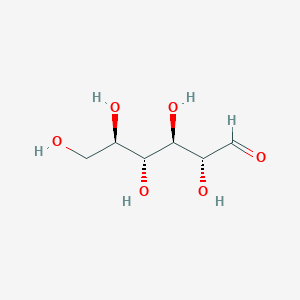
(2R,3R,4R,5R)-2,3,4,5,6-pentahydroxyhexanal
Overview
Description
“(2R,3R,4R,5R)-2,3,4,5,6-pentahydroxyhexanal” is a chemical compound with the molecular formula C6H12O6 . It is not a naturally occurring metabolite and is only found in individuals exposed to this compound or its derivatives . This molecule contains a total of 23 bonds, including 11 non-H bonds, 1 multiple bond, 5 rotatable bonds, 1 double bond, 1 aldehyde (aliphatic), 5 hydroxyl groups, 1 primary alcohol, and 4 secondary alcohols .
Molecular Structure Analysis
The molecular structure of “(2R,3R,4R,5R)-2,3,4,5,6-pentahydroxyhexanal” includes the arrangement of atoms and the chemical bonds that hold the atoms together . The 2D chemical structure image of this compound is also called a skeletal formula, which is the standard notation for organic molecules . The 3D chemical structure image of this compound is based on the ball-and-stick model which displays both the three-dimensional position of the atoms and the bonds between them .Physical And Chemical Properties Analysis
The molecular weight of “(2R,3R,4R,5R)-2,3,4,5,6-pentahydroxyhexanal” is 180.15588 g/mol . The molecule contains a total of 23 bonds, including 11 non-H bonds, 1 multiple bond, 5 rotatable bonds, 1 double bond, 1 aldehyde (aliphatic), 5 hydroxyl groups, 1 primary alcohol, and 4 secondary alcohols .Scientific Research Applications
Solubility in Ethanol-Water Solutions : A study by Gong et al. (2012) investigated the solubility of various saccharides, including "(2R,3R,4R,5R)-2,3,5,6-tetrahydroxy-4-[(2R,3R,4S,5S,6R)-3,4,5-trihydroxy-6-(hydroxymethyl)oxan-2-yl]oxyhexanal hydrate" (maltose monohydrate), in ethanol-water solutions. They found that the solubility of these saccharides in ethanol-water mixtures increased with equilibrium temperature. This research is significant for understanding the solubility behavior of complex saccharides in mixed solvents, which can be crucial for various applications in pharmaceutical and chemical industries (Gong, Wang, Zhang, & Qu, 2012).
Synthesis of Iminosugars : Estévez et al. (2010) described the synthesis of polyhydroxylated azepanes, including "(2R,3R,4R,5R,6R)-2-(hydroxymethyl)azepane-3,4,5,6-tetraol" from D-mannose. This compound showed weak but selective inhibition against α-L-fucosides, indicating its potential as a glycosidase inhibitor. This is relevant for the development of therapeutic agents targeting specific enzymes (Estévez, Soengas, Otero, Estévez, Nash, & Estévez, 2010).
Stereoselective Synthesis for Sialic Acid Aldolase Evolution : Woodhall et al. (2005) conducted research on the stereoselective synthesis of epimeric screening substrates for the directed evolution of sialic acid aldolase. This research is important for developing tailored enzymes for the preparation of influenza A sialidase inhibitor analogues. The study demonstrates the potential of these compounds in medicinal chemistry, particularly in the development of antiviral drugs (Woodhall, Williams, Berry, & Nelson, 2005).
NMR Spectroscopy to Reveal Hydrogen Bonding Properties : Oruç et al. (2018) used NMR spectroscopy to investigate the hydrogen bonding properties of polyols, including "(2R,3R,4R,5R)-hexane-1,2,3,4,5,6-hexaol" (D-mannitol). This research provides insight into the solution properties of polyols, which is crucial for understanding their behavior in biological systems and potential applications in drug delivery and formulation (Oruç, Varnali, & Bekiroğlu, 2018).
properties
IUPAC Name |
(2R,3R,4R,5R)-2,3,4,5,6-pentahydroxyhexanal | |
---|---|---|
Source | PubChem | |
URL | https://pubchem.ncbi.nlm.nih.gov | |
Description | Data deposited in or computed by PubChem | |
InChI |
InChI=1S/C6H12O6/c7-1-3(9)5(11)6(12)4(10)2-8/h1,3-6,8-12H,2H2/t3-,4+,5-,6+/m0/s1 | |
Source | PubChem | |
URL | https://pubchem.ncbi.nlm.nih.gov | |
Description | Data deposited in or computed by PubChem | |
InChI Key |
GZCGUPFRVQAUEE-BGPJRJDNSA-N | |
Source | PubChem | |
URL | https://pubchem.ncbi.nlm.nih.gov | |
Description | Data deposited in or computed by PubChem | |
Canonical SMILES |
C(C(C(C(C(C=O)O)O)O)O)O | |
Source | PubChem | |
URL | https://pubchem.ncbi.nlm.nih.gov | |
Description | Data deposited in or computed by PubChem | |
Isomeric SMILES |
C([C@H]([C@H]([C@H]([C@H](C=O)O)O)O)O)O | |
Source | PubChem | |
URL | https://pubchem.ncbi.nlm.nih.gov | |
Description | Data deposited in or computed by PubChem | |
Molecular Formula |
C6H12O6 | |
Source | PubChem | |
URL | https://pubchem.ncbi.nlm.nih.gov | |
Description | Data deposited in or computed by PubChem | |
DSSTOX Substance ID |
DTXSID901015879 | |
Record name | D-Allose | |
Source | EPA DSSTox | |
URL | https://comptox.epa.gov/dashboard/DTXSID901015879 | |
Description | DSSTox provides a high quality public chemistry resource for supporting improved predictive toxicology. | |
Molecular Weight |
180.16 g/mol | |
Source | PubChem | |
URL | https://pubchem.ncbi.nlm.nih.gov | |
Description | Data deposited in or computed by PubChem | |
Product Name |
(2R,3R,4R,5R)-2,3,4,5,6-pentahydroxyhexanal | |
CAS RN |
6038-51-3, 2595-97-3 | |
Record name | Allose | |
Source | CAS Common Chemistry | |
URL | https://commonchemistry.cas.org/detail?cas_rn=6038-51-3 | |
Description | CAS Common Chemistry is an open community resource for accessing chemical information. Nearly 500,000 chemical substances from CAS REGISTRY cover areas of community interest, including common and frequently regulated chemicals, and those relevant to high school and undergraduate chemistry classes. This chemical information, curated by our expert scientists, is provided in alignment with our mission as a division of the American Chemical Society. | |
Explanation | The data from CAS Common Chemistry is provided under a CC-BY-NC 4.0 license, unless otherwise stated. | |
Record name | D-Allose | |
Source | CAS Common Chemistry | |
URL | https://commonchemistry.cas.org/detail?cas_rn=2595-97-3 | |
Description | CAS Common Chemistry is an open community resource for accessing chemical information. Nearly 500,000 chemical substances from CAS REGISTRY cover areas of community interest, including common and frequently regulated chemicals, and those relevant to high school and undergraduate chemistry classes. This chemical information, curated by our expert scientists, is provided in alignment with our mission as a division of the American Chemical Society. | |
Explanation | The data from CAS Common Chemistry is provided under a CC-BY-NC 4.0 license, unless otherwise stated. | |
Record name | D-Allose | |
Source | ChemIDplus | |
URL | https://pubchem.ncbi.nlm.nih.gov/substance/?source=chemidplus&sourceid=0002595973 | |
Description | ChemIDplus is a free, web search system that provides access to the structure and nomenclature authority files used for the identification of chemical substances cited in National Library of Medicine (NLM) databases, including the TOXNET system. | |
Record name | DL-Allose | |
Source | ChemIDplus | |
URL | https://pubchem.ncbi.nlm.nih.gov/substance/?source=chemidplus&sourceid=0006038513 | |
Description | ChemIDplus is a free, web search system that provides access to the structure and nomenclature authority files used for the identification of chemical substances cited in National Library of Medicine (NLM) databases, including the TOXNET system. | |
Record name | D-Allose | |
Source | EPA DSSTox | |
URL | https://comptox.epa.gov/dashboard/DTXSID901015879 | |
Description | DSSTox provides a high quality public chemistry resource for supporting improved predictive toxicology. | |
Record name | D-allose | |
Source | European Chemicals Agency (ECHA) | |
URL | https://echa.europa.eu/substance-information/-/substanceinfo/100.018.178 | |
Description | The European Chemicals Agency (ECHA) is an agency of the European Union which is the driving force among regulatory authorities in implementing the EU's groundbreaking chemicals legislation for the benefit of human health and the environment as well as for innovation and competitiveness. | |
Explanation | Use of the information, documents and data from the ECHA website is subject to the terms and conditions of this Legal Notice, and subject to other binding limitations provided for under applicable law, the information, documents and data made available on the ECHA website may be reproduced, distributed and/or used, totally or in part, for non-commercial purposes provided that ECHA is acknowledged as the source: "Source: European Chemicals Agency, http://echa.europa.eu/". Such acknowledgement must be included in each copy of the material. ECHA permits and encourages organisations and individuals to create links to the ECHA website under the following cumulative conditions: Links can only be made to webpages that provide a link to the Legal Notice page. | |
Record name | ALLOSE, D- | |
Source | FDA Global Substance Registration System (GSRS) | |
URL | https://gsrs.ncats.nih.gov/ginas/app/beta/substances/SV1ATP0KYY | |
Description | The FDA Global Substance Registration System (GSRS) enables the efficient and accurate exchange of information on what substances are in regulated products. Instead of relying on names, which vary across regulatory domains, countries, and regions, the GSRS knowledge base makes it possible for substances to be defined by standardized, scientific descriptions. | |
Explanation | Unless otherwise noted, the contents of the FDA website (www.fda.gov), both text and graphics, are not copyrighted. They are in the public domain and may be republished, reprinted and otherwise used freely by anyone without the need to obtain permission from FDA. Credit to the U.S. Food and Drug Administration as the source is appreciated but not required. | |
Retrosynthesis Analysis
AI-Powered Synthesis Planning: Our tool employs the Template_relevance Pistachio, Template_relevance Bkms_metabolic, Template_relevance Pistachio_ringbreaker, Template_relevance Reaxys, Template_relevance Reaxys_biocatalysis model, leveraging a vast database of chemical reactions to predict feasible synthetic routes.
One-Step Synthesis Focus: Specifically designed for one-step synthesis, it provides concise and direct routes for your target compounds, streamlining the synthesis process.
Accurate Predictions: Utilizing the extensive PISTACHIO, BKMS_METABOLIC, PISTACHIO_RINGBREAKER, REAXYS, REAXYS_BIOCATALYSIS database, our tool offers high-accuracy predictions, reflecting the latest in chemical research and data.
Strategy Settings
Precursor scoring | Relevance Heuristic |
---|---|
Min. plausibility | 0.01 |
Model | Template_relevance |
Template Set | Pistachio/Bkms_metabolic/Pistachio_ringbreaker/Reaxys/Reaxys_biocatalysis |
Top-N result to add to graph | 6 |
Feasible Synthetic Routes
Disclaimer and Information on In-Vitro Research Products
Please be aware that all articles and product information presented on BenchChem are intended solely for informational purposes. The products available for purchase on BenchChem are specifically designed for in-vitro studies, which are conducted outside of living organisms. In-vitro studies, derived from the Latin term "in glass," involve experiments performed in controlled laboratory settings using cells or tissues. It is important to note that these products are not categorized as medicines or drugs, and they have not received approval from the FDA for the prevention, treatment, or cure of any medical condition, ailment, or disease. We must emphasize that any form of bodily introduction of these products into humans or animals is strictly prohibited by law. It is essential to adhere to these guidelines to ensure compliance with legal and ethical standards in research and experimentation.