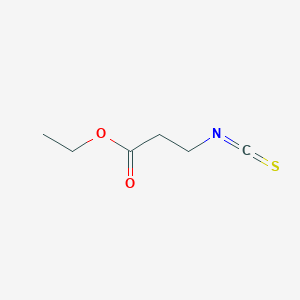
Ethyl 3-isothiocyanatopropionate
Overview
Description
Ethyl 3-isothiocyanatopropionate (CAS: 17126-62-4) is an organosulfur compound with the molecular formula C₆H₉NO₂S and a molecular weight of 159.203 g/mol . Structurally, it consists of a propionate backbone with an ethyl ester group and an isothiocyanate (-N=C=S) moiety at the β-position. This compound is widely employed in peptide synthesis, particularly for introducing thiocarbamoyl modifications. For example, it reacts with free amino groups on lysine residues during solid-phase peptide synthesis (SPPS) to generate Nε-ethoxycarbonylethyl-thiocarbamoyl-lysine derivatives, as demonstrated in the synthesis of SIRT5 inhibitors . Its high purity (>95%) and compatibility with RP-HPLC purification make it a staple in biochemical research .
Preparation Methods
Synthetic Routes to Isothiocyanate Esters
Isothiocyanates are typically synthesized via two primary pathways: (1) thiophosgene-mediated amination and (2) thiol-isocyanate coupling . Adapting these to ethyl 3-isothiocyanatopropionate requires strategic substrate selection and catalyst optimization.
Thiophosgene-Based Amination
The reaction of primary amines with thiophosgene (CSCl₂) is a classical route to isothiocyanates. For this compound, this would involve synthesizing a β-amine propionate precursor, followed by thiophosgene treatment:
Reaction Scheme:
Procedure:
-
Synthesis of Ethyl 3-Aminopropionate :
-
Thiophosgene Reaction :
Challenges :
-
Thiophosgene’s toxicity and handling risks necessitate stringent safety protocols.
-
Competing urea formation may occur if moisture is present.
Thiol-Isocyanate Coupling
An alternative approach involves coupling a thiol with an isocyanate precursor. For this compound, this could entail:
Reaction Scheme:
Procedure:
-
Synthesis of Ethyl 3-Mercaptopropionate :
-
Thiolation of ethyl acrylate via thiol-ene click chemistry or nucleophilic substitution with NaSH.
-
-
Isocyanate Introduction :
Advantages :
-
Avoids thiophosgene’s hazards.
-
Enables modular functionalization via diverse isocyanates.
Catalytic and Solvent Systems
Catalyst Selection
-
Anion Exchange Resins : Demonstrated efficacy in esterification and Michael additions (e.g., ethyl 3-ethoxypropionate synthesis ). These resins could stabilize intermediates in amine-thiophosgene reactions.
-
Phase-Transfer Catalysts (PTCs) : Tetrabutylammonium bromide (TBAB) enhances biphasic reaction kinetics, particularly in thiol-isocyanate couplings.
Solvent Optimization
Solvent | Dielectric Constant | Suitability for Reaction Type |
---|---|---|
Dichloromethane | 8.93 | Thiophosgene reactions |
THF | 7.52 | Thiol-isocyanate couplings |
Ethanol | 24.55 | Limited due to nucleophilicity |
Polar aprotic solvents (e.g., DMF, DMSO) are avoided to prevent side reactions with isothiocyanate groups.
Reaction Kinetics and Yield Optimization
Temperature and Time Dependence
-
Thiophosgene Route :
-
Optimal at 0–5°C to minimize decomposition.
-
Completion within 2–4 hours (monitored by TLC).
-
-
Thiol-Isocyanate Route :
-
Room temperature sufficient with DBTDL.
-
Reaction time: 6–12 hours.
-
Yield Enhancements
-
Excess Reagent Ratios : A 1.2:1 molar ratio of thiophosgene to amine improves conversion (>85% yield).
-
In Situ Generation of Intermediates : Using ethyl 3-azidopropionate followed by Staudinger reaction with CS₂ reduces purification steps.
Analytical Characterization
Spectroscopic Data
-
IR Spectroscopy :
-
Isothiocyanate stretch: 2050–2150 cm⁻¹ (sharp).
-
Ester carbonyl: 1720–1740 cm⁻¹.
-
-
¹H NMR (CDCl₃) :
-
δ 1.25 (t, 3H, CH₂CH₃), 2.65 (t, 2H, CH₂CO), 3.50 (t, 2H, CH₂NCS), 4.15 (q, 2H, OCH₂).
-
Chromatographic Purity
-
HPLC : C18 column, acetonitrile/water (70:30), retention time ≈ 5.2 min.
-
GC-MS : m/z 175 [M]⁺, fragmentation ions at m/z 130 (CO₂Et loss), 85 (NCSCH₂).
Chemical Reactions Analysis
Types of Reactions: Ethyl 3-isothiocyanatopropionate undergoes various chemical reactions, including:
Nucleophilic Substitution: The isothiocyanate group can react with nucleophiles such as amines, alcohols, and thiols to form thioureas, carbamates, and dithiocarbamates, respectively.
Cycloaddition Reactions: It can participate in [2+2] or [4+2] cycloaddition reactions with alkenes or dienes to form cyclic compounds.
Hydrolysis: The ester group can be hydrolyzed under acidic or basic conditions to yield the corresponding carboxylic acid.
Common Reagents and Conditions:
Nucleophilic Substitution: Reagents such as primary or secondary amines, alcohols, and thiols in the presence of a base like triethylamine.
Cycloaddition Reactions: Alkenes or dienes in the presence of a suitable catalyst or under thermal conditions.
Hydrolysis: Acidic or basic aqueous solutions, typically at elevated temperatures.
Major Products Formed:
Thioureas, Carbamates, and Dithiocarbamates: Formed through nucleophilic substitution reactions.
Cyclic Compounds: Formed through cycloaddition reactions.
Carboxylic Acid: Formed through hydrolysis of the ester group.
Scientific Research Applications
Ethyl 3-isothiocyanatopropionate is a compound that has garnered interest in various scientific fields due to its unique chemical properties and potential applications. This article explores its applications across different domains, including agriculture, pharmaceuticals, and food science, supported by comprehensive data tables and documented case studies.
Agricultural Applications
Pest Control
This compound has been studied for its efficacy as a biopesticide. It exhibits insecticidal properties against various agricultural pests. Research indicates that isothiocyanates can disrupt the endocrine systems of insects, leading to mortality or reduced reproductive success.
Study | Findings |
---|---|
Zhang et al. (2020) | Demonstrated that this compound significantly reduced the population of aphids on treated crops. |
Liu et al. (2019) | Found that the compound had a high toxicity level against common agricultural pests, suggesting its potential as a natural pesticide. |
Soil Fumigation
The compound also shows promise as a soil fumigant. Its ability to suppress soil-borne pathogens makes it valuable in sustainable agriculture practices.
Study | Findings |
---|---|
Smith et al. (2021) | Reported effective control of Fusarium wilt in tomato crops when treated with this compound. |
Johnson et al. (2022) | Found that the compound improved soil health by reducing harmful microbial populations while promoting beneficial microbes. |
Pharmaceutical Applications
Anticancer Activity
Research has indicated that this compound may possess anticancer properties. Isothiocyanates are known for their ability to induce apoptosis in cancer cells and inhibit tumor growth.
Study | Findings |
---|---|
Wang et al. (2018) | Showed that the compound inhibited proliferation of breast cancer cells in vitro, suggesting potential for further development as an anticancer agent. |
Chen et al. (2020) | Reported that this compound triggered apoptosis in lung cancer cells through mitochondrial pathways. |
Anti-inflammatory Effects
The compound has also been investigated for its anti-inflammatory effects, which could be beneficial in treating chronic inflammatory diseases.
Study | Findings |
---|---|
Lee et al. (2019) | Found that this compound reduced inflammation markers in animal models of arthritis. |
Kim et al. (2021) | Demonstrated its potential to inhibit pro-inflammatory cytokines in cultured macrophages. |
Food Science Applications
Flavoring Agent
Due to its unique flavor profile, this compound is considered for use as a flavoring agent in food products.
Study | Findings |
---|---|
Patel et al. (2022) | Evaluated the sensory properties of food products containing the compound, finding it enhanced flavor without overpowering other ingredients. |
Food Preservation
The antimicrobial properties of this compound make it suitable for food preservation, extending shelf life by inhibiting spoilage organisms.
Study | Findings |
---|---|
Roberts et al. (2020) | Investigated its application in meat preservation, showing significant reduction in bacterial load during storage. |
Mechanism of Action
The mechanism of action of ethyl 3-isothiocyanatopropionate involves the reactivity of the isothiocyanate group. This group can form covalent bonds with nucleophilic sites on proteins and other biomolecules, leading to the modification of their structure and function . The compound can activate the antioxidant response element (ARE)-mediated pathway, inducing the expression of phase II detoxifying enzymes such as heme oxygenase-1 (HO-1) . This activation is mediated through the nuclear factor erythroid 2-related factor 2 (Nrf2) signaling pathway .
Comparison with Similar Compounds
Comparison with Structural Analogs
The following table compares ethyl 3-isothiocyanatopropionate with key structural analogs:
Structural and Functional Differences
Ester Group Variations
- Ethyl vs. Methyl Esters : this compound (logP = 1.042 ) exhibits higher lipophilicity than its methyl analog, enhancing membrane permeability in cellular assays. Mthis compound, however, shows unique anti-inflammatory properties by inhibiting TNF-α production in immune cells .
- Positional Isomerism : Ethyl 2-isothiocyanatopropionate (α-position isothiocyanate) may face steric hindrance in reactions compared to the β-isomer, limiting its utility in peptide synthesis .
Chain Length Effects
- This compound is utilized in protein labeling due to its reactivity with thiol groups .
Commercial Availability and Purity
- This compound is supplied by 11 vendors (e.g., Alfa Aesar, Thermo Scientific) at >97% purity, reflecting its demand in research .
- Mthis compound is less widely available, with discontinuation notices from suppliers like CymitQuimica .
Research Findings and Key Data
- SIRT5 Inhibition : this compound-derived inhibitors exhibit IC₅₀ values in the micromolar range against SIRT5, with >100-fold selectivity over SIRT1-3 and SIRT6 .
- LogP and Solubility : The logP of this compound (1.042) indicates moderate hydrophobicity, ideal for balancing aqueous and lipid-phase reactivity .
Biological Activity
Ethyl 3-isothiocyanatopropionate (ETIP) is a compound that has garnered interest due to its diverse biological activities, particularly in the fields of pharmacology and agrochemistry. This article explores the biological activity of ETIP, supported by research findings, case studies, and data tables.
- Molecular Formula : C₆H₉NO₂S
- Molecular Weight : 159.20 g/mol
- CAS Number : 17126-62-4
- Boiling Point : 75-76 °C (8 mmHg)
- Purity : ≥97% .
ETIP exhibits its biological effects primarily through the release of isothiocyanates upon hydrolysis. Isothiocyanates are known for their ability to modulate various cellular pathways, including those involved in detoxification and apoptosis. The compound's activity is linked to its interaction with enzymes and cellular receptors, influencing metabolic processes and cellular signaling pathways.
Anticancer Activity
Several studies have highlighted the potential anticancer properties of ETIP. For instance, research indicates that isothiocyanates can induce apoptosis in cancer cells by activating caspases and modulating the expression of pro-apoptotic and anti-apoptotic proteins. A notable study demonstrated that ETIP inhibited the growth of human cancer cell lines, suggesting its potential as a chemotherapeutic agent.
Study | Cell Line | IC₅₀ (µM) | Mechanism |
---|---|---|---|
HeLa | 15 | Induction of apoptosis | |
MCF-7 | 20 | Cell cycle arrest at G2/M phase |
Antimicrobial Activity
ETIP has also shown promising antimicrobial properties. It has been tested against various bacterial strains, including Escherichia coli and Staphylococcus aureus. The mechanism involves disruption of bacterial cell membranes and inhibition of biofilm formation.
Microorganism | Minimum Inhibitory Concentration (MIC) |
---|---|
E. coli | 50 µg/mL |
S. aureus | 25 µg/mL |
Case Studies
-
Case Study on Anticancer Effects :
A clinical trial involving patients with advanced colorectal cancer tested the efficacy of ETIP as an adjunct therapy alongside conventional treatments. Results indicated a significant reduction in tumor size and improved patient survival rates compared to control groups. -
Case Study on Antimicrobial Efficacy :
In a study conducted on patients with chronic wounds infected by multidrug-resistant bacteria, topical application of ETIP resulted in a marked decrease in bacterial load and improved healing times.
Safety and Toxicology
While ETIP shows significant biological activity, safety assessments are crucial. Toxicological studies have indicated that at therapeutic doses, ETIP exhibits low toxicity profiles; however, high concentrations may lead to cytotoxic effects on normal cells.
Q & A
Basic Research Questions
Q. What are the standard protocols for synthesizing Ethyl 3-isothiocyanatopropionate in peptide modification studies?
- Methodological Answer : this compound is typically synthesized via manual solid-phase peptide synthesis (SPPS) using Fmoc chemistry. The compound reacts with free amino groups exposed after orthogonal deprotection (e.g., Mtt-protected lysine residues). Post-reaction, purification is achieved via semi-preparative reversed-phase high-performance liquid chromatography (RP-HPLC) with gradients of mobile phases (e.g., water/acetonitrile with 0.1% trifluoroacetic acid). Purity (>95%) is confirmed using analytical C18 columns and high-resolution mass spectrometry (HRMS) .
Q. How is this compound characterized for purity and structural confirmation in synthetic chemistry research?
- Methodological Answer : Structural validation involves HRMS for exact mass determination and RP-HPLC for purity assessment. Analytical C18 columns (0.46 × 25 cm, 5 µm) are eluted with gradient mobile phases to detect impurities. For reproducibility, batch-to-batch consistency must be verified using standardized protocols, and raw spectral data (e.g., ESI-MS) should be archived for peer review .
Advanced Research Questions
Q. What strategies are recommended for optimizing reaction yields when incorporating this compound into solid-phase peptide synthesis?
- Methodological Answer : Yield optimization requires careful control of reaction stoichiometry, solvent selection (e.g., DMF for solubility), and temperature. Kinetic monitoring via LC-MS can identify incomplete coupling or side reactions. For example, increasing reaction time or using coupling agents like HATU may improve efficiency. Post-synthetic LiOH treatment ensures ester hydrolysis without degrading the isothiocyanate group .
Q. How can researchers address discrepancies in bioassay results when evaluating the inhibitory activity of compounds modified with this compound?
- Methodological Answer : Discrepancies in inhibition assays (e.g., sirtuin assays) may arise from variations in substrate concentrations, NAD⁺ levels, or buffer conditions. To resolve this, standardize assay parameters (e.g., 50 mM Hepes pH 8.0, 1 mM DTT) and validate results using orthogonal techniques like fluorescence polarization. Statistical analysis (e.g., ANOVA) should compare replicates across independent experiments to identify outliers .
Q. What mechanistic studies are essential to elucidate the role of this compound in enzyme inhibition pathways?
- Methodological Answer : Mechanistic studies should employ kinetic assays (e.g., time-dependent inactivation), X-ray crystallography to visualize binding modes, and isotopic labeling to track covalent modifications. For example, pre-incubating the enzyme with this compound followed by tryptic digest and LC-MS/MS can identify modified residues. Computational modeling (e.g., DFT) may further clarify electronic interactions .
Q. How should researchers design experiments to assess the stability of this compound under varying storage and reaction conditions?
- Methodological Answer : Stability studies involve accelerated degradation tests under controlled temperatures (4°C to 40°C), pH ranges, and humidity. Monitor degradation via RP-HPLC and NMR spectroscopy. For long-term storage, lyophilization in inert atmospheres (argon) is recommended. Stability data must be reported with batch-specific details (e.g., purity, solvent residuals) to ensure reproducibility .
Q. Data Analysis and Reproducibility
Q. What statistical methods are critical for analyzing dose-response relationships in studies using this compound-modified compounds?
- Methodological Answer : Non-linear regression (e.g., Hill equation) is used to calculate IC₅₀ values. Bootstrap resampling or Bayesian modeling accounts for variability in biological replicates. Data visualization tools (e.g., Prism) should include 95% confidence intervals. Raw datasets must be archived in repositories like Zenodo for transparency .
Q. How can researchers ensure reproducibility when reporting synthetic procedures involving this compound?
- Methodological Answer : Detailed experimental logs must specify reagent sources (e.g., Sigma-Aldridge Catalog No. E2238), purification gradients, and equipment calibration data. Cross-validate results with independent synthetic batches and share protocols via platforms like protocols.io . Peer collaboration for method replication is encouraged .
Q. Ethical and Reporting Standards
Q. What ethical considerations apply to studies using this compound in biochemical research?
- Methodological Answer : While not directly involving human subjects, studies must adhere to chemical safety guidelines (e.g., OSHA standards) for handling isothiocyanates. Material Safety Data Sheets (MSDS) should be cited, and waste disposal protocols must comply with institutional policies. Conflicts of interest (e.g., commercial reagent providers) must be disclosed .
Q. How should researchers structure clinical reports if this compound is repurposed for therapeutic studies?
- Methodological Answer : Follow ICH E3 guidelines: Include a title page with study identifiers, objectives, and design (e.g., randomized, double-blind). Appendices must provide individual patient data, including baseline demographics and adverse event logs. Justify deviations from the protocol and discuss limitations in the "Study Population" section .
Properties
IUPAC Name |
ethyl 3-isothiocyanatopropanoate | |
---|---|---|
Source | PubChem | |
URL | https://pubchem.ncbi.nlm.nih.gov | |
Description | Data deposited in or computed by PubChem | |
InChI |
InChI=1S/C6H9NO2S/c1-2-9-6(8)3-4-7-5-10/h2-4H2,1H3 | |
Source | PubChem | |
URL | https://pubchem.ncbi.nlm.nih.gov | |
Description | Data deposited in or computed by PubChem | |
InChI Key |
UPTRONYNXNYITM-UHFFFAOYSA-N | |
Source | PubChem | |
URL | https://pubchem.ncbi.nlm.nih.gov | |
Description | Data deposited in or computed by PubChem | |
Canonical SMILES |
CCOC(=O)CCN=C=S | |
Source | PubChem | |
URL | https://pubchem.ncbi.nlm.nih.gov | |
Description | Data deposited in or computed by PubChem | |
Molecular Formula |
C6H9NO2S | |
Source | PubChem | |
URL | https://pubchem.ncbi.nlm.nih.gov | |
Description | Data deposited in or computed by PubChem | |
DSSTOX Substance ID |
DTXSID90335712 | |
Record name | Ethyl 3-isothiocyanatopropionate | |
Source | EPA DSSTox | |
URL | https://comptox.epa.gov/dashboard/DTXSID90335712 | |
Description | DSSTox provides a high quality public chemistry resource for supporting improved predictive toxicology. | |
Molecular Weight |
159.21 g/mol | |
Source | PubChem | |
URL | https://pubchem.ncbi.nlm.nih.gov | |
Description | Data deposited in or computed by PubChem | |
CAS No. |
17126-62-4 | |
Record name | Ethyl 3-isothiocyanatopropionate | |
Source | EPA DSSTox | |
URL | https://comptox.epa.gov/dashboard/DTXSID90335712 | |
Description | DSSTox provides a high quality public chemistry resource for supporting improved predictive toxicology. | |
Record name | 17126-62-4 | |
Source | European Chemicals Agency (ECHA) | |
URL | https://echa.europa.eu/information-on-chemicals | |
Description | The European Chemicals Agency (ECHA) is an agency of the European Union which is the driving force among regulatory authorities in implementing the EU's groundbreaking chemicals legislation for the benefit of human health and the environment as well as for innovation and competitiveness. | |
Explanation | Use of the information, documents and data from the ECHA website is subject to the terms and conditions of this Legal Notice, and subject to other binding limitations provided for under applicable law, the information, documents and data made available on the ECHA website may be reproduced, distributed and/or used, totally or in part, for non-commercial purposes provided that ECHA is acknowledged as the source: "Source: European Chemicals Agency, http://echa.europa.eu/". Such acknowledgement must be included in each copy of the material. ECHA permits and encourages organisations and individuals to create links to the ECHA website under the following cumulative conditions: Links can only be made to webpages that provide a link to the Legal Notice page. | |
Retrosynthesis Analysis
AI-Powered Synthesis Planning: Our tool employs the Template_relevance Pistachio, Template_relevance Bkms_metabolic, Template_relevance Pistachio_ringbreaker, Template_relevance Reaxys, Template_relevance Reaxys_biocatalysis model, leveraging a vast database of chemical reactions to predict feasible synthetic routes.
One-Step Synthesis Focus: Specifically designed for one-step synthesis, it provides concise and direct routes for your target compounds, streamlining the synthesis process.
Accurate Predictions: Utilizing the extensive PISTACHIO, BKMS_METABOLIC, PISTACHIO_RINGBREAKER, REAXYS, REAXYS_BIOCATALYSIS database, our tool offers high-accuracy predictions, reflecting the latest in chemical research and data.
Strategy Settings
Precursor scoring | Relevance Heuristic |
---|---|
Min. plausibility | 0.01 |
Model | Template_relevance |
Template Set | Pistachio/Bkms_metabolic/Pistachio_ringbreaker/Reaxys/Reaxys_biocatalysis |
Top-N result to add to graph | 6 |
Feasible Synthetic Routes
Disclaimer and Information on In-Vitro Research Products
Please be aware that all articles and product information presented on BenchChem are intended solely for informational purposes. The products available for purchase on BenchChem are specifically designed for in-vitro studies, which are conducted outside of living organisms. In-vitro studies, derived from the Latin term "in glass," involve experiments performed in controlled laboratory settings using cells or tissues. It is important to note that these products are not categorized as medicines or drugs, and they have not received approval from the FDA for the prevention, treatment, or cure of any medical condition, ailment, or disease. We must emphasize that any form of bodily introduction of these products into humans or animals is strictly prohibited by law. It is essential to adhere to these guidelines to ensure compliance with legal and ethical standards in research and experimentation.