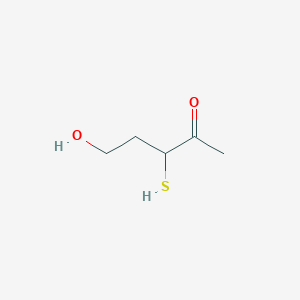
5-Hydroxy-3-sulfanylpentan-2-one
- Click on QUICK INQUIRY to receive a quote from our team of experts.
- With the quality product at a COMPETITIVE price, you can focus more on your research.
Overview
Description
5-Hydroxy-3-sulfanylpentan-2-one (C₅H₁₀O₂S, monoisotopic mass: 134.04015 Da) is a sulfur-containing ketone derivative with a hydroxyl group at the C5 position and a sulfhydryl (-SH) group at C2. It is a metabolite of thiamin (vitamin B₁) hydrolysis, generated in soil environments through microbial activity, such as by Bacillus halodurans . This compound is of interest in biochemistry and organic synthesis due to its reactive functional groups, which enable participation in redox reactions and nucleophilic substitutions.
Key Properties (from PubChemLite ):
- Molecular Formula: C₅H₁₀O₂S
- InChIKey: BEIYSLIPXPVYNV-UHFFFAOYSA-N
- Patent Count: 11 (as of 2025)
- Literature Count: 1
Preparation Methods
Catalytic Hydrothiolation of Alkenes
Cobalt-Catalyzed Thiolation Mechanisms
The hydrothiolation of alkenes via cobalt silicate (CoOx-SiOy) catalysts represents a paradigm shift from traditional H₂S-based routes. In this system, elemental sulfur (S₈) reacts with hydrogen gas under high pressure (7 MPa) to generate reactive sulfur species in situ, which undergo anti-Markovnikov addition to alkenes . For 5-hydroxy-3-sulfanylpentan-2-one, a plausible precursor is 4-hydroxy-3-penten-2-one, where the alkene moiety undergoes thiolation at the β-position. The CoOx-SiOy catalyst facilitates sulfur radical formation, enabling terminal thiol attachment while preserving the ketone and hydroxy groups .
Reaction Optimization
Key parameters for maximizing thiol yield include:
-
Sulfur stoichiometry : A 3.3:1 molar ratio of S₈ to alkene ensures complete conversion, as lower ratios (1.7:1) reduce yields by 38% .
-
Hydrogen pressure : 7 MPa optimizes H₂ dissociation on cobalt sites, whereas pressures below 5 MPa lead to polysulfane byproducts .
-
Temperature : 130°C balances reaction kinetics and selectivity, with higher temperatures (140°C) promoting desulfurization side reactions .
A representative optimization table for tetradecene thiolation illustrates these effects (Table 1) :
Condition Modification | Conversion (%) | Thiol Yield (%) | Polysulfane Yield (%) |
---|---|---|---|
Standard (7 MPa H₂, 130°C) | 99 | 64 | 24 |
H₂ reduced to 5 MPa | 99 | 54 | 20 |
H₂ reduced to 3 MPa | 99 | 18 | 81 |
Temperature increased to 140°C | 99 | 62 | 29 |
Substrate Scope and Functional Group Tolerance
The CoOx-SiOy system demonstrates remarkable compatibility with hydroxy-containing alkenes. For example, 3-methyl-3-buten-1-ol undergoes hydrothiolation to yield 3-methyl-3-sulfanylbutanol in 99% yield under optimized conditions . This suggests that the hydroxy group in 4-hydroxy-3-penten-2-one would remain intact during thiolation, avoiding protection-deprotection steps. However, steric hindrance near the ketone may necessitate tailored catalyst modifications.
Carbonyl Reduction Pathways
Thiolation of Keto-Enol Tautomers
An alternative route involves the reduction of 5-hydroxy-3-ketopentane-1-thiol precursors. Cobalt catalysts mediate the insertion of sulfur into carbonyl groups via hydrogenative desulfurization of S₈. For instance, 2-octanone reacts with S₈ and H₂ over CoOx-SiOy to form 2-octanethiol in 85% yield . Applied to 5-hydroxy-3-ketopentane-1-thiol, this method could directly install the sulfanyl group adjacent to the ketone.
Mechanistic Insights
The reaction proceeds through a keto-enol tautomerization step, where the enol form coordinates to cobalt sites, enabling nucleophilic attack by sulfur radicals (Scheme 1) :
2, \text{S}8} \text{R-C(SH)-R'}R-C(=O)-R’⇌R-C(OH)-R’H2,S8CoOx-SiOyR-C(SH)-R’
X-ray photoelectron spectroscopy (XPS) confirms that Co²⁺/Co³⁺ redox cycles mediate sulfur activation, while XRD analysis reveals the amorphous nature of CoOx-SiOy, critical for high surface area and active site accessibility .
Catalyst Design and Recyclability
Structural Characterization of CoOx-SiOy
Post-synthesis analysis of CoOx-SiOy via STEM-EDS shows a homogeneous distribution of Co, Si, and O, with a Co:Si molar ratio of 1:1.2 . After calcination at 1000°C under H₂, the catalyst crystallizes into α-Co₂SiO₄, though the amorphous phase exhibits superior activity due to defective sites enhancing sulfur adsorption .
Leaching and Recycling Performance
Leaching tests using inductively coupled plasma mass spectrometry (ICP-MS) detect <0.1% cobalt loss after five reaction cycles, affirming the catalyst’s stability . Recycled CoOx-SiOy maintains >90% thiol yield in consecutive runs, underscoring its industrial viability .
Comparative Analysis of Sulfur Sources
Elemental Sulfur vs. H₂S
Traditional H₂S-based thiolation suffers from toxicity and low yields (4–36%) due to polysulfane formation . In contrast, S₈/H₂ systems achieve yields up to 99% with minimal byproducts, as H₂ moderates sulfur chain growth .
Alternative Sulfur Donors
Thiourea and potassium thioacetate offer atom-economic routes but require stoichiometric reductants (e.g., LiAlH₄), complicating scalability . The CoOx-SiOy/S₈/H₂ system circumvents these issues through catalytic sulfur recycling.
Chemical Reactions Analysis
Types of Reactions: Flunixin-d3, like its non-deuterated counterpart, undergoes various chemical reactions, including:
Oxidation: Flunixin-d3 can be oxidized to form hydroxylated metabolites.
Reduction: The nitro group in the flunixin structure can be reduced under specific conditions.
Substitution: The aromatic ring in Flunixin-d3 can undergo electrophilic substitution reactions.
Common Reagents and Conditions:
Oxidation: Common oxidizing agents include potassium permanganate and hydrogen peroxide.
Reduction: Reducing agents such as sodium borohydride and catalytic hydrogenation are used.
Substitution: Electrophilic substitution reactions often involve reagents like halogens and nitrating agents.
Major Products Formed:
Oxidation: Hydroxylated derivatives of Flunixin-d3.
Reduction: Amino derivatives of Flunixin-d3.
Substitution: Halogenated or nitrated derivatives of Flunixin-d3.
Scientific Research Applications
Flunixin-d3 is extensively used in scientific research due to its stable isotopic labeling. Some key applications include:
Analytical Chemistry: Used as an internal standard in liquid chromatography-mass spectrometry (LC-MS) for the quantification of flunixin in biological samples.
Pharmacokinetics: Helps in studying the absorption, distribution, metabolism, and excretion (ADME) of flunixin in animals.
Veterinary Medicine: Employed in research to understand the pharmacodynamics and therapeutic efficacy of flunixin.
Toxicology: Used in studies to monitor and control flunixin residues in food-producing animals.
Mechanism of Action
Flunixin-d3, like flunixin, exerts its effects primarily through the inhibition of cyclooxygenase (COX) enzymes. This inhibition reduces the formation of prostaglandins and thromboxanes, which are mediators of inflammation, pain, and fever . The molecular targets include COX-1 and COX-2 enzymes, and the pathways involved are the arachidonic acid cascade .
Comparison with Similar Compounds
Comparison with Structurally Similar Compounds
4-Methyl-3-sulfanylpentan-2-one (CAS: 75832-79-0)
- Structure : Differs by a methyl group at C4 instead of a hydroxyl group at C3.
- Molecular Formula: C₆H₁₀OS (monoisotopic mass: 130.0453 Da)
- Reactivity : The sulfhydryl group remains reactive, but the absence of a hydroxyl group reduces polarity and hydrogen-bonding capacity compared to 5-hydroxy-3-sulfanylpentan-2-one.
- Applications : Used in flavor and fragrance industries due to its thiol-derived aroma .
5-(Furan-3-yl)-5-hydroxypentan-2-one (HMDB0030473)
- Structure : Features a furan ring at C5 instead of a sulfhydryl group.
- Molecular Formula: C₉H₁₂O₃ (monoisotopic mass: 168.0786 Da)
- Biological Role : Found in microbial metabolic pathways, though its exact function is less characterized .
Functional Analogs: Thiol-Containing Ketones
- Example : 3-Sulfanylhexan-2-one (C₆H₁₂OS)
- Comparison : Longer carbon chain increases hydrophobicity, affecting solubility and volatility. Widely studied in wine and food chemistry for its role in aroma profiles.
Data Tables
Table 1: Structural and Physicochemical Comparison
Q & A
Basic Research Questions
Q. What are the recommended analytical techniques for identifying and quantifying 5-Hydroxy-3-sulfanylpentan-2-one in microbial degradation studies?
- Methodological Answer :
- Liquid Chromatography-Mass Spectrometry (LC-MS) : Ideal for detecting sulfur-containing compounds due to high sensitivity. Use reverse-phase C18 columns with mobile phases like methanol/water (0.1% formic acid) to enhance ionization. Monitor for [M-H]⁻ ions at m/z 148.1 (calculated molecular weight: 148.24 g/mol).
- Nuclear Magnetic Resonance (NMR) : Employ 1H and 13C NMR to resolve the hydroxyl (-OH) and sulfanyl (-SH) groups. Chemical shifts for -SH typically appear at δ 1.5–2.5 ppm (1H) and δ 25–35 ppm (13C).
- Thiol-Specific Derivatization : Use Ellman’s reagent (DTNB) to quantify free thiol groups via UV-Vis spectroscopy (λ = 412 nm).
- Reference : Hydrolysis pathways involving soil microbes (e.g., Bacillus halodurans) suggest stability under neutral pH .
Q. How can researchers synthesize this compound in laboratory settings?
- Methodological Answer :
- Step 1 : Start with 3-mercaptopentan-2-one as a precursor. Protect the thiol group using tert-butyl disulfide to avoid oxidation.
- Step 2 : Introduce a hydroxyl group at the C5 position via hydroxylation using m-CPBA (meta-chloroperbenzoic acid) in dichloromethane at 0°C.
- Step 3 : Deprotect the thiol group using tributylphosphine (TBP) in methanol.
- Validation : Confirm purity via LC-MS and 1H NMR. Yield optimization requires strict oxygen-free conditions to prevent disulfide formation.
- Reference : Analogous synthesis strategies for thiol-containing ketones are validated in microbial degradation studies .
Advanced Research Questions
Q. What enzymatic pathways degrade this compound in soil ecosystems, and how can these be modeled experimentally?
- Methodological Answer :
- Key Enzymes :
- Amidohydrolase : Catalyzes deformylation of formylaminopyrimidine, a precursor in thiamin hydrolysis.
- Thiaminase II : Hydrolyzes aminopyrimidine to toxopyrimidine, indirectly influencing this compound stability.
- Experimental Design :
- Soil Microcosm Assays : Incubate soil samples with 13C-labeled thiamin. Track isotopic incorporation into this compound using GC-MS.
- Enzyme Inhibition Studies : Use sodium azide to inhibit microbial activity and isolate enzymatic contributions.
- Reference : Bacillus halodurans mediates thiamin hydrolysis to produce this compound in soil .
Q. How do pH and temperature variations affect the stability of this compound in aqueous solutions?
- Methodological Answer :
- pH Stability Assay : Prepare buffered solutions (pH 3–9) and incubate the compound at 25°C. Monitor degradation via LC-MS every 24 hours.
- Observed Trend : Degradation accelerates at pH > 7 due to thiol oxidation.
- Thermal Stability Test : Use differential scanning calorimetry (DSC) to determine melting points and decomposition thresholds (expected range: 80–100°C).
- Reference : Stability in neutral soil conditions suggests moderate resilience under environmental pH .
Properties
IUPAC Name |
5-hydroxy-3-sulfanylpentan-2-one |
Source
|
---|---|---|
Details | Computed by Lexichem TK 2.7.0 (PubChem release 2021.10.14) | |
Source | PubChem | |
URL | https://pubchem.ncbi.nlm.nih.gov | |
Description | Data deposited in or computed by PubChem | |
InChI |
InChI=1S/C5H10O2S/c1-4(7)5(8)2-3-6/h5-6,8H,2-3H2,1H3 |
Source
|
Details | Computed by InChI 1.0.6 (PubChem release 2021.10.14) | |
Source | PubChem | |
URL | https://pubchem.ncbi.nlm.nih.gov | |
Description | Data deposited in or computed by PubChem | |
InChI Key |
BEIYSLIPXPVYNV-UHFFFAOYSA-N |
Source
|
Details | Computed by InChI 1.0.6 (PubChem release 2021.10.14) | |
Source | PubChem | |
URL | https://pubchem.ncbi.nlm.nih.gov | |
Description | Data deposited in or computed by PubChem | |
Canonical SMILES |
CC(=O)C(CCO)S |
Source
|
Details | Computed by OEChem 2.3.0 (PubChem release 2021.10.14) | |
Source | PubChem | |
URL | https://pubchem.ncbi.nlm.nih.gov | |
Description | Data deposited in or computed by PubChem | |
Molecular Formula |
C5H10O2S |
Source
|
Details | Computed by PubChem 2.2 (PubChem release 2021.10.14) | |
Source | PubChem | |
URL | https://pubchem.ncbi.nlm.nih.gov | |
Description | Data deposited in or computed by PubChem | |
Molecular Weight |
134.20 g/mol |
Source
|
Details | Computed by PubChem 2.2 (PubChem release 2021.10.14) | |
Source | PubChem | |
URL | https://pubchem.ncbi.nlm.nih.gov | |
Description | Data deposited in or computed by PubChem | |
Disclaimer and Information on In-Vitro Research Products
Please be aware that all articles and product information presented on BenchChem are intended solely for informational purposes. The products available for purchase on BenchChem are specifically designed for in-vitro studies, which are conducted outside of living organisms. In-vitro studies, derived from the Latin term "in glass," involve experiments performed in controlled laboratory settings using cells or tissues. It is important to note that these products are not categorized as medicines or drugs, and they have not received approval from the FDA for the prevention, treatment, or cure of any medical condition, ailment, or disease. We must emphasize that any form of bodily introduction of these products into humans or animals is strictly prohibited by law. It is essential to adhere to these guidelines to ensure compliance with legal and ethical standards in research and experimentation.