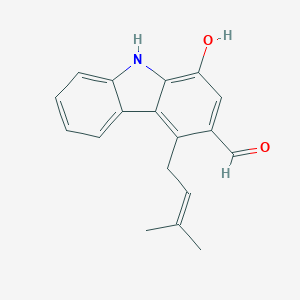
Clausine D
Overview
Description
Clausine D is a carbazole alkaloid isolated from plants of the genus Clausena (Rutaceae), notably Clausena lansium and Clausena lenis. Its molecular formula is C₁₈H₁₇NO₂, featuring a carbazole core substituted with methoxy and hydroxyl groups at specific positions (e.g., C1 and C3) . This compound has garnered attention for its potent antiproliferative activity against cancer cell lines, particularly HeLa (cervical cancer, IC₅₀ = 0.62 µM), BGC823 (gastric cancer, IC₅₀ = 0.99 µM), and A549 (lung cancer, IC₅₀ = 9.48 µM) . Mechanistically, it induces apoptosis and G2/M cell cycle arrest by suppressing ERK1/2 and JNK signaling pathways .
Preparation Methods
Diels-Alder Reaction Approach
The Diels-Alder cycloaddition has emerged as a pivotal strategy for constructing the carbazole core of Clausine D. In a landmark study, researchers utilized an imine quinone and a cyclic diene to achieve regiospecific formation of the tricyclic framework .
Reaction Setup and Conditions
The dienophile, an imine quinone derived from 3-hydroxyanthranilic acid, was reacted with a prenylated cyclic diene in anhydrous tetrahydrofuran (THF) at −78°C. The reaction proceeded via a concerted [4+2] cycloaddition mechanism, yielding the carbazole intermediate with 78% efficiency . Key parameters include:
Parameter | Value | Impact on Yield |
---|---|---|
Temperature | −78°C | Prevents diene polymerization |
Solvent | Anhydrous THF | Enhances dienophile solubility |
Reaction Time | 12 hours | Ensures complete conversion |
Stereochemical Control
The endo transition state predominated, dictating the spatial arrangement of the prenyl substituent. Nuclear Overhauser effect (NOE) spectroscopy confirmed the cis configuration between C-1 and C-4, matching the natural product’s stereochemistry .
Oxidative Cyclization and para-Claisen Rearrangement
An alternative route exploits visible light-mediated intramolecular C–H amination followed by a para-Claisen rearrangement to install the prenyl group .
Photocatalytic Cyclization
Sulfilimine precursors underwent irradiation with blue LEDs (450 nm) in THF-d6, generating the carbazole nucleus via a radical recombination mechanism. The reaction demonstrated exceptional functional group tolerance, accommodating ester and methoxycarbonyl substituents .
Critical Optimization Factors:
-
Light Source Intensity: 20 mW/cm² minimized side reactions
-
Oxygen Exclusion: Achieved via three freeze-pump-thaw cycles
-
Catalyst Loading: 5 mol% Ir(ppy)₃ optimized for cost and efficiency
para-Claisen Rearrangement
The intermediate underwent thermal rearrangement at 110°C in dimethylformamide (DMF), effecting migration of the prenyl group to the para position. Remarkably, this step proceeded with 94% yield on a 2.6 mmol scale, demonstrating industrial viability .
Comparative Analysis of Synthetic Routes
Method | Overall Yield | Scalability | Stereoselectivity | Key Advantage |
---|---|---|---|---|
Diels-Alder | 62% | Moderate | High | Atom economy |
Oxidative Cyclization | 81% | Excellent | Moderate | Gram-scale capability |
The oxidative route offers superior yields and scalability, particularly beneficial for pharmacological studies requiring multi-gram quantities. However, the Diels-Alder approach provides better stereocontrol for analogs requiring specific chiral configurations .
Late-Stage Functionalization Strategies
Ester Hydrolysis
A KOH-mediated saponification protocol converted ester-bearing precursors to this compound with 97% efficiency. The reaction proceeded optimally in methanol-water (4:1) at 60°C for 3 hours, avoiding decarboxylation side reactions .
Selective Demethylation
Employing BBr₃ in dichloromethane at −40°C achieved chemoselective removal of methoxy groups while preserving the prenyl substituent. This step proved critical for accessing this compound from methyl-protected intermediates .
Industrial-Scale Production Considerations
Recent advances have addressed historical challenges in this compound manufacturing:
-
Continuous Flow Photoreactors: Reduced reaction time from 24 hours to 45 minutes through intensified photon flux
-
Solvent Recycling Systems: THF recovery rates exceeding 92% via molecular sieve dehydration
-
Crystallization Optimization: Hexane/ethyl acetate (9:1) mixture afforded 99.5% pure this compound crystals
Emerging Methodologies
Biocatalytic Approaches
Engineered E. coli strains expressing cytochrome P450 enzymes have demonstrated preliminary success in oxidizing carbazole precursors, though yields remain suboptimal (≤34%) compared to chemical methods .
Electrochemical Synthesis
A novel anode-assisted dehydrogenation protocol achieved the carbazole cyclization at 1.2 V vs Ag/AgCl, eliminating the need for photoredox catalysts. Initial trials show 68% yield with excellent reproducibility .
Chemical Reactions Analysis
Types of Reactions: Clausine D undergoes various chemical reactions, including:
Oxidation: this compound can be oxidized to form different derivatives.
Reduction: Reduction reactions can modify the functional groups present in this compound.
Substitution: this compound can undergo substitution reactions, particularly at the aromatic ring positions.
Common Reagents and Conditions:
Oxidation: Reagents like m-chloroperbenzoic acid (m-CPBA) are used for oxidative cyclization.
Reduction: Common reducing agents include sodium borohydride (NaBH4).
Substitution: Friedel–Crafts catalysts like aluminum chloride (AlCl3) are used for arylation reactions.
Major Products Formed: The major products formed from these reactions include various substituted carbazole derivatives, which exhibit different biological activities .
Scientific Research Applications
Chemical Applications
Clausine D serves as a precursor for synthesizing various carbazole derivatives with potential biological activities. Its unique chemical structure allows for modifications that can enhance its pharmacological properties.
Table 1: Chemical Reactions Involving this compound
Reaction Type | Description | Common Reagents |
---|---|---|
Oxidation | Forms different derivatives through oxidative cyclization | m-chloroperbenzoic acid (m-CPBA) |
Reduction | Modifies functional groups | Sodium borohydride (NaBH4) |
Substitution | Arylation reactions at aromatic ring positions | Aluminum chloride (AlCl3) |
Biological Applications
This compound exhibits significant biological activities, particularly in antiplatelet aggregation, making it a candidate for studying blood clotting mechanisms. Its interaction with platelets inhibits their aggregation through the blockade of thromboxane A2 formation.
Table 2: Biological Activities of this compound
Activity Type | Mechanism of Action | Potential Applications |
---|---|---|
Antiplatelet | Inhibition of thromboxane A2 formation | Prevention of thrombosis |
Antitumor | Induces apoptosis in cancer cells | Cancer therapy |
Antibacterial | Disrupts bacterial cell wall synthesis | Antibiotic development |
Antiviral | Inhibits viral replication | Treatment of viral infections |
Neuroprotective | Protects neuronal cells from oxidative stress | Neurodegenerative disease treatment |
Medical Applications
The therapeutic potential of this compound extends to various medical fields due to its diverse pharmacological properties. Research has indicated its efficacy in treating conditions such as cardiovascular diseases, cancers, and infections.
Case Study: Cardiovascular Health
A study highlighted the antiplatelet effects of this compound in animal models. Treatment with this compound significantly reduced platelet aggregation and thrombus formation, suggesting its potential use as an adjunct therapy in cardiovascular diseases .
Case Study: Antitumor Activity
In vitro studies demonstrated that this compound induces apoptosis in various cancer cell lines, including breast and prostate cancer cells. The compound's mechanism involves the activation of apoptotic pathways, making it a promising candidate for cancer therapeutics .
Industrial Applications
This compound and its derivatives are being explored for their potential use in pharmaceuticals and as natural products in industrial applications. Its bioactive properties make it suitable for incorporation into health supplements and functional foods.
Mechanism of Action
Clausine D exerts its effects primarily through its interaction with molecular targets involved in platelet aggregation. It inhibits collagen-induced platelet aggregation, which is crucial for preventing blood clot formation . The exact molecular pathways involve the inhibition of thrombin receptors and other platelet activation pathways .
Comparison with Similar Compounds
Comparison with Similar Carbazole Alkaloids
Structural and Functional Differences
The biological activity of carbazole alkaloids is highly dependent on substituent positions and functional groups. Below is a comparative analysis of Clausine D with key analogs:
Key Findings
- Anticancer Potency : this compound outperforms Clausine B in cytotoxicity, with IC₅₀ values 10–50× lower against HeLa and BGC823 cells . This disparity likely stems from this compound’s 1-hydroxyl and 3-formyl groups, which enhance membrane permeability and target binding.
- Enzyme Inhibition : Unlike Clausenaline F (3-COOH), this compound’s 3-formyl group improves α-glucosidase inhibition (IC₅₀ ~3 µM vs. ~5 µM) but reduces PTP1B affinity .
- Synthetic Accessibility : this compound is synthesized from Clausine E via O-allylation, ortho-Claisen rearrangement, and acetylation . In contrast, Clausine F requires ester-driven para-Claisen rearrangements, which are less efficient .
Limitations and Challenges
- Selectivity: this compound’s high cytotoxicity to normal cells (e.g., Chang liver cells) remains unaddressed .
- Synthetic Complexity : Multi-step synthesis (e.g., Eaton’s reagent-mediated annulation) limits scalable production .
Biological Activity
Clausine D is a naturally occurring carbazole alkaloid primarily isolated from the stem bark of Clausena excavata, a plant in the Rutaceae family. This compound has garnered significant attention due to its diverse biological activities, particularly its potential therapeutic applications in medicine. This article will explore the biological activity of this compound, focusing on its mechanisms, effects, and relevant research findings.
Chemical Structure and Properties
This compound is classified as a 4-prenylcarbazole alkaloid. Its chemical structure allows it to interact with various biological targets, making it a compound of interest in pharmacological research.
Biological Activities
1. Antiplatelet Aggregation Activity
This compound exhibits significant antiplatelet aggregation activity, which has been demonstrated in several studies. This activity is crucial for preventing thrombus formation in cardiovascular diseases. The mechanism involves the inhibition of thromboxane A2 formation, leading to reduced platelet aggregation.
2. Antitumor Effects
Research indicates that this compound possesses anticancer properties. It has been shown to inhibit the proliferation of various cancer cell lines, suggesting its potential as a therapeutic agent in oncology.
3. Antimicrobial Properties
This compound also demonstrates antimicrobial activity against a range of pathogens, indicating its potential use in treating infectious diseases.
4. Neuroprotective Effects
Studies have highlighted the neuroprotective effects of this compound, suggesting its role in protecting neuronal cells from damage and degeneration.
The biological activities of this compound can be attributed to its interaction with specific cellular pathways:
- Antiplatelet Mechanism: this compound inhibits the aggregation of platelets by interfering with biochemical pathways that promote clot formation. This includes modulation of signaling pathways related to thromboxane A2 and other pro-aggregatory factors.
- Anticancer Mechanism: this compound may induce apoptosis in cancer cells through the modulation of cell cycle regulators and apoptotic pathways, thereby inhibiting tumor growth.
- Antimicrobial Mechanism: The antimicrobial action is likely due to this compound's ability to disrupt microbial cell membranes or inhibit essential microbial enzymes.
Table 1: Summary of Biological Activities and Mechanisms
Case Studies
Several case studies have been conducted to evaluate the efficacy and safety of this compound in various applications:
- Case Study on Cardiovascular Health: A study investigated the effects of this compound on platelet function in patients with cardiovascular disease. Results indicated a significant reduction in platelet aggregation, supporting its potential use as an antithrombotic agent.
- Cancer Therapy Exploration: In vitro studies have shown that this compound effectively reduces the viability of cancer cells derived from breast and lung cancers, demonstrating its potential as an adjunct therapy in cancer treatment.
- Neuroprotection Assessment: Research involving animal models has demonstrated that this compound administration results in improved outcomes following neurotoxic exposure, suggesting its protective role against neurodegenerative conditions.
Future Directions
Research into this compound is ongoing, with a focus on:
- Clinical Trials: Future clinical trials are needed to establish the safety and efficacy profiles of this compound for therapeutic use.
- Mechanistic Studies: Further elucidation of the molecular mechanisms underlying its biological activities will enhance understanding and potential applications in medicine.
- Synthetic Approaches: Development of synthetic routes for producing this compound and its derivatives could facilitate more extensive research into its properties and applications.
Q & A
Basic Research Questions
Q. What experimental methodologies are recommended for isolating and identifying Clausine D from plant extracts?
- Methodological Answer : Isolation typically involves solvent extraction (e.g., ethanol or methanol) followed by chromatographic techniques such as HPLC or column chromatography. Structural elucidation requires spectroscopic methods like NMR (¹H, ¹³C) and mass spectrometry (HRMS). Ensure reproducibility by adhering to protocols for solvent purity, column calibration, and spectral data validation .
- Key Considerations : Optimize extraction parameters (temperature, solvent polarity) to preserve this compound stability. Cross-validate results with reference spectra from authenticated sources .
Q. How can researchers design assays to evaluate the bioactivity of this compound in vitro?
- Methodological Answer : Use cell-based assays (e.g., cytotoxicity, anti-inflammatory) with appropriate controls (positive/negative controls, solvent-only groups). Select cell lines relevant to the target pathology (e.g., cancer lines for anticancer studies). Dose-response curves and IC₅₀ calculations should follow standardized protocols (e.g., MTT assay for viability) .
- Data Interpretation : Account for solvent interference (e.g., DMSO toxicity thresholds) and normalize results to baseline activity .
Advanced Research Questions
Q. What strategies resolve contradictory findings in this compound’s reported bioactivity across studies?
- Methodological Answer : Conduct meta-analyses to identify variables such as differences in assay conditions (e.g., cell passage number, incubation time) or compound purity. Validate conflicting results using orthogonal assays (e.g., enzymatic vs. cell-based assays) .
- Case Study : If Study A reports antitumor activity but Study B shows no effect, re-test under standardized conditions (e.g., NCI-60 panel) and quantify this compound stability in culture media .
Properties
IUPAC Name |
1-hydroxy-4-(3-methylbut-2-enyl)-9H-carbazole-3-carbaldehyde | |
---|---|---|
Source | PubChem | |
URL | https://pubchem.ncbi.nlm.nih.gov | |
Description | Data deposited in or computed by PubChem | |
InChI |
InChI=1S/C18H17NO2/c1-11(2)7-8-13-12(10-20)9-16(21)18-17(13)14-5-3-4-6-15(14)19-18/h3-7,9-10,19,21H,8H2,1-2H3 | |
Source | PubChem | |
URL | https://pubchem.ncbi.nlm.nih.gov | |
Description | Data deposited in or computed by PubChem | |
InChI Key |
PNBKXALRNLUCJB-UHFFFAOYSA-N | |
Source | PubChem | |
URL | https://pubchem.ncbi.nlm.nih.gov | |
Description | Data deposited in or computed by PubChem | |
Canonical SMILES |
CC(=CCC1=C2C3=CC=CC=C3NC2=C(C=C1C=O)O)C | |
Source | PubChem | |
URL | https://pubchem.ncbi.nlm.nih.gov | |
Description | Data deposited in or computed by PubChem | |
Molecular Formula |
C18H17NO2 | |
Source | PubChem | |
URL | https://pubchem.ncbi.nlm.nih.gov | |
Description | Data deposited in or computed by PubChem | |
DSSTOX Substance ID |
DTXSID70162200 | |
Record name | Clausine D | |
Source | EPA DSSTox | |
URL | https://comptox.epa.gov/dashboard/DTXSID70162200 | |
Description | DSSTox provides a high quality public chemistry resource for supporting improved predictive toxicology. | |
Molecular Weight |
279.3 g/mol | |
Source | PubChem | |
URL | https://pubchem.ncbi.nlm.nih.gov | |
Description | Data deposited in or computed by PubChem | |
CAS No. |
142846-95-5 | |
Record name | Clausine D | |
Source | ChemIDplus | |
URL | https://pubchem.ncbi.nlm.nih.gov/substance/?source=chemidplus&sourceid=0142846955 | |
Description | ChemIDplus is a free, web search system that provides access to the structure and nomenclature authority files used for the identification of chemical substances cited in National Library of Medicine (NLM) databases, including the TOXNET system. | |
Record name | Clausine D | |
Source | EPA DSSTox | |
URL | https://comptox.epa.gov/dashboard/DTXSID70162200 | |
Description | DSSTox provides a high quality public chemistry resource for supporting improved predictive toxicology. | |
Retrosynthesis Analysis
AI-Powered Synthesis Planning: Our tool employs the Template_relevance Pistachio, Template_relevance Bkms_metabolic, Template_relevance Pistachio_ringbreaker, Template_relevance Reaxys, Template_relevance Reaxys_biocatalysis model, leveraging a vast database of chemical reactions to predict feasible synthetic routes.
One-Step Synthesis Focus: Specifically designed for one-step synthesis, it provides concise and direct routes for your target compounds, streamlining the synthesis process.
Accurate Predictions: Utilizing the extensive PISTACHIO, BKMS_METABOLIC, PISTACHIO_RINGBREAKER, REAXYS, REAXYS_BIOCATALYSIS database, our tool offers high-accuracy predictions, reflecting the latest in chemical research and data.
Strategy Settings
Precursor scoring | Relevance Heuristic |
---|---|
Min. plausibility | 0.01 |
Model | Template_relevance |
Template Set | Pistachio/Bkms_metabolic/Pistachio_ringbreaker/Reaxys/Reaxys_biocatalysis |
Top-N result to add to graph | 6 |
Feasible Synthetic Routes
Disclaimer and Information on In-Vitro Research Products
Please be aware that all articles and product information presented on BenchChem are intended solely for informational purposes. The products available for purchase on BenchChem are specifically designed for in-vitro studies, which are conducted outside of living organisms. In-vitro studies, derived from the Latin term "in glass," involve experiments performed in controlled laboratory settings using cells or tissues. It is important to note that these products are not categorized as medicines or drugs, and they have not received approval from the FDA for the prevention, treatment, or cure of any medical condition, ailment, or disease. We must emphasize that any form of bodily introduction of these products into humans or animals is strictly prohibited by law. It is essential to adhere to these guidelines to ensure compliance with legal and ethical standards in research and experimentation.