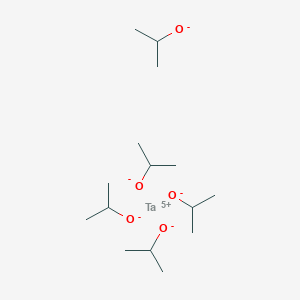
Tantalum (V) isopropoxide
Overview
Description
Tantalum(V) isopropoxide (CAS: 16761-83-4), also known as pentaisopropoxytantalum, is a metal-organic compound with the formula Ta(OCH(CH₃)₂)₅. This alkoxide derivative of tantalum is a liquid at room temperature and reacts vigorously with water, undergoing hydrolysis to form tantalum oxides and isopropyl alcohol . It is commonly used as a precursor in sol-gel synthesis, thin-film deposition, and catalysis due to its high reactivity and ability to form homogeneous metal oxide networks .
Preparation Methods
Direct Alkoxylation of Tantalum(V) Chloride
The most widely documented method for synthesizing tantalum (V) isopropoxide involves the reaction of tantalum(V) chloride (TaCl₅) with isopropanol under anhydrous conditions. This approach leverages the high reactivity of TaCl₅ with alcohols to form the corresponding alkoxide.
Reaction Mechanism
The reaction proceeds via a nucleophilic substitution mechanism, where isopropanol displaces chloride ligands on the tantalum center. The stoichiometric equation is:
\text{TaCl}5 + 5 \text{CH}3\text{CH(OH)CH}3 \rightarrow \text{Ta(OCH(CH}3\text{)}2\text{)}5 + 5 \text{HCl} $$
.
Anhydrous conditions are critical to prevent hydrolysis, which yields tantalum oxychloride (TaOCl₃) or tantalum pentoxide (Ta₂O₅) as byproducts. The reaction is typically conducted in an inert atmosphere (e.g., nitrogen or argon) at temperatures between 60–80°C to optimize kinetics while minimizing thermal decomposition.
Industrial Production Methods
Industrial-scale synthesis employs continuous-flow reactors to enhance efficiency and safety. Key parameters include:
Parameter | Laboratory Scale | Industrial Scale |
---|---|---|
Temperature | 60–80°C | 70–90°C |
Reaction Time | 12–24 hours | 2–4 hours |
Yield | 70–85% | 85–92% |
Purity | ≥95% | ≥98% |
Industrial processes often incorporate in-line HCl scrubbing systems to mitigate corrosion risks and improve product purity.
Solvent-Mediated Synthesis
Polar aprotic solvents such as 2-methoxyethanol or tetrahydrofuran (THF) are employed to stabilize intermediates and reduce side reactions. This method is favored for producing high-purity this compound for optoelectronic applications.
Procedure
- Solvent Preparation : 2-Methoxyethanol is dried over molecular sieves and degassed via nitrogen sparging.
- Reagent Addition : TaCl₅ is dissolved in the solvent, followed by dropwise addition of isopropanol.
- Stirring and Reflux : The mixture is stirred at 50°C for 6 hours, then refluxed at 120°C for 2 hours to ensure complete ligand exchange.
Advantages
- Reduced Hydrolysis : Solvent coordination to tantalum lowers hydrolysis susceptibility.
- Enhanced Solubility : Facilitates homogeneous mixing, critical for thin-film precursor solutions.
Purification Techniques
Vacuum Distillation
Crude product is purified via fractional distillation under reduced pressure (0.1–1 mmHg). This compound distills at 110–115°C, separating it from residual isopropanol and oligomeric byproducts.
Recrystallization
For ultrahigh-purity applications, the distillate is recrystallized from hexane at -20°C. This step removes trace chlorides and solvent residues, achieving purities >99.9%.
Comparative Analysis of Synthesis Methods
Method | Purity (%) | Yield (%) | Scalability | Cost Efficiency |
---|---|---|---|---|
Direct Alkoxylation | 95–98 | 70–92 | High | Moderate |
Solvent-Mediated | 98–99.9 | 65–80 | Moderate | Low |
Direct alkoxylation is preferred for bulk production due to its scalability, while solvent-mediated routes are reserved for high-purity niche applications.
Emerging Innovations
Microwave-Assisted Synthesis
Recent studies demonstrate that microwave irradiation reduces reaction times by 50% while maintaining yields >80%. This method enhances energy efficiency and is under exploration for industrial adoption.
Green Chemistry Approaches
Researchers are substituting isopropanol with bio-derived alcohols (e.g., 2-butanol) to reduce the carbon footprint. Preliminary results show comparable yields but require optimization for purity.
Chemical Reactions Analysis
Types of Reactions
Tantalum (V) isopropoxide undergoes various types of chemical reactions, including:
Hydrolysis: Reacts with water to form tantalum pentoxide and isopropanol.
Alcoholysis: Reacts with other alcohols to exchange isopropoxy groups with other alkoxy groups.
Thermal Decomposition: Decomposes upon heating to form tantalum pentoxide and isopropanol.
Common Reagents and Conditions
Hydrolysis: Water or aqueous solutions.
Alcoholysis: Different alcohols such as methanol or ethanol.
Thermal Decomposition: Elevated temperatures in an inert atmosphere.
Major Products
Hydrolysis: Tantalum pentoxide and isopropanol.
Alcoholysis: Mixed alkoxides and isopropanol.
Thermal Decomposition: Tantalum pentoxide and isopropanol.
Scientific Research Applications
Thin Film Deposition
Overview
Tantalum (V) isopropoxide is primarily used in the production of tantalum oxide (Ta₂O₅) thin films through various deposition techniques, including:
- Chemical Vapor Deposition (CVD)
- Atomic Layer Deposition (ALD)
- Sol-Gel Processing
Applications
These thin films exhibit properties suitable for:
- Dielectric materials in microelectronics
- Optical coatings with high refractive indices
- Electrochromic devices , which change color upon electrical stimulus
Table 1: Properties of Tantalum Oxide Films Produced from this compound
Property | Value |
---|---|
Refractive Index | Up to 2.039 |
Dielectric Constant | High (varies by method) |
Thickness Control | Nanometer scale |
Optical Transparency | High |
Biomedical Applications
Nanoparticle Development
Tantalum-based nanoparticles synthesized from this compound have shown promise in the field of cancer treatment. These nanoparticles leverage unique properties such as:
- Biocompatibility
- Efficient photothermal conversion
Case Study: Cancer Management
Recent research highlights the use of tantalum nanoparticles in photothermal therapy, where they are used to target and destroy cancer cells through localized heating. Modifications to enhance their biocompatibility and targeting capabilities are ongoing.
Electronics and Optoelectronics
Dielectric Applications
Tantalum oxide films are crucial for:
- Dynamic Random Access Memory (DRAM)
- Field-Effect Transistors (FETs)
These applications benefit from the high dielectric constant of Ta₂O₅, which enhances device performance.
Optical Materials
This compound-derived films are employed in optical devices due to their:
- High refractive index
- Low absorption loss
These properties make them suitable for applications in lenses, mirrors, and other optical components.
Synthesis of Mixed-Metal Oxides
This compound can react with other metal alkoxides to produce mixed-metal oxides, such as lithium tantalate (LiTaO₃), which possess nonlinear optical properties beneficial for telecommunications and laser technologies.
Table 2: Mixed-Metal Oxides Derived from this compound
Mixed Metal Oxide | Application |
---|---|
LiTaO₃ | Nonlinear optics |
SrTa₂O₆ | Photonic devices |
Mechanism of Action
The mechanism of action of pentaisopropoxytantalum(V) in catalytic processes involves the coordination of the tantalum center with reactant molecules, facilitating their transformation. The isopropoxy groups can be replaced by other ligands, allowing the tantalum center to participate in various catalytic cycles. The molecular targets and pathways depend on the specific reaction and conditions used.
Comparison with Similar Compounds
Key Properties :
- Molecular Weight : ~475.95 g/mol (calculated from stoichiometry).
- Reactivity : Hydrolyzes in water, requiring anhydrous handling .
- Safety : Classified as a flammable liquid (Hazard Class 3, UN3274) with handling precautions for flammability and reactivity .
The following section compares Tantalum(V) isopropoxide with structurally or functionally related compounds, including tantalum derivatives and group V transition metal alkoxides.
Tantalum(V) Ethoxide (CAS: 6074-84-6)
Molecular Formula: Ta(OCH₂CH₃)₅ Molecular Weight: 406.25 g/mol . Physical State: Liquid with a boiling point range starting at 21°C and a flash point of 29°C (closed cup) . Reactivity: Similar to Tantalum(V) isopropoxide, it hydrolyzes in water but offers slower reaction kinetics due to the ethoxide ligand’s lower steric hindrance compared to isopropoxide. Applications: Used in the synthesis of tantalum-based thin films and nanomaterials . Safety: Classified as a Category 3 flammable liquid with skin corrosion (Category 1B) and severe eye damage (Category 1) hazards .
Niobium(V) Ethoxide (CAS: Nb(OCH₂CH₃)₅)
Molecular Formula: Nb(OCH₂CH₃)₅ Molecular Weight: ~317.91 g/mol (calculated). Physical State: Liquid (analogous to tantalum ethoxide). Reactivity: Less hydrolytically stable than tantalum derivatives due to niobium’s lower electronegativity, leading to faster hydrolysis rates. Applications: Employed in catalytic systems and dielectric material synthesis .
Zirconium(IV) Isopropoxide (CAS: Zr(OCH(CH₃)₂)₄·(CH₃)₂CHOH)
Molecular Formula: Zr(OCH(CH₃)₂)₄·(CH₃)₂CHOH Molecular Weight: ~387.32 g/mol (calculated). Physical State: Liquid, often stabilized as an isopropanol adduct . Reactivity: Hydrolyzes to form zirconium oxides but exhibits lower Lewis acidity compared to tantalum analogs, reducing catalytic activity in certain reactions. Applications: Widely used in sol-gel processes for zirconia coatings and ceramics .
Tantalum(V) Oxide (CAS: 1314-61-0)
Molecular Formula: Ta₂O₅ Molecular Weight: 441.89 g/mol . Physical State: White solid with a melting point of 1,800°C and a density of 8.2 g/cm³ . Reactivity: Insoluble in water and most acids, with high thermal and chemical stability . Applications: Critical in electronics for high-k dielectric layers in capacitors and optical coatings . Safety: Non-flammable but requires dust control to prevent inhalation hazards .
Comparative Data Tables
Table 1: Physicochemical Properties
Key Research Findings
- Reactivity Trends : Tantalum alkoxides exhibit faster hydrolysis rates compared to zirconium analogs due to higher Lewis acidity, making them more suitable for rapid oxide formation .
- Thermal Stability : Tantalum(V) oxide’s high decomposition temperature (1,470°C) contrasts with alkoxides’ lower thermal stability, limiting alkoxides to low-temperature processing .
- Safety Handling : Alkoxides require stringent moisture control and flammability precautions, whereas oxides demand respiratory protection against dust .
Biological Activity
Tantalum (V) isopropoxide (Ta(OiPr)₅) is an organometallic compound that has garnered interest due to its unique properties and potential applications in various fields, particularly in biomedical and materials science. This article explores the biological activity of this compound, focusing on its biocompatibility, toxicity, and applications in drug delivery and imaging.
Chemical Structure and Properties
This compound consists of a tantalum atom coordinated to five isopropoxide groups, resulting in a pale yellow to colorless liquid. Its high oxidation state (+5) contributes to its stability and reactivity, making it suitable for various applications, including thin film deposition and catalysis.
Biocompatibility and Toxicity
Research indicates that this compound exhibits low toxicity and good biocompatibility , which are critical factors for its use in biomedical applications. The biological performance of tantalum compounds generally shows inertness in vivo and in vitro, with low solubility and toxicity levels. This makes this compound a promising candidate for medical imaging and drug delivery systems .
Case Studies on Biocompatibility
- Medical Imaging : Tantalum oxide nanoparticles derived from this compound have been effectively utilized in medical imaging due to their radiopacity, enhancing contrast in X-ray imaging techniques. Studies have demonstrated their potential for improving diagnostic capabilities.
- Drug Delivery Systems : The incorporation of functional groups into the tantalum oxide framework has shown promise in enhancing interactions with biological systems, thereby improving the performance of drug delivery vehicles.
Applications in Catalysis
This compound serves as a catalyst or catalyst precursor for various chemical reactions, including olefin metathesis and dehydrogenation of alkanes. These reactions are significant for polymer synthesis and clean fuel production, respectively. Ongoing research is exploring its effectiveness in other catalytic processes such as hydrogenation and selective oxidation of organic molecules .
Comparative Analysis with Other Metal Alkoxides
The following table summarizes the properties and applications of this compound compared to other metal alkoxides:
Compound Name | Formula | Unique Features |
---|---|---|
This compound | Ta(OiPr)₅ | Low toxicity, good biocompatibility |
Tantalum (V) Ethoxide | Ta(OEt)₅ | Primarily used for chemical vapor deposition |
Niobium (V) Ethoxide | Nb(OEt)₅ | Similar structure but different metal properties |
Zirconium(IV) Propoxide | Zr(OiPr)₄ | Commonly used in sol-gel processes for ceramics |
Titanium(IV) Isopropoxide | Ti(OiPr)₄ | Widely utilized in photocatalysis |
Research Findings
Recent studies have focused on the synthesis methods of this compound and its derivatives. Techniques such as sol-gel processing have been employed to create tantalum oxide nanoparticles with tailored properties for specific applications. For instance, varying the concentration of organic templates during synthesis influences the optical properties and porosity of the resulting films, enhancing their applicability in sensors and other advanced materials .
Q & A
Q. Basic: What are the critical considerations for synthesizing Tantalum (V) isopropoxide in an academic laboratory?
Answer:
Synthesis of this compound requires strict control of moisture and oxygen due to its high sensitivity to hydrolysis and oxidation. The reaction typically involves TaCl₅ and isopropanol in an inert atmosphere (e.g., nitrogen or argon), followed by distillation to isolate the product . Key steps include:
- Use of anhydrous solvents and reagents.
- Monitoring reaction progress via gas chromatography or FTIR to confirm ligand exchange.
- Purification via vacuum distillation to remove excess alcohol and byproducts.
Safety protocols, such as wearing gloves and eye protection, are essential due to the compound’s reactivity .
Q. Basic: How should researchers characterize the purity of this compound?
Answer:
Characterization involves a combination of analytical techniques:
- Elemental analysis : Verify Ta and C/H/O ratios via ICP-OES and combustion analysis.
- Spectroscopy :
- Thermogravimetric analysis (TGA) to assess thermal stability and decomposition behavior .
Q. Advanced: How can discrepancies in reported melting points (e.g., 92°C vs. literature values) be resolved during experimental validation?
Answer:
Discrepancies may arise from impurities, polymorphic forms, or measurement conditions. To address this:
Repeat measurements using calibrated DSC/TGA under inert conditions.
Compare with structurally analogous alkoxides (e.g., Ta(V) ethoxide, mp 80°C) to contextualize thermal behavior .
Analyze crystallinity via XRD; amorphous phases may exhibit broad melting ranges.
Document procedural details (heating rate, sample encapsulation) to ensure reproducibility .
Q. Advanced: What methodological challenges arise when using this compound as a precursor for thin-film deposition?
Answer:
Key challenges include:
- Hydrolysis control : Even trace moisture causes premature hydrolysis, leading to particulate formation. Solutions include using gloveboxes and anhydrous solvents .
- Volatility : The compound’s low volatility (bp 122°C at 0.1 mmHg) requires optimized CVD/ALD parameters (e.g., carrier gas flow rates, substrate temperature) .
- Film stoichiometry : Off-stoichiometry due to ligand decomposition during deposition. Mitigation involves in-situ mass spectrometry to monitor gas-phase species .
Q. Basic: What safety protocols are essential when handling this compound?
Answer:
- Personal protective equipment (PPE) : Gloves, lab coat, and goggles to prevent skin/eye contact .
- Ventilation : Use fume hoods to avoid inhalation of vapors.
- Spill management : Neutralize spills with dry sand; avoid water to prevent exothermic reactions .
- Storage : Keep in sealed, moisture-free containers under inert gas .
Q. Advanced: How can researchers address contradictions in spectroscopic data (e.g., NMR shifts) across studies?
Answer:
Contradictions often stem from solvent effects, concentration, or impurities. Methodological steps:
Reproduce conditions : Match solvent (e.g., deuterated benzene vs. chloroform) and temperature.
Spike testing : Add known impurities (e.g., hydrolysis products) to identify interference.
Cross-validate with alternative techniques : Use X-ray crystallography or Raman spectroscopy to confirm structural assignments .
Q. Basic: What are the primary applications of this compound in materials science?
Answer:
- Thin-film deposition : Precursor for Ta₂O₅ films in dielectric applications.
- Catalysis : Lewis acid catalyst in organic synthesis (e.g., esterification).
- Nanoparticle synthesis : Template for Ta-based nanostructures via sol-gel methods .
Q. Advanced: What strategies optimize the sol-gel synthesis of Ta₂O₅ using this compound?
Answer:
- Controlled hydrolysis : Add water slowly via syringe pump to avoid rapid condensation.
- Chelating agents : Use acetylacetone to moderate reactivity and improve gel stability.
- Aging conditions : Adjust pH and temperature to tailor pore size and crystallinity .
Q. Basic: How should experimental procedures involving this compound be documented for reproducibility?
Answer:
- Detailed synthesis logs : Include reaction times, temperatures, and purification steps.
- Instrument calibration data : Specify NMR spectrometer frequencies and DSC heating rates.
- Supporting information : Provide raw spectral data and TGA curves in supplementary files .
Q. Advanced: How does this compound’s reactivity compare to other Group V alkoxides (e.g., Niobium isopropoxide)?
Answer:
- Electrophilicity : Ta(V) is less electrophilic than Nb(V) due to higher atomic radius, leading to slower hydrolysis rates.
- Thermal stability : Ta(V) alkoxides decompose at higher temperatures (e.g., Ta vs. Nb ethoxide: ΔT ~50°C).
- Coordination chemistry : Ta(V) prefers six-coordinate geometries, influencing precursor design for mixed-metal oxides .
Properties
IUPAC Name |
propan-2-olate;tantalum(5+) | |
---|---|---|
Source | PubChem | |
URL | https://pubchem.ncbi.nlm.nih.gov | |
Description | Data deposited in or computed by PubChem | |
InChI |
InChI=1S/5C3H7O.Ta/c5*1-3(2)4;/h5*3H,1-2H3;/q5*-1;+5 | |
Source | PubChem | |
URL | https://pubchem.ncbi.nlm.nih.gov | |
Description | Data deposited in or computed by PubChem | |
InChI Key |
BZXLPZMNHQWSNR-UHFFFAOYSA-N | |
Source | PubChem | |
URL | https://pubchem.ncbi.nlm.nih.gov | |
Description | Data deposited in or computed by PubChem | |
Canonical SMILES |
CC(C)[O-].CC(C)[O-].CC(C)[O-].CC(C)[O-].CC(C)[O-].[Ta+5] | |
Source | PubChem | |
URL | https://pubchem.ncbi.nlm.nih.gov | |
Description | Data deposited in or computed by PubChem | |
Molecular Formula |
C15H35O5Ta | |
Source | PubChem | |
URL | https://pubchem.ncbi.nlm.nih.gov | |
Description | Data deposited in or computed by PubChem | |
DSSTOX Substance ID |
DTXSID90501834 | |
Record name | Tantalum(5+) pentapropan-2-olate | |
Source | EPA DSSTox | |
URL | https://comptox.epa.gov/dashboard/DTXSID90501834 | |
Description | DSSTox provides a high quality public chemistry resource for supporting improved predictive toxicology. | |
Molecular Weight |
476.38 g/mol | |
Source | PubChem | |
URL | https://pubchem.ncbi.nlm.nih.gov | |
Description | Data deposited in or computed by PubChem | |
CAS No. |
16761-83-4 | |
Record name | Tantalum(5+) pentapropan-2-olate | |
Source | EPA DSSTox | |
URL | https://comptox.epa.gov/dashboard/DTXSID90501834 | |
Description | DSSTox provides a high quality public chemistry resource for supporting improved predictive toxicology. | |
Disclaimer and Information on In-Vitro Research Products
Please be aware that all articles and product information presented on BenchChem are intended solely for informational purposes. The products available for purchase on BenchChem are specifically designed for in-vitro studies, which are conducted outside of living organisms. In-vitro studies, derived from the Latin term "in glass," involve experiments performed in controlled laboratory settings using cells or tissues. It is important to note that these products are not categorized as medicines or drugs, and they have not received approval from the FDA for the prevention, treatment, or cure of any medical condition, ailment, or disease. We must emphasize that any form of bodily introduction of these products into humans or animals is strictly prohibited by law. It is essential to adhere to these guidelines to ensure compliance with legal and ethical standards in research and experimentation.