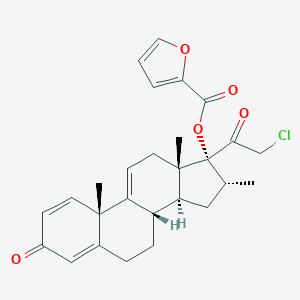
Mometasone Furoate Impurity A
Overview
Description
Mometasone Furoate Impurity A is a structurally related compound of the corticosteroid drug mometasone furoate, which is widely used for its anti-inflammatory and immunosuppressive properties. Impurity A is identified as a process-related or degradation impurity during the synthesis or storage of mometasone furoate. The European Pharmacopoeia (EP) monograph for mometasone furoate lists known impurities, including Impurity A, which must be controlled within strict limits (≤0.15% per ICH Q3B(R2) guidelines) to ensure drug safety and efficacy .
Preparation Methods
Chlorination and Furoylation Pathways
Dichloro-Hydantoin-Mediated Chlorination
The most direct method for generating Mometasone Furoate Impurity A involves the chlorination of a precursor steroid structure using dichloro-hydantoin (DCH) in a ketone solvent. As detailed in the ChemicalBook synthesis route, 45 g of the precursor (compound V) is dissolved in 180 mL of methyl ethyl ketone (MEK) at -50°C, followed by the addition of 5 mL glacial acetic acid and 36 g DCH in four equal portions . The reaction proceeds at -50°C for 3 hours, achieving a mass yield of 102.2% and a purity of 97.5% after workup . This method leverages the electrophilic chlorination properties of DCH, which selectively targets the 21-position of the steroid backbone, a critical step in forming Impurity A .
Key parameters:
-
Temperature : -50°C to 0°C
-
Solvent : Methyl ethyl ketone
-
Catalyst : Glacial acetic acid
-
Workup : Sodium sulfite quenching, solvent concentration, and recrystallization in dichloromethane-methanol .
Lithium Chloride-Catalyzed Furoic Acylation
Patent CN106636289A describes a furoic acylation step using lithium chloride (LiCl) as a catalyst, which may indirectly relate to Impurity A formation . In this process, compound II (a dehydrogenated intermediate) reacts with furoyl chloride in dichloromethane or tetrahydrofuran (THF) at 0–50°C, facilitated by organic bases such as DMAP or triethylamine . While the patent focuses on mometasone furoate synthesis, incomplete acylation or side reactions during this step could theoretically yield Impurity A as a byproduct. For instance, suboptimal stoichiometry of furoyl chloride or premature termination of the reaction might leave unreacted intermediates that match Impurity A’s structure .
One-Pot Synthesis and Impurity Formation
Hydrochloric Acid-Glacial Acetic Acid Ring Opening
The same patent highlights a ring-opening step using a mixture of concentrated hydrochloric acid and glacial acetic acid . Under controlled conditions (0–30°C), this step converts acylated intermediates into mometasone furoate. However, variations in acid concentration or reaction time may result in partial ring opening, leaving behind intermediates structurally analogous to Impurity A .
Fermentation-Dehydrogenation Routes
Microbial Dehydrogenation of Steroid Precursors
Patent CN106636289A employs microbial fermentation to dehydrogenate compound I (9α-hydroxy-16α-methylprednisolone) into compound II, a step critical for subsequent acylation . The fermentation medium comprises yeast extract, peptone, and glucose, with pH adjusted to 7.2 using sodium hydroxide . While this step primarily targets compound II, microbial side reactions or incomplete dehydrogenation could yield derivatives that align with Impurity A’s chemical profile .
Impact of Catalysts on Intermediate Purity
The use of zinc sulfate or magnesium sulfate as co-catalysts in the fermentation step enhances reaction efficiency but may also influence the distribution of byproducts . For instance, magnesium sulfate’s Lewis acidity could promote alternative dehydrogenation pathways, increasing the likelihood of Impurity A formation under specific conditions .
Comparative Analysis of Synthetic Methods
Optimization Strategies for Impurity A Isolation
Solvent Selection and Recrystallization
The ChemicalBook method employs a dichloromethane-methanol system for recrystallization, effectively removing polar byproducts and enhancing Impurity A’s purity to 97.5% . Similarly, patent CN106636289A utilizes tetrahydrofuran and benzene in later stages, which could be adapted to isolate Impurity A through fractional crystallization .
Chemical Reactions Analysis
Mometasone Furoate Impurity A undergoes various chemical reactions, including:
Oxidation: This reaction can lead to the formation of additional oxygen-containing functional groups.
Reduction: This process can reduce ketone or aldehyde groups to alcohols.
Substitution: Halogen atoms in the compound can be substituted with other functional groups under appropriate conditions. Common reagents used in these reactions include oxidizing agents like potassium permanganate, reducing agents such as sodium borohydride, and nucleophiles for substitution reactions. The major products formed depend on the specific reaction conditions and reagents used.
Scientific Research Applications
Toxicological assessments are crucial for understanding the safety profile of drugs containing mometasone furoate. Mometasone Furoate Impurity A is often included in nonclinical toxicology studies to evaluate its effects alongside the active pharmaceutical ingredient. These studies help in identifying any potential additive or synergistic effects when combined with other compounds, such as formoterol fumarate.
Key findings from toxicology studies include:
- Histopathological Analysis : Evaluations show significant changes in lymphoid tissues and organs in animal models exposed to mometasone furoate and its impurities .
- Dose-Response Relationships : Studies indicate that various doses of mometasone furoate lead to observable adverse effects, emphasizing the need for thorough impurity characterization .
Pharmaceutical Formulation
This compound plays a role in the formulation of corticosteroid medications. Understanding its properties aids in optimizing drug formulations to enhance stability and therapeutic efficacy. This impurity must be monitored during the manufacturing process to ensure that it remains within acceptable limits as specified by regulatory bodies.
Regulatory Compliance
The presence of impurities like this compound is subject to strict regulatory scrutiny. Regulatory agencies require comprehensive data on impurities for drug approval processes. This includes:
- Characterization : Detailed chemical characterization using techniques such as mass spectrometry and nuclear magnetic resonance (NMR) spectroscopy.
- Quantification : Establishing acceptable limits for impurities through validated analytical methods.
Case Study 1: Multi-Gram Scale Synthesis
A recent study reported the synthesis of this compound on a multi-gram scale, highlighting its importance in toxicological studies and method development . The study emphasized the need for high-purity standards to facilitate accurate analytical assessments.
Case Study 2: Toxicological Assessment
In a toxicological study involving rats and dogs, researchers examined the effects of mometasone furoate and its impurities on various organ systems. The findings indicated significant adverse effects linked to both the active ingredient and its impurities, underscoring the importance of thorough impurity analysis .
Mechanism of Action
As an impurity, Mometasone Furoate Impurity A does not have a direct mechanism of action like the parent compound, mometasone furoate. understanding its formation and behavior is essential for ensuring the overall efficacy and safety of the pharmaceutical product. The impurity can interact with the same molecular targets as mometasone furoate, potentially affecting the drug’s pharmacokinetics and pharmacodynamics .
Comparison with Similar Compounds
Structural Features
Mometasone furoate and its impurities share a common pregnane backbone but differ in substituents and functional groups:
Note: Impurity A’s structure is inferred from synthesis pathways, where intermediates or by-products may lack esterification (e.g., 21-acetoxy derivatives) or exhibit incomplete chlorination .
Physicochemical Properties
Key differences in log P, pKa, and solubility influence analytical separation and toxicity:
- Log P : Mometasone furoate has a higher log P (~3.5) due to its lipophilic furoate ester, whereas impurities like G (log P ~2.5) and A (estimated log P ~2.0–2.8) are less lipophilic .
- pKa : Impurities with hydroxyl or carboxylic acid groups (e.g., degradation products) may exhibit pKa values between 7.0 and 9.0, affecting ionization under chromatographic conditions .
Analytical Methods
Orthogonal methods are required to resolve structurally similar impurities:
- RP-HPLC : Used for Impurity A quantification in nasal spray formulations, with retention times (RT) varying by <1 minute compared to mometasone furoate .
- SFC : Provides superior resolution for polar impurities (e.g., Impurity G) due to its compatibility with acidic mobile phases and CO₂-based gradients .
- Hyphenated Techniques: LC-MS and LC-NMR aid in structural elucidation, particularly for unknown degradation products .
Regulatory and Toxicity Profiles
Parameter | Mometasone Furoate | Impurity A | Impurity G |
---|---|---|---|
ICH Q3B Limit | N/A (API) | ≤0.15% | ≤0.10% |
Ecotoxicity (LC₅₀) | 0.11 mg/L (fish) | Not reported | Not reported |
Synthetic Origin | Final product | Process intermediate | Degradation product |
Regulatory thresholds for Impurity A align with ICH guidelines, but its toxicity profile remains less studied compared to mometasone furoate, which shows moderate aquatic toxicity .
Key Research Findings
- Synthetic Pathways : Impurity A likely forms during incomplete esterification or chlorination steps, as seen in intermediates like 21-acetoxy-17α-hydroxy-16α-methyl-pregna derivatives .
- Chromatographic Challenges : Impurity A co-elutes with other impurities in RP-HPLC, necessitating SFC or HILIC for baseline separation .
- Stability : Impurity A is stable under accelerated storage conditions (40°C/75% RH), but oxidative degradation may generate secondary impurities .
Biological Activity
Mometasone furoate (MF) is a synthetic corticosteroid widely used for its anti-inflammatory properties in treating various conditions, including asthma, allergic rhinitis, and skin disorders. However, its impurities, particularly Mometasone Furoate Impurity A, have garnered attention regarding their biological activity and potential effects on pharmacokinetics and pharmacodynamics. This article delves into the biological activity of this compound, supported by data tables, case studies, and detailed research findings.
Overview of this compound
This compound is one of the several impurities that can arise during the synthesis of mometasone furoate. Understanding the biological activity of this impurity is critical for assessing the safety and efficacy of formulations containing mometasone furoate. The impurity's structure and metabolic profile may influence its pharmacological effects and interactions with glucocorticoid receptors.
Biological Activity
Mechanism of Action:
Mometasone furoate exerts its effects primarily through glucocorticoid receptor (GR) activation, leading to the modulation of inflammatory pathways. The affinity for GR is significantly higher than that of other corticosteroids, which suggests that both MF and its impurities can exhibit similar or altered biological activities depending on their receptor binding affinities.
Metabolism:
Research indicates that mometasone furoate undergoes extensive hepatic metabolism via cytochrome P450 enzymes, primarily CYP3A4. This metabolic pathway generates several metabolites, some of which may retain biological activity. For instance, in studies involving rat and human liver S9 fractions, it was found that both MF and its metabolites exhibited varying degrees of glucocorticoid receptor binding affinity .
Comparative Biological Activity
Compound | Relative Binding Affinity (RBA) | Biological Activity |
---|---|---|
Mometasone Furoate | 2900 | High anti-inflammatory activity |
This compound | Unknown | Potentially lower than MF |
MET1 (major metabolite) | Varies | Active metabolite |
MET2 | 700 | Moderate activity |
Case Studies
-
Topical Application Studies:
Clinical trials have demonstrated that topical formulations containing mometasone furoate are well tolerated with minimal side effects. In one study involving healthy volunteers applying a 0.1% cream for 12 months, no significant atrophogenic effects were observed . This suggests that even impurities present in low concentrations do not significantly compromise safety. -
Inhalation Studies:
Inhaled mometasone furoate has been shown to effectively reduce symptoms in asthma patients. The systemic absorption of the drug is low (<1%), indicating that impurities like this compound may have limited systemic exposure and thus reduced potential for adverse effects . However, the cumulative effects of impurities in chronic use scenarios warrant further investigation. -
Nasal Spray Efficacy:
Mometasone furoate nasal spray has been effective in treating nasal polyps, significantly improving symptoms and quality of life in patients . The role of impurities in this formulation remains to be fully elucidated but is critical for understanding long-term safety.
Research Findings
Recent studies emphasize the need for rigorous impurity testing in pharmaceutical formulations. Analytical methods such as high-performance liquid chromatography (HPLC) and supercritical fluid chromatography (SFC) are essential for quantifying impurities like this compound . The development of sensitive methods ensures that any potential biological activity associated with these impurities is adequately assessed.
Q & A
Basic Research Questions
Q. What analytical methods are validated for quantifying Mometasone Furoate Impurity A in pharmaceutical formulations?
A reverse-phase high-performance liquid chromatography (RP-HPLC) method with UV/PDA detection is widely used. The method employs a Bakerbond C18 column (250 × 4.6 mm, 5 μm) and a mobile phase of octane sulfonate sodium salt in phosphate buffer (pH 3.0) and acetonitrile (45:55 v/v) at 0.8 mL/min. Validation per ICH guidelines confirmed linearity (0.1–1.5 µg/mL), precision (RSD < 2%), and robustness. This method is stability-indicating, with forced degradation studies showing ≤12.4% degradation under alkaline stress .
Q. What are the key physicochemical parameters required to characterize this compound?
Key parameters include:
- CAS Number : 83880-65-3
- Molecular Formula : CHClO
- Molecular Weight : 468.97 g/mol Structural characterization via spectroscopic techniques (e.g., NMR, MS) and chromatographic retention time matching against reference standards are critical for identification .
Q. How does TLC compare to HPLC for identifying this compound in drug products?
Thin-layer chromatography (TLC) is a qualitative screening tool but lacks the precision and sensitivity of HPLC. For example, TLC methods in cream formulations rely on retention factor (R) matching, whereas HPLC provides quantitative data with resolution >2.0 between Impurity A and the active pharmaceutical ingredient (API) .
Advanced Research Questions
Q. How can orthogonal methods like supercritical fluid chromatography (SFC) enhance impurity profiling of this compound?
SFC coupled with mass spectrometry offers complementary selectivity to RP-HPLC, particularly for polar degradation products. A study demonstrated baseline separation of Impurity A from structurally similar impurities using a chiral stationary phase and CO-methanol mobile phase, achieving a detection limit of 0.05 µg/mL. This is critical for resolving co-eluting peaks in stressed samples .
Q. What experimental design principles apply to forced degradation studies for Impurity A?
Follow the ICH Q1A(R2) and Q2(R1) guidelines:
- Stress Conditions : Acid (0.1 N HCl), base (0.1 N NaOH), oxidative (3% HO), thermal (80°C), and photolytic (1.2 million lux-hours).
- Endpoint Criteria : ≥10% degradation of the API to ensure impurity formation.
- Specificity Testing : Demonstrate resolution of Impurity A from degradation products (e.g., diastereomers like Impurity J, CAS 1370190-55-8) .
Q. How can the FINER framework guide research on Impurity A's synthetic pathway?
- Feasible : Use scalable synthetic routes (e.g., esterification of mometasone with furoyl chloride).
- Novel : Investigate undocumented intermediates (e.g., 11,17-difuroate derivatives).
- Ethical : Adhere to green chemistry principles for solvent selection.
- Relevant : Align with pharmacopeial limits (e.g., USP/EP thresholds of 0.15% for specified impurities) .
Q. What strategies ensure method specificity when Impurity A co-elutes with structurally related impurities (e.g., Impurity E)?
- Column Screening : Test polar-embedded (e.g., ACE C18-AR) and HILIC columns.
- MS Detection : Use high-resolution mass spectrometry (HRMS) to differentiate isotopic patterns (e.g., Impurity E: CHClO vs. Impurity A: CHClO).
- Spiking Studies : Confirm recovery of individual impurities in spiked API samples .
Q. How can degradation kinetics data resolve contradictions in impurity stability profiles?
For example, if Impurity A shows inconsistent formation under thermal stress:
Properties
IUPAC Name |
[(8S,10S,13S,14S,16R,17R)-17-(2-chloroacetyl)-10,13,16-trimethyl-3-oxo-7,8,12,14,15,16-hexahydro-6H-cyclopenta[a]phenanthren-17-yl] furan-2-carboxylate | |
---|---|---|
Source | PubChem | |
URL | https://pubchem.ncbi.nlm.nih.gov | |
Description | Data deposited in or computed by PubChem | |
InChI |
InChI=1S/C27H29ClO5/c1-16-13-21-19-7-6-17-14-18(29)8-10-25(17,2)20(19)9-11-26(21,3)27(16,23(30)15-28)33-24(31)22-5-4-12-32-22/h4-5,8-10,12,14,16,19,21H,6-7,11,13,15H2,1-3H3/t16-,19-,21+,25+,26+,27+/m1/s1 | |
Source | PubChem | |
URL | https://pubchem.ncbi.nlm.nih.gov | |
Description | Data deposited in or computed by PubChem | |
InChI Key |
GSJLBVBYMSNTPZ-XACSLSAESA-N | |
Source | PubChem | |
URL | https://pubchem.ncbi.nlm.nih.gov | |
Description | Data deposited in or computed by PubChem | |
Canonical SMILES |
CC1CC2C3CCC4=CC(=O)C=CC4(C3=CCC2(C1(C(=O)CCl)OC(=O)C5=CC=CO5)C)C | |
Source | PubChem | |
URL | https://pubchem.ncbi.nlm.nih.gov | |
Description | Data deposited in or computed by PubChem | |
Isomeric SMILES |
C[C@@H]1C[C@H]2[C@@H]3CCC4=CC(=O)C=C[C@@]4(C3=CC[C@@]2([C@]1(C(=O)CCl)OC(=O)C5=CC=CO5)C)C | |
Source | PubChem | |
URL | https://pubchem.ncbi.nlm.nih.gov | |
Description | Data deposited in or computed by PubChem | |
Molecular Formula |
C27H29ClO5 | |
Source | PubChem | |
URL | https://pubchem.ncbi.nlm.nih.gov | |
Description | Data deposited in or computed by PubChem | |
Molecular Weight |
469.0 g/mol | |
Source | PubChem | |
URL | https://pubchem.ncbi.nlm.nih.gov | |
Description | Data deposited in or computed by PubChem | |
CAS No. |
83880-65-3 | |
Record name | 21-Chloro-16alpha-methyl-3,20-dioxopregna-1,4,9(11)-trien-17-yl furan-2-carboxylate | |
Source | ChemIDplus | |
URL | https://pubchem.ncbi.nlm.nih.gov/substance/?source=chemidplus&sourceid=0083880653 | |
Description | ChemIDplus is a free, web search system that provides access to the structure and nomenclature authority files used for the identification of chemical substances cited in National Library of Medicine (NLM) databases, including the TOXNET system. | |
Record name | 21-CHLORO-16.ALPHA.-METHYL-3,20-DIOXOPREGNA-1,4,9(11)-TRIEN-17-YL FURAN-2-CARBOXYLATE | |
Source | FDA Global Substance Registration System (GSRS) | |
URL | https://gsrs.ncats.nih.gov/ginas/app/beta/substances/M2A68F897F | |
Description | The FDA Global Substance Registration System (GSRS) enables the efficient and accurate exchange of information on what substances are in regulated products. Instead of relying on names, which vary across regulatory domains, countries, and regions, the GSRS knowledge base makes it possible for substances to be defined by standardized, scientific descriptions. | |
Explanation | Unless otherwise noted, the contents of the FDA website (www.fda.gov), both text and graphics, are not copyrighted. They are in the public domain and may be republished, reprinted and otherwise used freely by anyone without the need to obtain permission from FDA. Credit to the U.S. Food and Drug Administration as the source is appreciated but not required. | |
Disclaimer and Information on In-Vitro Research Products
Please be aware that all articles and product information presented on BenchChem are intended solely for informational purposes. The products available for purchase on BenchChem are specifically designed for in-vitro studies, which are conducted outside of living organisms. In-vitro studies, derived from the Latin term "in glass," involve experiments performed in controlled laboratory settings using cells or tissues. It is important to note that these products are not categorized as medicines or drugs, and they have not received approval from the FDA for the prevention, treatment, or cure of any medical condition, ailment, or disease. We must emphasize that any form of bodily introduction of these products into humans or animals is strictly prohibited by law. It is essential to adhere to these guidelines to ensure compliance with legal and ethical standards in research and experimentation.