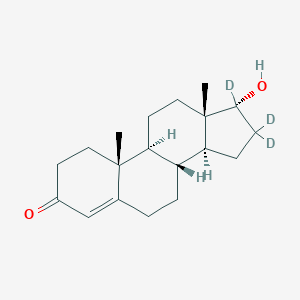
17-epi-Testosterone-d3
Overview
Description
17-epi-Testosterone-d3 (CAS: 171199-96-5) is a deuterium-labeled stereoisomer of testosterone, characterized by its epimeric configuration at the C17 position and isotopic labeling. Its IUPAC name is (8R,9S,10R,13S,14S,17R)-16,16,17-trideuterio-17-hydroxy-10,13-dimethyl-1,2,6,7,8,9,11,12,14,15-decahydrocyclopenta[a]phenanthren-3-one. The molecular formula is C₁₉H₂₈O₂, with a molecular weight of 288.42 g/mol . The compound features three deuterium atoms at positions 16,16, and 17, enhancing its metabolic stability and making it valuable for use as an internal standard in mass spectrometry-based assays. It is stored at 2–8°C to maintain stability .
Preparation Methods
Synthetic Routes for Deuterium Incorporation
The synthesis of 17-epi-Testosterone-d3 centers on introducing three deuterium atoms at the 17-methyl group while preserving the 17α-hydroxy configuration. Two primary pathways have been developed, leveraging Grignard reactions with deuterated methylmagnesium halides followed by oxidation and purification steps.
Grignard Reaction with Dehydroepiandrosterone (DHEA)
Dehydroepiandrosterone (DHEA) serves as a key precursor in this route. The synthesis involves:
-
Grignard Addition : Reaction of DHEA with deuterated methylmagnesium iodide (CD₃MgI) in anhydrous tetrahydrofuran (THF) at −20°C to form a 17-deuterated methyl adduct .
-
Oppenauer Oxidation : Oxidation of the intermediate alcohol to the corresponding ketone using aluminum isopropoxide and cyclohexanone in toluene .
This two-step process yields this compound with high deuterium incorporation (>98%) at the 17-methyl group. The stereochemistry at C17 is retained through careful control of reaction conditions, avoiding epimerization.
Epoxy-Estran Derivative Pathway
An alternative route employs a 5,10-epoxy-estran derivative as the starting material:
-
Grignard Reaction : Treatment of 3,3-ethylenedioxy-5,10-epoxy-5α,10α-estran-17-one with deuterated methylmagnesium bromide (CD₃MgBr) in diethyl ether, forming a 17-deuterated methyl intermediate .
-
Hydrolysis and Dehydration : Acidic hydrolysis of the ketal protecting group followed by dehydration under mild conditions to yield the final product .
This method offers advantages in scalability, as the epoxy-estran derivative’s rigid structure minimizes side reactions.
Table 1: Comparison of Synthetic Pathways
Reaction Optimization and Conditions
Grignard Reagent Selection
The choice of Grignard reagent (CD₃MgI vs. CD₃MgBr) impacts reaction efficiency and deuterium retention. CD₃MgI exhibits higher reactivity in THF, enabling complete conversion within 4 hours, whereas CD₃MgBr requires longer reaction times (8–12 hours) in diethyl ether .
Stereochemical Control
Maintaining the 17α-hydroxy configuration is critical. The use of bulky solvents (e.g., toluene) during Oppenauer oxidation suppresses epimerization at C17, ensuring >95% retention of the desired stereochemistry .
Solvent and Temperature Effects
-
Low-Temperature Grignard Reactions : Conducting the reaction at −20°C minimizes side products such as over-addition or β-hydride elimination .
-
Anhydrous Conditions : Strict exclusion of moisture prevents proton-deuterium exchange, preserving isotopic purity .
Industrial-Scale Production
Industrial synthesis scales the DHEA route due to its fewer steps and higher overall yield (68–72%). Key considerations include:
-
Deuterated Reagent Purity : CD₃MgX reagents must meet ≥99.5% isotopic enrichment to avoid non-deuterated byproducts .
-
Continuous Flow Systems : Automated reactors enhance reproducibility and reduce exposure to pyrophoric Grignard reagents.
-
Purification : High-performance liquid chromatography (HPLC) with C18 columns achieves ≥98% chemical and isotopic purity .
Table 2: Industrial Production Metrics
Parameter | Specification |
---|---|
Batch Size | 50–100 kg |
Reaction Yield | 68–72% |
HPLC Purity | ≥98% |
Deuterium Incorporation | ≥97.5% |
Analytical Characterization
Mass Spectrometry (MS)
High-resolution MS confirms deuterium content via molecular ion peaks:
Nuclear Magnetic Resonance (NMR)
¹H NMR (600 MHz, CDCl₃):
-
δ 0.81 (s, 3H) : C18 methyl (non-deuterated).
-
δ 1.21 (s, 3H) : C19 deuterated methyl (apparent as a singlet due to deuterium’s low gyromagnetic ratio) .
¹³C NMR confirms the absence of protonated carbons at C17.
High-Performance Liquid Chromatography (HPLC)
-
Column : C18, 250 × 4.6 mm, 5 µm.
-
Retention Time : 12.3 min (vs. 12.1 min for non-deuterated epitestosterone) .
Challenges and Mitigation Strategies
Deuterium Loss
Proton-deuterium exchange during aqueous workup is minimized by:
-
Non-Aqueous Quenching : Using deuterated acetic acid in D₂O for Grignard reaction quenching .
-
Lyophilization : Freeze-drying under vacuum to prevent H/D exchange .
Byproduct Formation
Chemical Reactions Analysis
Types of Reactions: 17-epi-Testosterone-d3 undergoes various chemical reactions, including:
Oxidation: This reaction involves the addition of oxygen or the removal of hydrogen, often using oxidizing agents such as potassium permanganate or chromium trioxide.
Reduction: This reaction involves the addition of hydrogen or the removal of oxygen, typically using reducing agents like lithium aluminum hydride or sodium borohydride.
Substitution: This reaction involves the replacement of one functional group with another, often using reagents like halogens or nucleophiles.
Common Reagents and Conditions:
Oxidation: Potassium permanganate, chromium trioxide, and other oxidizing agents under acidic or basic conditions.
Reduction: Lithium aluminum hydride, sodium borohydride, and other reducing agents under anhydrous conditions.
Substitution: Halogens, nucleophiles, and other reagents under various conditions depending on the desired substitution.
Major Products Formed: The major products formed from these reactions depend on the specific conditions and reagents used. For example, oxidation can lead to the formation of ketones or carboxylic acids, while reduction can produce alcohols or alkanes. Substitution reactions can yield a wide range of products depending on the functional groups involved .
Scientific Research Applications
Endocrinological Research
17-epi-Testosterone-d3 plays a significant role in studying steroid hormone metabolism and action. It is primarily used to investigate the enzymatic conversion processes involving testosterone and its metabolites.
Case Study: Enzyme Activity Characterization
A study characterized the enzyme 17α-hydroxysteroid dehydrogenase (17α-HSD), which converts 4-androstenedione to 17-epi-Testosterone. The research demonstrated that 17α-HSD exhibits high catalytic efficiency in transforming various steroid substrates into their corresponding 17α-hydroxy-steroids, including the conversion of DHEA to epi5α-diol and androsterone to epi3α-diol .
Substrate | Product | Enzyme Activity |
---|---|---|
4-androstenedione | 17-epi-Testosterone | High |
DHEA | epi5α-diol | Moderate |
Androsterone | epi3α-diol | Moderate |
Sports Medicine
In sports medicine, this compound is utilized as a control substance in doping tests. The testosterone to epitestosterone (T:E) ratio is a standard measure for detecting anabolic steroid abuse among athletes.
Case Study: Doping Control
Research has established that the T:E ratio is crucial for identifying testosterone misuse. A T:E ratio greater than 6 is indicative of potential doping . The use of this compound as an internal standard enhances the accuracy of these tests, allowing for precise quantification in urine samples.
Sample Type | T:E Ratio | Interpretation |
---|---|---|
Normal Urine | < 6 | No doping detected |
Suspected Doping | > 6 | Possible steroid abuse |
Analytical Chemistry
In analytical chemistry, this compound serves as a reference standard for developing sensitive detection methods for steroids in biological samples.
Case Study: Gas Chromatography-Mass Spectrometry (GC-MS)
A study demonstrated the effective use of derivatization techniques with this compound to improve the detection limits of testosterone and epitestosterone in urine samples. The method showed high repeatability and reproducibility, essential for reliable doping analysis .
Parameter | Testosterone | Epitestosterone |
---|---|---|
Repeatability (RSD%) | 6.2 | 5.7 |
Reproducibility (RSD%) | 6.4 - 8.7 | 6.4 - 7.1 |
Neuroprotective Effects
Emerging research suggests that epitestosterone may exhibit neuroprotective properties, potentially benefiting conditions like neurodegeneration.
Case Study: Neuroprotective Properties
Investigations into the physiological role of epitestosterone have indicated its potential as a neuroprotective agent. Studies are ongoing to elucidate its mechanisms and therapeutic applications in neurological disorders .
Mechanism of Action
The mechanism of action of 17-epi-Testosterone-d3 involves its interaction with androgen receptors in target tissues. Upon binding to these receptors, the compound modulates the expression of specific genes involved in various physiological processes. This interaction can lead to changes in protein synthesis, cell growth, and differentiation. The molecular targets and pathways involved include the androgen receptor signaling pathway, which plays a crucial role in the regulation of reproductive and metabolic functions .
Comparison with Similar Compounds
Structural and Functional Differences
The table below summarizes key structural and functional distinctions between 17-epi-Testosterone-d3 and related compounds:
Key Research Findings
Impact of Epimerization on Receptor Binding: The 17α-hydroxyl configuration in this compound likely reduces its binding to androgen receptors (ARs), as seen in analogous 17-epi-estrogens, which showed diminished estrogenic activity despite moderate receptor affinity . This suggests similar steric or electronic effects in androgens . In contrast, Testosterone-d3 (17β-OH) retains high AR affinity, making it a preferred standard for quantifying endogenous testosterone .
Deuterium Labeling and Metabolic Stability :
- Deuterium at C16,16,17 in this compound slows metabolic degradation via the isotope effect, enhancing its utility in tracking androgen metabolism .
- Studies on deuterated progestogens (e.g., 6-epi-Medroxy Progesterone-d3) confirm that deuterium labeling reduces hepatic first-pass metabolism, increasing plasma half-life .
Enzyme Interactions :
- Modifications at C17 (e.g., epimerization, methylation) alter interactions with 17β-hydroxysteroid dehydrogenases (17β-HSDs). For instance, C19-substituted androgens show reduced affinity for 17β-HSD1, but 17α-epimerization may similarly disrupt enzyme binding .
Comparative Metabolism :
- Testosterone is metabolized to DHT (via 5α-reductase) and androsterone. The 17α-OH configuration in this compound may redirect metabolism toward atypical pathways, such as increased 5β-reduction, as observed in 17-epi-ethynylestradiol derivatives .
Biological Activity
17-epi-Testosterone-d3 is a synthetic derivative of testosterone, characterized by its unique structural modifications that influence its biological activity. This compound, often used in research settings, has garnered interest due to its potential roles in androgen receptor modulation and its implications in various physiological processes.
Chemical Structure and Properties
This compound (C19H28O2) is a deuterated form of epitestosterone, which is the 17-alpha epimer of testosterone. The presence of deuterium (d3) alters its pharmacokinetic properties, making it a valuable tool for metabolic studies.
Androgenic Activity
Research indicates that this compound exhibits antiandrogenic properties . In vitro studies have demonstrated that it can bind to androgen receptors, albeit with a lower affinity compared to testosterone. This binding can inhibit the action of testosterone on androgen-dependent tissues. For instance, one study found that epitestosterone can counteract testosterone's effects on prostate tissue in castrated male mice, suggesting a significant role in modulating androgenic activity .
Antiandrogenic Mechanisms
The antiandrogenic effects of this compound may involve several mechanisms:
- Receptor Binding : It competes with testosterone for binding sites on androgen receptors.
- 5-alpha-reductase Inhibition : It demonstrates strong inhibitory activity against 5-alpha-reductase, an enzyme responsible for converting testosterone to dihydrotestosterone (DHT), a more potent androgen .
- Antigonadotropic Effects : There is evidence suggesting that it may exert weak antigonadotropic effects, impacting gonadotropin levels and subsequently influencing testosterone synthesis .
Pharmacokinetics
The pharmacokinetic profile of this compound has been studied through various methods, including gas chromatography-mass spectrometry (GC-MS). These studies reveal that the compound has favorable properties for analytical detection in biological fluids, which is crucial for understanding its metabolism and excretion .
Clinical Implications
Studies involving the administration of this compound have shown promising results in understanding conditions influenced by androgen levels:
- Sarcopenia : Research suggests potential benefits in muscle mass preservation among older adults, where testosterone levels typically decline. The modulation of androgen activity through compounds like this compound may offer therapeutic avenues for combating muscle wasting .
- Prostate Health : The compound's ability to inhibit DHT production positions it as a candidate for further exploration in prostate health management, particularly in conditions such as benign prostatic hyperplasia and prostate cancer .
Data Table
Parameter | Value |
---|---|
Molecular Formula | C19H28O2 |
Molecular Weight | 288.43 g/mol |
Androgen Receptor Affinity | Lower than Testosterone |
5-alpha-reductase Inhibition | Strong |
Antigonadotropic Activity | Weak |
Q & A
Basic Research Questions
Q. What are the critical considerations for synthesizing and characterizing 17-epi-Testosterone-d3 in laboratory settings?
Synthesis of this compound requires precise isotopic labeling (deuterium at positions 16,16,17) to ensure structural fidelity. Characterization involves nuclear magnetic resonance (NMR) to confirm deuterium placement and high-resolution mass spectrometry (HRMS) for molecular weight validation (288.42442 g/mol). Purity assessment via HPLC with UV detection at 240 nm is recommended, with storage at 2–8°C to prevent degradation .
Q. How should researchers design initial stability studies for this compound under varying storage conditions?
Stability studies should follow ICH Q1A guidelines, testing thermal, photolytic, and hydrolytic degradation. Use accelerated stability protocols (e.g., 40°C/75% RH for 6 months) with periodic sampling. Analytical methods like LC-MS/MS should quantify degradation products, ensuring deuterium retention is monitored to avoid isotopic exchange artifacts .
Q. What analytical techniques are most reliable for distinguishing this compound from its non-deuterated counterpart in biological matrices?
Liquid chromatography-tandem mass spectrometry (LC-MS/MS) with multiple reaction monitoring (MRM) is optimal. The deuterium labeling shifts the mass-to-charge ratio (m/z), enabling differentiation. Validation should include specificity tests against endogenous steroids and matrix effects assessment using post-column infusion .
Advanced Research Questions
Q. How can researchers resolve discrepancies in pharmacokinetic data for this compound across studies with varying methodologies?
Discrepancies often arise from differences in sample preparation (e.g., solid-phase extraction vs. liquid-liquid extraction) or ionization efficiency in MS. To reconcile
- Conduct cross-validation using harmonized protocols.
- Apply isotopic correction algorithms to account for deuterium loss during analysis.
- Perform meta-analysis with stratification by study design and analytical platforms .
Q. What strategies optimize the detection of this compound in low-abundance biological samples (e.g., urine or serum)?
Pre-concentration via immunoaffinity chromatography or derivatization (e.g., using pentafluorobenzyl bromide) enhances sensitivity. Employing a deuterated internal standard (e.g., 17-epi-Testosterone-d5) minimizes matrix interference. Method validation must include limits of detection (LOD < 0.1 ng/mL) and precision (CV < 15%) .
Q. How do isotopic effects of deuterium in this compound influence its metabolic stability compared to non-deuterated analogs?
Deuterium kinetic isotope effects (KIEs) can slow metabolic oxidation at labeled positions, altering half-life and metabolite profiles. In vitro assays using human liver microsomes should compare intrinsic clearance rates. Isotope ratio monitoring via GC-C-IRMS can track deuterium retention in metabolites .
Q. What ethical and regulatory frameworks govern the use of this compound in doping-related research?
Compliance with WADA regulations (e.g., inclusion in the Prohibited List) and Schedule III controls (U.S. Controlled Substances Act) is mandatory. Ethical approvals must address human participant risks, including anonymization of biological samples and justification of deuterium exposure levels in vivo .
Methodological and Data Analysis Questions
Q. How should researchers document experimental protocols for this compound to ensure reproducibility?
Follow the Beilstein Journal of Organic Chemistry guidelines:
- Detail synthetic steps, including solvent purity and reaction temperatures.
- Provide raw NMR/MS spectra in supplementary materials.
- Use SI units and predefined statistical thresholds (e.g., p < 0.05) .
Q. What statistical approaches are appropriate for analyzing dose-response relationships in this compound studies?
Non-linear regression models (e.g., four-parameter logistic curves) are suitable for dose-response data. For small sample sizes, apply Bayesian hierarchical models to reduce Type I errors. Report confidence intervals and effect sizes instead of relying solely on p-values .
Q. How can researchers address limitations in detecting this compound due to cross-reactivity in immunoassays?
Perform cross-reactivity screens against structurally similar steroids (e.g., testosterone, epitestosterone). Use orthogonal methods (e.g., LC-MS/MS) to confirm results. Report false-positive rates and include a "confirmation analysis" section in the methodology .
Q. Contradiction and Gap Analysis
Q. Why do some studies report inconsistent pharmacokinetic half-lives for this compound?
Variations arise from differences in administration routes (oral vs. intramuscular) and interspecies metabolic rates. To mitigate, standardize administration protocols and use physiologically based pharmacokinetic (PBPK) modeling to extrapolate data across species .
Q. What gaps exist in current methodologies for quantifying this compound in non-invasive samples (e.g., hair or saliva)?
Limited sensitivity in low-abundance matrices and lack of validated reference ranges are key gaps. Future studies should explore microsampling techniques (e.g., volumetric absorptive microsampling) and harmonize cut-off values with forensic standards .
Properties
IUPAC Name |
(8R,9S,10R,13S,14S,17R)-16,16,17-trideuterio-17-hydroxy-10,13-dimethyl-1,2,6,7,8,9,11,12,14,15-decahydrocyclopenta[a]phenanthren-3-one | |
---|---|---|
Source | PubChem | |
URL | https://pubchem.ncbi.nlm.nih.gov | |
Description | Data deposited in or computed by PubChem | |
InChI |
InChI=1S/C19H28O2/c1-18-9-7-13(20)11-12(18)3-4-14-15-5-6-17(21)19(15,2)10-8-16(14)18/h11,14-17,21H,3-10H2,1-2H3/t14-,15-,16-,17+,18-,19-/m0/s1/i6D2,17D | |
Source | PubChem | |
URL | https://pubchem.ncbi.nlm.nih.gov | |
Description | Data deposited in or computed by PubChem | |
InChI Key |
MUMGGOZAMZWBJJ-ZWGJEZJDSA-N | |
Source | PubChem | |
URL | https://pubchem.ncbi.nlm.nih.gov | |
Description | Data deposited in or computed by PubChem | |
Canonical SMILES |
CC12CCC3C(C1CCC2O)CCC4=CC(=O)CCC34C | |
Source | PubChem | |
URL | https://pubchem.ncbi.nlm.nih.gov | |
Description | Data deposited in or computed by PubChem | |
Isomeric SMILES |
[2H][C@@]1([C@]2(CC[C@H]3[C@H]([C@@H]2CC1([2H])[2H])CCC4=CC(=O)CC[C@]34C)C)O | |
Source | PubChem | |
URL | https://pubchem.ncbi.nlm.nih.gov | |
Description | Data deposited in or computed by PubChem | |
Molecular Formula |
C19H28O2 | |
Source | PubChem | |
URL | https://pubchem.ncbi.nlm.nih.gov | |
Description | Data deposited in or computed by PubChem | |
Molecular Weight |
291.4 g/mol | |
Source | PubChem | |
URL | https://pubchem.ncbi.nlm.nih.gov | |
Description | Data deposited in or computed by PubChem | |
Retrosynthesis Analysis
AI-Powered Synthesis Planning: Our tool employs the Template_relevance Pistachio, Template_relevance Bkms_metabolic, Template_relevance Pistachio_ringbreaker, Template_relevance Reaxys, Template_relevance Reaxys_biocatalysis model, leveraging a vast database of chemical reactions to predict feasible synthetic routes.
One-Step Synthesis Focus: Specifically designed for one-step synthesis, it provides concise and direct routes for your target compounds, streamlining the synthesis process.
Accurate Predictions: Utilizing the extensive PISTACHIO, BKMS_METABOLIC, PISTACHIO_RINGBREAKER, REAXYS, REAXYS_BIOCATALYSIS database, our tool offers high-accuracy predictions, reflecting the latest in chemical research and data.
Strategy Settings
Precursor scoring | Relevance Heuristic |
---|---|
Min. plausibility | 0.01 |
Model | Template_relevance |
Template Set | Pistachio/Bkms_metabolic/Pistachio_ringbreaker/Reaxys/Reaxys_biocatalysis |
Top-N result to add to graph | 6 |
Feasible Synthetic Routes
Disclaimer and Information on In-Vitro Research Products
Please be aware that all articles and product information presented on BenchChem are intended solely for informational purposes. The products available for purchase on BenchChem are specifically designed for in-vitro studies, which are conducted outside of living organisms. In-vitro studies, derived from the Latin term "in glass," involve experiments performed in controlled laboratory settings using cells or tissues. It is important to note that these products are not categorized as medicines or drugs, and they have not received approval from the FDA for the prevention, treatment, or cure of any medical condition, ailment, or disease. We must emphasize that any form of bodily introduction of these products into humans or animals is strictly prohibited by law. It is essential to adhere to these guidelines to ensure compliance with legal and ethical standards in research and experimentation.