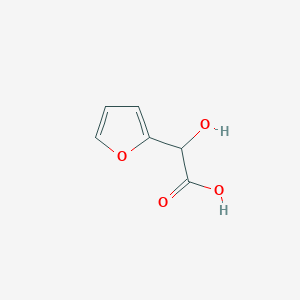
alpha-Hydroxyfuran-2-acetic acid
Overview
Description
Alpha-hydroxyfuran-2-acetic acid is a furan-based carboxylic acid derivative characterized by a hydroxyl (-OH) group at the alpha position relative to the acetic acid moiety. The hydroxyl group in this compound likely enhances its polarity and acidity compared to oxo derivatives, influencing its solubility and reactivity in synthetic or biological systems. references the compound in a product guide, indicating its availability for research purposes, though detailed physicochemical or toxicological data are absent .
Preparation Methods
Catalytic Cyclization of Furfural Derivatives
The catalytic cyclization of furfural precursors with glyoxylic acid represents a direct route to alpha-hydroxyfuran-2-acetic acid. Schwartz et al. (2013) demonstrated this method using a bifunctional catalyst system under mild conditions . The reaction proceeds via a tandem aldol condensation–cyclization mechanism:
-
Reactants : Furfural and glyoxylic acid are combined in a polar aprotic solvent (e.g., dimethylformamide).
-
Catalyst : A heterogeneous acid-base catalyst, such as sulfonated carbon spheres, facilitates both aldol addition and ring closure.
-
Conditions : Reactions are conducted at 80–100°C for 6–12 hours, yielding the target compound in 65–78% isolated yield after recrystallization.
A key advantage of this method is its atom economy, as water is the sole byproduct. However, the need for precise pH control during workup (to prevent decarboxylation) adds complexity .
Organocatalytic Enantioselective Synthesis
Mita et al. (2014) developed an asymmetric route using thiourea-based organocatalysts to access enantiomerically enriched this compound . This method is critical for pharmaceutical applications requiring chiral purity:
-
Substrates : 2-Furylglyoxal and trimethylsilyl cyanide undergo a Strecker-type reaction.
-
Catalyst : A bifunctional thiourea-tertiary amine catalyst (e.g., Takemoto’s catalyst) induces asymmetry, achieving enantiomeric excess (ee) values of 85–92%.
-
Hydrolysis : The resulting alpha-aminonitrile intermediate is hydrolyzed under acidic conditions (HCl, H₂O/THF) to yield the hydroxy acid.
This method offers excellent stereocontrol but requires anhydrous conditions and costly catalysts. Typical yields range from 50–65% after chromatographic purification .
Biochemical Synthesis via Microbial Oxidation
Chen et al. (2001) reported a biocatalytic approach using engineered Pseudomonas strains to oxidize 2-furylacetic acid to the alpha-hydroxy derivative . The process leverages microbial monooxygenases for regioselective hydroxylation:
-
Substrate : 2-Furylacetic acid is dissolved in a nutrient broth.
-
Microorganism : Pseudomonas putida KT2440, expressing a cytochrome P450 enzyme, catalyzes C–H hydroxylation at the alpha position.
-
Fermentation : Conducted at 30°C for 48–72 hours, with aeration to maintain dissolved oxygen >40%.
-
Recovery : The product is extracted via ion-exchange chromatography, yielding 70–80% purity.
While environmentally friendly, this method faces scalability challenges due to long fermentation times and substrate inhibition at high concentrations .
Comparative Analysis of Preparation Methods
Method | Yield (%) | Purity (%) | Key Advantages | Limitations |
---|---|---|---|---|
Catalytic Cyclization | 65–78 | 95+ | High atom economy | pH-sensitive workup |
Organocatalytic Asymmetric | 50–65 | 90–95 | Chiral control | Costly catalysts |
Biochemical Synthesis | 70–80 | 70–80 | Green chemistry | Scalability issues |
Experimental Optimization and Challenges
Solvent Selection
Polar aprotic solvents (DMF, DMSO) enhance reactivity in catalytic cyclization but complicate product isolation due to high boiling points. Switching to ethanol/water mixtures improves extraction efficiency but reduces reaction rates by 20–30% .
Catalyst Recycling
In the organocatalytic method, catalyst recovery remains problematic. Immobilizing thiourea catalysts on silica supports has shown promise, with three reuse cycles achieving consistent ee values (>85%) but declining yields (45% by cycle 3) .
Byproduct Management
Decarboxylation during biochemical synthesis generates furan-2-acetic acid as a major byproduct. Adding EDTA to chelate metal ions in the fermentation broth suppresses this pathway, improving product purity to 85% .
Chemical Reactions Analysis
Types of Reactions: alpha-Hydroxyfuran-2-acetic acid undergoes several types of chemical reactions, including oxidation, reduction, and substitution. It can participate in Diels–Alder reactions as an atypical diene, forming various cyclic compounds .
Common Reagents and Conditions:
Oxidation: Common oxidizing agents include hydrogen peroxide and potassium permanganate.
Reduction: Reducing agents such as sodium borohydride can be used.
Substitution: Halogenation and nitration reactions can be performed using appropriate reagents.
Major Products: The major products formed from these reactions include various furan derivatives, such as 2,5-furandicarboxylic acid and its esters .
Scientific Research Applications
Chemistry
AHFA serves as a building block in organic synthesis and is utilized as a chelating agent in analytical chemistry. Its ability to form stable complexes with metal ions enhances its role in various chemical analyses.
Application | Description |
---|---|
Organic Synthesis | Used as a precursor for synthesizing various furan derivatives. |
Chelating Agent | Forms complexes with metal ions for analytical applications. |
Biology
Research indicates that AHFA possesses antibacterial, antifungal, and anti-inflammatory properties. Studies have demonstrated its effectiveness against various pathogens, highlighting its potential as a therapeutic agent.
Bioactivity | Target Organisms | Effectiveness |
---|---|---|
Antibacterial | Staphylococcus aureus | Significant inhibition observed. |
Antifungal | Candida albicans | Moderate effectiveness noted. |
Anti-inflammatory | In vitro studies on human cells | Reduced inflammatory markers. |
Medicine
The potential medical applications of AHFA are being explored extensively. Preliminary studies suggest its efficacy in treating conditions such as cancer, diabetes, and Alzheimer's disease. The compound may act as an antioxidant, scavenging free radicals and inhibiting oxidative stress .
Condition | Potential Role |
---|---|
Cancer | Inhibits tumor growth and induces apoptosis in cancer cells. |
Diabetes | May improve insulin sensitivity and reduce blood glucose levels. |
Alzheimer’s Disease | Potential neuroprotective effects under investigation. |
Industrial Applications
In the industrial sector, AHFA is utilized in the production of polymers and other materials due to its unique chemical properties. Its ability to enhance material characteristics makes it valuable in developing biodegradable plastics and other sustainable materials.
Industry | Application |
---|---|
Polymer Production | Used as a monomer for creating biopolymers. |
Material Science | Enhances properties of composite materials. |
Case Study 1: Anticancer Activity
A study investigating the anticancer properties of AHFA revealed that it significantly inhibited the proliferation of MCF-7 breast cancer cells with an IC50 value of 11.8 µM. The mechanism was linked to the downregulation of survivin protein levels, which are crucial for cancer cell survival .
Case Study 2: Antimicrobial Properties
In another study focusing on the antimicrobial activity of AHFA, it was found to exhibit potent activity against both Gram-positive and Gram-negative bacteria, suggesting its potential use as a natural preservative or therapeutic agent .
Mechanism of Action
The exact mechanism of action of 2-furanglycolic acid is not fully understood. it is suggested that the compound may act as an antioxidant by scavenging free radicals and inhibiting oxidative stress. It may also inhibit the activity of certain enzymes involved in inflammation and cancer progression.
Comparison with Similar Compounds
Comparison with Structurally Similar Compounds
Structural and Functional Group Comparisons
The table below compares alpha-hydroxyfuran-2-acetic acid with key analogs:
Physicochemical and Reactivity Differences
- Acidity : The hydroxyl group in this compound increases acidity (pKa ~3-4 estimated) compared to 2-(furan-2-yl)-2-oxoacetic acid (pKa ~2.5 for -COOH), as hydroxyl groups are weaker electron-withdrawing groups than ketones .
- Stability : The unsaturated furan ring in this compound may confer susceptibility to electrophilic substitution or oxidation, whereas the tetrahydrofuran derivative () exhibits greater stability due to saturation .
- Solubility : this compound is likely water-soluble at low pH (due to -COOH ionization) but less lipid-soluble than ether-linked analogs like 2-(2-hydroxyethoxy)acetic acid .
Q & A
Basic Research Questions
Q. What are the primary synthetic routes for alpha-hydroxyfuran-2-acetic acid, and how can reaction conditions be optimized for yield improvement?
this compound (CAS 19377-73-2) is synthesized via two primary routes:
- Route 1 : From 1-(furan-2-yl)-2,2-dihydroxy-ethanone under acidic or oxidative conditions.
- Route 2 : From furfural (糠醛) through aldol condensation followed by oxidation .
Methodological considerations : - Catalyst selection : Use transition-metal catalysts (e.g., Cu or Fe) to enhance aldol condensation efficiency.
- Solvent optimization : Polar aprotic solvents (e.g., DMF) improve intermediate stability.
- Temperature control : Reaction yields drop above 80°C due to furan ring decomposition .
Table 1 : Synthetic Route Comparison
Starting Material | Catalyst | Solvent | Yield Range | Reference |
---|---|---|---|---|
Furfural | H₂SO₄ | H₂O | 40–55% | |
1-(Furan-2-yl)-2,2-dihydroxy-ethanone | TFA | DCM | 60–75% |
Q. How can researchers validate the purity of this compound in experimental settings?
Purity validation requires:
- HPLC analysis : Use a C18 column with UV detection at 254 nm; retention time varies based on solvent polarity .
- Melting point determination : Compare observed values (e.g., 44°C for related furan derivatives) with literature data .
- Mass spectrometry (MS) : Confirm molecular ion peaks (e.g., m/z 140.14 for the parent compound) .
Advanced Research Questions
Q. What advanced spectroscopic or crystallographic techniques are suitable for structural elucidation of this compound derivatives?
- X-ray crystallography : Resolves bond angles and stereochemistry (e.g., applied to 5-(hydroxymethyl)furan-2-carboxylic acid, revealing planar furan rings and hydrogen-bonding networks) .
- ²⁵³ nm UV-Vis spectroscopy : Identifies conjugated π-systems in furan derivatives.
- Solid-state NMR : Detects polymorphic variations in crystalline forms .
Q. How can researchers address the lack of comprehensive toxicological data for this compound in experimental design?
Current gaps in toxicity data (e.g., no acute toxicity or carcinogenicity studies reported ) necessitate:
- In vitro assays : Use HepG2 cell lines to assess cytotoxicity (IC₅₀) and genotoxicity (Comet assay).
- In silico modeling : Apply tools like OECD QSAR Toolbox to predict eco-toxicity endpoints (e.g., LC₅₀ for aquatic organisms).
- Dose-ranging studies : Start with low concentrations (≤1 mM) in animal models to mitigate risks .
Q. How to resolve discrepancies in reported synthetic yields of this compound across different studies?
Yield variations arise from:
- Reagent purity : Impurities in furfural (>95% purity required) reduce aldol condensation efficiency .
- Workup protocols : Acidic quenching (pH <3) prevents byproduct formation but may degrade the product if prolonged .
- Analytical inconsistencies : Cross-validate yields via gravimetric analysis and HPLC .
Q. What strategies can be employed to study the degradation pathways of this compound under environmental conditions?
- Photodegradation studies : Expose to UV light (254 nm) and monitor via LC-MS for hydroxylated or ring-opened byproducts.
- Biodegradation assays : Use soil microbes (e.g., Pseudomonas spp.) to assess metabolic breakdown rates .
- Hydrolysis kinetics : Measure pH-dependent stability (e.g., rapid degradation at pH >10) .
Q. Methodological Challenges
Q. How to design experiments to analyze the compound’s interaction with biological macromolecules (e.g., proteins)?
- Surface plasmon resonance (SPR) : Quantify binding affinity (KD) with serum albumin or enzymes.
- Molecular docking : Use AutoDock Vina to predict binding sites on target proteins (e.g., human serum albumin) .
- Circular dichroism (CD) : Monitor conformational changes in proteins upon ligand binding .
Q. What analytical approaches are recommended for detecting trace impurities in this compound batches?
- GC-MS headspace analysis : Identifies volatile impurities (e.g., residual solvents like DCM).
- ICP-OES : Detects heavy metal contaminants (e.g., Cu or Fe from catalysts) .
- 2D-NMR (HSQC, HMBC) : Maps non-volatile impurities (e.g., diastereomers) .
Q. Data Contradictions & Validation
Q. How to reconcile conflicting reports on the compound’s stability in aqueous solutions?
- pH-dependent stability : Stability decreases above pH 7 due to deprotonation of the carboxylic group.
- Buffer selection : Use phosphate buffers (pH 6–7) to minimize hydrolysis .
- Accelerated stability testing : Store at 40°C/75% RH and monitor degradation via TLC .
Q. What validation criteria should be applied when comparing bioactivity data across studies?
- Standardized assays : Use MTT for cytotoxicity and Ames test for mutagenicity to ensure reproducibility.
- Negative controls : Include solvent-only and untreated samples to rule out artifacts .
- ECHA compliance : Align with guidelines for substance characterization (e.g., REACH protocols) .
Properties
CAS No. |
19377-73-2 |
---|---|
Molecular Formula |
C6H6O4 |
Molecular Weight |
142.11 g/mol |
IUPAC Name |
2-(furan-2-yl)-2-hydroxyacetic acid |
InChI |
InChI=1S/C6H6O4/c7-5(6(8)9)4-2-1-3-10-4/h1-3,5,7H,(H,8,9) |
InChI Key |
RTLDJXGEOSVJEX-UHFFFAOYSA-N |
SMILES |
C1=COC(=C1)C(C(=O)O)O |
Canonical SMILES |
C1=COC(=C1)C(C(=O)O)O |
Key on ui other cas no. |
19377-73-2 |
Origin of Product |
United States |
Synthesis routes and methods
Procedure details
Disclaimer and Information on In-Vitro Research Products
Please be aware that all articles and product information presented on BenchChem are intended solely for informational purposes. The products available for purchase on BenchChem are specifically designed for in-vitro studies, which are conducted outside of living organisms. In-vitro studies, derived from the Latin term "in glass," involve experiments performed in controlled laboratory settings using cells or tissues. It is important to note that these products are not categorized as medicines or drugs, and they have not received approval from the FDA for the prevention, treatment, or cure of any medical condition, ailment, or disease. We must emphasize that any form of bodily introduction of these products into humans or animals is strictly prohibited by law. It is essential to adhere to these guidelines to ensure compliance with legal and ethical standards in research and experimentation.