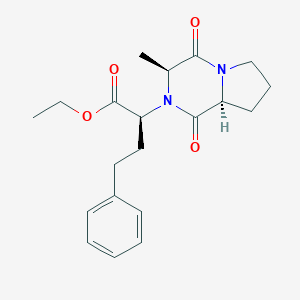
Enalapril Diketopiperazine
Overview
Description
Enalapril Diketopiperazine (DKP) is a cyclic dipeptide derivative formed as a major degradation product of the angiotensin-converting enzyme inhibitor (ACEI) enalapril maleate (EM). It arises via intramolecular cyclization under acidic conditions (pH < 2) or thermal stress during manufacturing processes such as hot-melt extrusion (HME) . Structurally, DKP retains the core diketopiperazine ring but lacks the active pharmacophore required for ACE inhibition, rendering it therapeutically inert.
Preparation Methods
Thermal-Induced Solid-State Cyclization
Mechanism of Intramolecular Cyclization
Enalapril DKP forms through a thermally driven intramolecular cyclization reaction, wherein the secondary amine and carboxylic acid groups of enalapril react to eliminate water and maleic acid. Fourier transform infrared (FT-IR) microspectroscopy has been pivotal in identifying key spectral changes during this process. For instance, the disappearance of the secondary amine peak at 3215 cm⁻¹ and the emergence of carbonyl bands at 1738 cm⁻¹ and 1672 cm⁻¹ confirm DKP formation . Differential scanning calorimetry (DSC) corroborates these findings, showing an endothermic peak at 144°C with an extrapolated onset temperature of 137°C .
Temperature-Dependent Reaction Kinetics
Thermogravimetric studies reveal that DKP synthesis initiates at 129°C in solid-state EM, reaching maximum formation at 137°C . The reaction’s activation energy, calculated using the Kissinger–Akahira–Sunose isoconversional method, varies significantly with experimental conditions. For pure EM, activation energies range from 141.2 kJ/mol to 198 kJ/mol , depending on the analytical technique and environmental factors. These discrepancies highlight the sensitivity of DKP formation to heating rates and sample preparation.
Table 1: Thermal Parameters for DKP Formation
Parameter | Value | Method | Source |
---|---|---|---|
Onset Temperature | 129°C | Thermal FT-IR | |
Peak Temperature | 137°C | DSC | |
Activation Energy (EM) | 141.2–198 kJ/mol | Isoconversional KAS |
Influence of Excipients on DKP Formation
Accelerated Degradation with Eudragit E
Eudragit E, a cationic copolymer used in drug coatings, markedly accelerates DKP formation. Reflectance FT-IR studies demonstrate that mixing EM with Eudragit E (1:1 weight ratio) reduces the activation energy to 86.7 kJ/mol, compared to 141.2 kJ/mol for pure EM . This effect arises from interactions between Eudragit E’s tertiary amine groups and EM’s carboxyl moieties, which destabilize the maleate salt and promote cyclization. However, at higher Eudragit E ratios (e.g., 2:1), the polymer’s excess binds free carboxyl groups, paradoxically stabilizing EM .
Role of Hydrophobic Binders
Stearic acid and glyceryl monostearate, employed in high-shear melt granulation, mitigate DKP formation by reducing EM’s exposure to moisture and heat. Formulations with stearic acid exhibit only 2.5% DKP after six months at 40°C, compared to 12% in unmodified tablets . This stabilization occurs via hydrophobic encapsulation, which limits intramolecular interactions critical for cyclization.
Table 2: Impact of Excipients on DKP Formation
Excipient | Weight Ratio (EM:Excipient) | Activation Energy (kJ/mol) | DKP Yield (%) |
---|---|---|---|
None (Pure EM) | – | 141.2 | 14.0 |
Eudragit E | 1:1 | 86.7 | 32.0 |
Stearic Acid | 1:0.5 | – | 2.5 |
Kinetic Analysis and Reaction Mechanisms
Solid-State vs. Liquid-State Pathways
Below EM’s melting point (≈155°C), DKP formation follows a nucleation-controlled mechanism, where reaction progress depends on the spontaneous formation of critical nuclei in the solid matrix . Above 155°C, the melt-mediated pathway dominates, adhering to first-order kinetics with a rate constant proportional to temperature. This duality complicates kinetic modeling but underscores the importance of phase transitions in optimizing synthesis conditions.
Model-Free Kinetic Simulations
Isothermal simulations at 125–155°C predict DKP yields using the model-free method, which relies solely on activation energy values. For instance, at 135°C, the reaction reaches 50% conversion within 120 minutes, aligning with experimental data . These simulations enable precise control over reaction duration and temperature, critical for scaling DKP production.
Table 3: Kinetic Parameters for DKP Synthesis
Temperature (°C) | Mechanism | Rate Constant (min⁻¹) | Half-Life (min) |
---|---|---|---|
125 | Nucleation | 0.0032 | 216 |
135 | Nucleation | 0.0058 | 120 |
155 | First-Order | 0.0124 | 56 |
Formulation Techniques and Stability Considerations
Patent Innovations in Liquid Formulations
US Patent 11,173,141-B2 discloses stable enalapril oral liquids using buffering agents (e.g., citrate) to maintain pH 3.5–4.5, thereby suppressing DKP formation . While primarily aimed at enhancing shelf-life, this approach informs DKP synthesis by illustrating how pH modulation can steer degradation pathways toward or away from cyclization.
Chemical Reactions Analysis
Types of Reactions: Enalapril diketopiperazine undergoes various chemical reactions, including oxidation, reduction, and substitution. The compound is known to form through thermal-induced intramolecular interactions, leading to the formation of a six-membered cyclic anhydride .
Common Reagents and Conditions: The formation of this compound is influenced by the presence of polymers like Eudragit E, which interact with the carboxyl group of enalapril maleate. The degradation process is also affected by the pH of the environment, with neutral pH promoting the highest stability .
Major Products Formed: The major product formed from the thermal degradation of enalapril maleate is this compound. This compound is characterized by its six-membered cyclic anhydride structure .
Scientific Research Applications
Chemical Properties and Stability
Enalapril DKP has a molecular formula of and a molecular weight of 358.43 g/mol. It is formed through thermal degradation processes of enalapril, often influenced by environmental conditions such as temperature and humidity. The stability of enalapril and its derivatives, including DKP, is crucial for ensuring the efficacy and safety of pharmaceutical formulations.
Stability Studies
Research indicates that enalapril undergoes intramolecular cyclization leading to DKP formation under thermal stress. A study demonstrated that the presence of excipients like Eudragit E can accelerate this degradation process, highlighting the need for careful formulation strategies to enhance the stability of enalapril-based products .
Condition | Degradation Product | Formation Rate |
---|---|---|
High Temperature | Enalaprilat, DKP | Increased |
Low Temperature | Minimal DKP Formation | Decreased |
Humidity | Accelerated DKP Formation | High |
Analytical Methods for Detection
Various analytical techniques have been employed to quantify Enalapril DKP in pharmaceutical formulations. High-Performance Thin-Layer Chromatography (HPTLC) has been effectively utilized to distinguish between enalapril, enalaprilat, and DKP based on their retention factors (Rf values). For instance, Rf values were reported as 0.52 for enalaprilat, 0.62 for enalapril, and 0.82 for DKP . This method allows for accurate monitoring of DKP levels during stability studies.
Clinical Implications
The clinical relevance of Enalapril DKP lies in its implications for drug formulation and safety. As a known impurity, its presence in pharmaceutical products can affect the therapeutic efficacy and safety profile of enalapril. Research has shown that formulations containing stearic acid can stabilize enalapril by reducing the formation of both enalaprilate and DKP .
Case Studies
- LENA Project : A clinical study aimed at developing an age-appropriate formulation of enalapril for pediatric use highlighted the importance of controlling degradation products like DKP. The study found that a novel formulation demonstrated bioavailability comparable to traditional tablets while being clinically safe for children .
- Stability Analysis : A study on the interaction between enalapril maleate and various excipients revealed that certain combinations could lead to increased DKP formation, necessitating thorough compatibility testing during formulation development .
Mechanism of Action
Enalapril diketopiperazine can be compared with other diketopiperazine derivatives and angiotensin-converting enzyme inhibitors. Similar compounds include captopril and quinapril, both of which are angiotensin-converting enzyme inhibitors used to treat hypertension and heart failure . Unlike this compound, captopril contains a sulfhydryl group, which may be associated with a higher incidence of adverse reactions . Quinapril, on the other hand, is de-esterified in the liver to its active form, quinaprilat, similar to the conversion of enalapril to enalaprilat . The unique thermal degradation properties and the influence of polymers on the stability of this compound distinguish it from these similar compounds.
Comparison with Similar Compounds
Comparison with Structurally Related ACE Inhibitors
ACE inhibitors sharing the N-carboxyalkyl dipeptide backbone are prone to DKP formation. Below is a comparative analysis of enalapril DKP with analogous degradation products:
Structural and Degradation Pathway Similarities
Compound | Degradation Pathway | Primary Degradant | Conditions Promoting DKP Formation |
---|---|---|---|
Enalapril | Intramolecular cyclization | Enalapril DKP | Acidic pH (<2), thermal stress (>70°C) |
Imidapril | Hydrolysis + cyclization | Imidapril DKP | High humidity, nitrosation |
Quinapril | Cyclization | Quinapril DKP | Solid-state storage, pH variability |
Ramipril | Thermal degradation | Ramipril DKP | High-temperature processing |
Moexipril | Hydrolysis followed by cyclization | Moexipril DKP | Moisture, excipient interactions |
Key Observations :
- Enalapril DKP is predominantly formed under acidic or thermal conditions, with up to 26.48% degradation observed during HME at 100°C .
- typhimurium TA 1535 post-nitrosation (1.12 mg/mL) .
- Quinapril DKP resists stabilization via salt formation, a method effective for enalapril and moexipril .
Mutagenicity and Carcinogenic Potential
- Enalapril DKP : Demonstrates mutagenicity in S. typhimurium TA 1535 after N-nitrosation, likely due to nitroso compound generation in gastric environments .
- Imidapril DKP: Non-mutagenic in Ames tests (TA 98/100 strains) unless nitrosated, highlighting divergent safety profiles among ACEIs .
Stabilization Strategies
Notable Findings:
- β-cyclodextrin enhances enalapril stability by 2.1-fold in solution via molecular encapsulation .
- Stearic acid reduces DKP formation in enalapril pellets by mitigating intramolecular cyclization .
Pharmaceutical Implications
- Thermal Degradation : Enalapril DKP formation escalates at extrusion temperatures >70°C, necessitating low-temperature processing with polymers like Eudragit® E PO .
- Dissolution Impact : Tablets with 20% infill release 81.93% enalapril and 19.27% DKP , underscoring formulation-dependent degradation .
- Analytical Methods : HPTLC and HPLC methods (LOD: 19.4 ng/mL) reliably quantify DKP, critical for quality control .
Biological Activity
Enalapril, a widely used antihypertensive medication, is a prodrug that converts to its active form, enalaprilat, after administration. During the degradation of enalapril maleate (EM), diketopiperazine (DKP) is formed as a significant degradation product. This article examines the biological activity of enalapril diketopiperazine, focusing on its pharmacological effects, stability, and mechanisms of action.
Enalapril itself acts primarily by inhibiting the angiotensin-converting enzyme (ACE), which leads to decreased production of angiotensin II, a potent vasoconstrictor. This results in lowered blood pressure and reduced strain on the cardiovascular system. The diketopiperazine derivative formed during the degradation process may exhibit similar or altered biological activities.
Pharmacological Studies
- Antioxidant Properties : Research indicates that enalapril and its derivatives enhance glutathione-dependent antioxidant defenses in various tissues. For instance, a study demonstrated that enalapril significantly increased total glutathione content and activities of antioxidant enzymes in mouse tissues, suggesting potential protective effects against oxidative stress .
- Respiratory Effects : A study involving co-administration of enalapril with diltiazem found that while enalapril increased cough reflex and tracheal smooth muscle reactivity, the combination therapy mitigated these adverse effects. This suggests that DKP might influence respiratory responses when formed from enalapril .
- Stability and Degradation : The stability of enalapril and its conversion to diketopiperazine are influenced by environmental factors such as pH and temperature. Under acidic conditions, DKP formation is favored, while alkaline conditions lead primarily to enalaprilat formation . The degradation kinetics indicate that DKP can form through thermal degradation pathways, which can impact the biological availability and efficacy of enalapril formulations .
Case Studies
Several studies have investigated the biological implications of diketopiperazine formation:
- Study on Stability : A study assessed the stability of enalapril maleate under various stress conditions, revealing that DKP was a major degradation product under both hydrolytic and thermal stress. The findings emphasized the need for formulation strategies to minimize DKP formation to maintain drug efficacy .
- Pharmacokinetic Studies : Clinical pharmacokinetic studies have shown that variations in formulation can affect the rate at which enalapril is converted to its active form and its degradation products. For example, formulations containing stearic acid were found to stabilize enalapril against rapid degradation into DKP .
Table 1: Stability Data of Enalapril Maleate Under Different Conditions
Condition | Time (hrs) | % Remaining Enalapril | Major Degradation Product |
---|---|---|---|
Hydrolysis (0.1 N NaOH) | 0.5 | 7.5 | Enalaprilat |
Hydrolysis (Water) | 24 | 95.2 | Diketopiperazine |
Thermal Stress | Varies | Decreases over time | Diketopiperazine |
Table 2: Antioxidant Activity Enhancement by Enalapril
Tissue | GSSG + GSH Content Increase (%) | Se-GPx Activity Increase (%) |
---|---|---|
Erythrocytes | 147 | 21 |
Brain | 112 | 42 |
Lung | 67 | Not measured |
Q & A
Basic Research Questions
Q. What analytical methods are recommended for identifying and quantifying Enalapril Diketopiperazine in pharmaceutical formulations?
- Methodology : Use reversed-phase HPLC with a C18 column (e.g., 150 mm × 4.6 mm, 5 μm) and a mobile phase of methanol-phosphate buffer (pH 2.2, 0.01 M) in a 55:45 ratio. Detection is performed at 215 nm with a flow rate of 1.0 mL/min. System suitability requires resolution ≥2.0 between DKP, enalapril, enalaprilat, and maleic acid peaks. Salicylic acid (60 μg/mL) is used as an internal standard for quantification .
- Validation : Ensure limits of detection (DL: 0.18 μg/mL) and quantitation (QL: 0.56 μg/mL) are met using calibration curves. Intra-assay precision should have RSD ≤2.0% .
Q. Which factors accelerate DKP formation in enalapril formulations?
- Key Factors :
- Temperature/Humidity : Accelerated stability studies (40°C/75% RH) show increased DKP formation over time due to cyclization .
- pH : Neutral and alkaline conditions favor intramolecular aminolysis, leading to DKP, while acidic conditions promote concurrent hydrolysis .
- Excipients : Certain tablet excipients (e.g., binders) may catalyze degradation. Use pH 2.2 phosphate buffers to stabilize formulations during testing .
Q. What are the standard protocols for stress testing enalapril to study DKP degradation?
- Stress Conditions :
- Hydrolytic : Expose enalapril to 0.1 M HCl (acidic), pH 7.0 buffer (neutral), or 0.1 M NaOH (alkaline) at 80°C for 24–72 hours .
- Thermal : Heat solid-state enalapril maleate at 120–130°C to observe DKP formation via FT-IR (peaks at 1672 cm⁻¹ and 1738 cm⁻¹) .
- Oxidative : Use 3% H₂O₂; enalapril is stable under oxidative stress, with minimal DKP formation .
Q. How are detection limits and system suitability validated for DKP analysis?
- Detection Limits : Calculate using DL = 3.3σ/S and QL = 10σ/S, where σ is the standard deviation of the response and S is the calibration curve slope. For HPLC, DL = 0.18 μg/mL and QL = 0.56 μg/mL .
- System Suitability : Ensure column efficiency ≥1000 theoretical plates/meter for enalaprilat and ≥2500 plates/meter for DKP. Peak tailing for enalapril must be ≤2.0 .
Q. What pharmacopeial specifications govern DKP quantification?
- USP/BP Requirements : Total related substances (including DKP) must not exceed 5.0% in formulations. Use resolution solutions containing 0.003 mg/mL enalaprilat and 0.3 mg/mL enalapril maleate to validate chromatographic separation .
Advanced Research Questions
Q. What mechanistic pathways explain DKP formation versus amide hydrolysis in enalapril degradation?
- Cyclization vs. Hydrolysis : Under neutral/alkaline conditions, intramolecular aminolysis dominates, forming DKP via a six-membered transition state. Acidic conditions favor ester hydrolysis to enalaprilat, with slower DKP formation due to protonation of the amine nucleophile .
- Kinetics : Solid-state degradation follows autocatalytic kinetics (activation energy: 195 ± 12 kJ/mol) with sigmoidal decomposition curves .
Q. How can contradictions in degradation rates across studies be resolved?
- Case Example : Some studies report higher DKP levels in tablets under accelerated conditions, while others note variability due to excipient interactions. Resolve discrepancies by standardizing stress protocols (e.g., fixed humidity/temperature) and using excipient-free controls .
- Data Normalization : Express degradation rates as % w/w of initial enalapril content and account for matrix effects using forced degradation studies .
Q. How do advanced formulation techniques (e.g., 3D printing) influence DKP formation?
- Hot-Melt Extrusion : Processing at 70°C increases DKP content (up to 22.74% in 3D-printed tablets) due to thermal cyclization. Reduced infill density (20–25%) marginally improves stability but requires geometry adjustments to maintain dosage .
- Polymer Interactions : FT-IR analysis reveals no chemical interactions between enalapril and polymers like bPMMA, suggesting physical stability challenges dominate .
Q. What computational models predict DKP stability and crystallinity?
- In Silico Tools : Simulated annealing Monte Carlo (SAMC) predicts hydrogen-bonded crystalline packing of DKP derivatives. Validate using powder diffraction and Ck* (crystalline packing efficiency) metrics .
- Degradation Modeling : Use Arrhenius equations to extrapolate shelf life from accelerated stability data, incorporating activation energy values .
Q. Which advanced spectroscopic techniques characterize DKP and its degradation intermediates?
Properties
IUPAC Name |
ethyl (2S)-2-[(3S,8aS)-3-methyl-1,4-dioxo-6,7,8,8a-tetrahydro-3H-pyrrolo[1,2-a]pyrazin-2-yl]-4-phenylbutanoate | |
---|---|---|
Source | PubChem | |
URL | https://pubchem.ncbi.nlm.nih.gov | |
Description | Data deposited in or computed by PubChem | |
InChI |
InChI=1S/C20H26N2O4/c1-3-26-20(25)17(12-11-15-8-5-4-6-9-15)22-14(2)18(23)21-13-7-10-16(21)19(22)24/h4-6,8-9,14,16-17H,3,7,10-13H2,1-2H3/t14-,16-,17-/m0/s1 | |
Source | PubChem | |
URL | https://pubchem.ncbi.nlm.nih.gov | |
Description | Data deposited in or computed by PubChem | |
InChI Key |
BMZHNHHJUGMMLV-XIRDDKMYSA-N | |
Source | PubChem | |
URL | https://pubchem.ncbi.nlm.nih.gov | |
Description | Data deposited in or computed by PubChem | |
Canonical SMILES |
CCOC(=O)C(CCC1=CC=CC=C1)N2C(C(=O)N3CCCC3C2=O)C | |
Source | PubChem | |
URL | https://pubchem.ncbi.nlm.nih.gov | |
Description | Data deposited in or computed by PubChem | |
Isomeric SMILES |
CCOC(=O)[C@H](CCC1=CC=CC=C1)N2[C@H](C(=O)N3CCC[C@H]3C2=O)C | |
Source | PubChem | |
URL | https://pubchem.ncbi.nlm.nih.gov | |
Description | Data deposited in or computed by PubChem | |
Molecular Formula |
C20H26N2O4 | |
Source | PubChem | |
URL | https://pubchem.ncbi.nlm.nih.gov | |
Description | Data deposited in or computed by PubChem | |
DSSTOX Substance ID |
DTXSID10151178 | |
Record name | Enalapril diketopiperazine | |
Source | EPA DSSTox | |
URL | https://comptox.epa.gov/dashboard/DTXSID10151178 | |
Description | DSSTox provides a high quality public chemistry resource for supporting improved predictive toxicology. | |
Molecular Weight |
358.4 g/mol | |
Source | PubChem | |
URL | https://pubchem.ncbi.nlm.nih.gov | |
Description | Data deposited in or computed by PubChem | |
CAS No. |
115729-52-7 | |
Record name | Ethyl (αS,3S,8aS)-hexahydro-3-methyl-1,4-dioxo-α-(2-phenylethyl)pyrrolo[1,2-a]pyrazine-2(1H)-acetate | |
Source | CAS Common Chemistry | |
URL | https://commonchemistry.cas.org/detail?cas_rn=115729-52-7 | |
Description | CAS Common Chemistry is an open community resource for accessing chemical information. Nearly 500,000 chemical substances from CAS REGISTRY cover areas of community interest, including common and frequently regulated chemicals, and those relevant to high school and undergraduate chemistry classes. This chemical information, curated by our expert scientists, is provided in alignment with our mission as a division of the American Chemical Society. | |
Explanation | The data from CAS Common Chemistry is provided under a CC-BY-NC 4.0 license, unless otherwise stated. | |
Record name | Enalapril diketopiperazine | |
Source | ChemIDplus | |
URL | https://pubchem.ncbi.nlm.nih.gov/substance/?source=chemidplus&sourceid=0115729527 | |
Description | ChemIDplus is a free, web search system that provides access to the structure and nomenclature authority files used for the identification of chemical substances cited in National Library of Medicine (NLM) databases, including the TOXNET system. | |
Record name | Enalapril diketopiperazine | |
Source | EPA DSSTox | |
URL | https://comptox.epa.gov/dashboard/DTXSID10151178 | |
Description | DSSTox provides a high quality public chemistry resource for supporting improved predictive toxicology. | |
Record name | 2-[1-(S)-Ethoxycarbonyl-3-phenylpropyl]-3-methyl-hexahydro-pyrrolo[1,2-a]pyrazin-1,4-dion | |
Source | European Chemicals Agency (ECHA) | |
URL | https://echa.europa.eu/information-on-chemicals | |
Description | The European Chemicals Agency (ECHA) is an agency of the European Union which is the driving force among regulatory authorities in implementing the EU's groundbreaking chemicals legislation for the benefit of human health and the environment as well as for innovation and competitiveness. | |
Explanation | Use of the information, documents and data from the ECHA website is subject to the terms and conditions of this Legal Notice, and subject to other binding limitations provided for under applicable law, the information, documents and data made available on the ECHA website may be reproduced, distributed and/or used, totally or in part, for non-commercial purposes provided that ECHA is acknowledged as the source: "Source: European Chemicals Agency, http://echa.europa.eu/". Such acknowledgement must be included in each copy of the material. ECHA permits and encourages organisations and individuals to create links to the ECHA website under the following cumulative conditions: Links can only be made to webpages that provide a link to the Legal Notice page. | |
Record name | ENALAPRIL DIKETOPIPERAZINE | |
Source | FDA Global Substance Registration System (GSRS) | |
URL | https://gsrs.ncats.nih.gov/ginas/app/beta/substances/609HCJ5DV4 | |
Description | The FDA Global Substance Registration System (GSRS) enables the efficient and accurate exchange of information on what substances are in regulated products. Instead of relying on names, which vary across regulatory domains, countries, and regions, the GSRS knowledge base makes it possible for substances to be defined by standardized, scientific descriptions. | |
Explanation | Unless otherwise noted, the contents of the FDA website (www.fda.gov), both text and graphics, are not copyrighted. They are in the public domain and may be republished, reprinted and otherwise used freely by anyone without the need to obtain permission from FDA. Credit to the U.S. Food and Drug Administration as the source is appreciated but not required. | |
Retrosynthesis Analysis
AI-Powered Synthesis Planning: Our tool employs the Template_relevance Pistachio, Template_relevance Bkms_metabolic, Template_relevance Pistachio_ringbreaker, Template_relevance Reaxys, Template_relevance Reaxys_biocatalysis model, leveraging a vast database of chemical reactions to predict feasible synthetic routes.
One-Step Synthesis Focus: Specifically designed for one-step synthesis, it provides concise and direct routes for your target compounds, streamlining the synthesis process.
Accurate Predictions: Utilizing the extensive PISTACHIO, BKMS_METABOLIC, PISTACHIO_RINGBREAKER, REAXYS, REAXYS_BIOCATALYSIS database, our tool offers high-accuracy predictions, reflecting the latest in chemical research and data.
Strategy Settings
Precursor scoring | Relevance Heuristic |
---|---|
Min. plausibility | 0.01 |
Model | Template_relevance |
Template Set | Pistachio/Bkms_metabolic/Pistachio_ringbreaker/Reaxys/Reaxys_biocatalysis |
Top-N result to add to graph | 6 |
Feasible Synthetic Routes
Disclaimer and Information on In-Vitro Research Products
Please be aware that all articles and product information presented on BenchChem are intended solely for informational purposes. The products available for purchase on BenchChem are specifically designed for in-vitro studies, which are conducted outside of living organisms. In-vitro studies, derived from the Latin term "in glass," involve experiments performed in controlled laboratory settings using cells or tissues. It is important to note that these products are not categorized as medicines or drugs, and they have not received approval from the FDA for the prevention, treatment, or cure of any medical condition, ailment, or disease. We must emphasize that any form of bodily introduction of these products into humans or animals is strictly prohibited by law. It is essential to adhere to these guidelines to ensure compliance with legal and ethical standards in research and experimentation.