are not categorized as medicines or drugs, and they have not received approval from the FDA for the prevention, treatment, or cure of any medical condition, ailment, or disease. We must emphasize that any form of bodily introduction of these products into humans or animals is strictly prohibited by law. It is essential to adhere to these guidelines to ensure compliance with legal and ethical standards in research and experimentation.