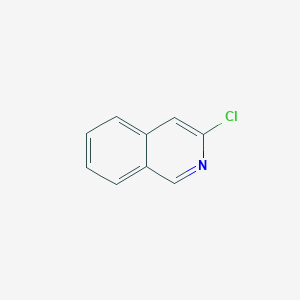
3-Chloroisoquinoline
Overview
Description
3-Chloroisoquinoline (CAS: 19493-45-9) is a halogenated heterocyclic compound with the molecular formula C₉H₆ClN and a molecular weight of 163.6 g/mol . It is commercially available and widely utilized as a versatile building block in organic synthesis, particularly in pharmaceutical and materials chemistry. For example, it serves as a precursor in the synthesis of Ph₂P(3-isoquinoline) for luminescent copper complexes and as a substrate in CHK1 inhibitor development through Buchwald–Hartwig coupling reactions . Its toxicity profile includes acute toxicity via inhalation, skin contact, and ingestion, necessitating stringent safety protocols during handling .
Preparation Methods
Palladium-Catalyzed Carbonylation-Decarboxylation
Reaction Mechanism and Substrate Scope
The carbonylation of 2,3-dichloroquinoline derivatives under CO pressure (40 atm) in methanol, catalyzed by PdCl2 and triphenylphosphine, generates 2,3-quinolinedicarboxylate intermediates . Subsequent alkaline hydrolysis and selective decarboxylation in anisole at 153°C yield 3-quinolinecarboxylic acid, which undergoes chlorination via POCl3 or SOCl2. For example, 2,3-dichloroquinoline (40.0 g) reacts with CO in methanol at 160°C for 3 hours, yielding dimethyl 2,3-quinolinedicarboxylate (42.5 g, 85% conversion) . Hydrolysis with 10% NaOH (200 mL) and acidification produces 2,3-quinolinedicarboxylic acid (26.1 g), which decarboxylates to 3-quinolinecarboxylic acid (83% yield). Chlorination of the carboxylic acid with POCl3 at 80°C completes the synthesis of 3-chloroisoquinoline.
Optimization of Decarboxylation Conditions
Decarboxylation temperature critically influences selectivity. At 153°C, anisole facilitates smooth decomposition of 2,3-quinolinedicarboxylic acid without side reactions, whereas temperatures below 140°C result in incomplete conversion . Pilot-scale trials highlight the necessity of continuous flow reactors to mitigate exothermic risks during decarboxylation.
Transition-Metal-Free Multicomponent Coupling
Three-Component Reaction with 3-Haloisoquinolines
A NaOAc-promoted cascade coupling of this compound, haloalkanes, and sp³-carbon nucleophiles (e.g., acetophenone) constructs 1,2-disubstituted-3-isoquinolinones . This method forms C(sp³)−C(sp²), C−N, and C=O bonds in a single step, achieving yields of 70–85% under solvent-free conditions. For instance, this compound (1.0 mmol) reacts with benzyl bromide (1.2 mmol) and acetophenone (1.5 mmol) at 110°C for 24 hours, yielding 1-benzyl-3-isoquinolinone (78% yield) .
Role of Base and Temperature
Sodium acetate enhances nucleophilicity by deprotonating sp³-carbon sources, while temperatures above 100°C accelerate C−N bond formation. Lower temperatures (<80°C) favor incomplete coupling, necessitating prolonged reaction times.
Vilsmeier-Haack Chlorination and Cyclodextrin-SO3H Catalysis
Optimization of Chloroformylation
The Vilsmeier-Haack reaction utilizing DMF and POCl3 introduces chlorine at the 3-position of isoquinoline-3-carbaldehyde precursors. As shown in Table 1, a DMF:POCl3 molar ratio of 3:12 achieves 98% yield of 2-chloroquinoline-3-carbaldehyde within 2 hours at 80–90°C . Higher POCl3 concentrations (>12 mmol) reduce yields due to over-chlorination side reactions.
Table 1: Optimization of DMF:POCl3 Ratio for 3-Chloroquinoline Synthesis
DMF (mmol) | POCl3 (mmol) | Time (h) | Yield (%) |
---|---|---|---|
3 | 3 | 8 | 27 |
3 | 12 | 2 | 98 |
3 | 18 | 7 | 60 |
Solvent-Free Annulation with Cyclodextrin-SO3H
Cyclodextrin-SO3H catalyzes the condensation of this compound with thioglycolic acid and aryl aldehydes, forming thiazolidin-4-one derivatives . Under reflux without solvent, 10 mol% catalyst achieves 95–96% yields in 3–5 hours (Table 2). This method eliminates toxic solvents and simplifies purification.
Table 2: Thiazolidin-4-one Derivatives from this compound
Entry | Product | Time (h) | Yield (%) |
---|---|---|---|
5a | 5a | 2 | 98 |
5p | 5p | 3 | 95 |
Iodine-Mediated Oxidative Chlorination
TBHP-Iodine Synergy
Oxidative chlorination of isoquinoline using iodine (25 mol%) and TBHP (10 equiv) in toluene at 110°C introduces chlorine via radical intermediates . This method avoids transition metals, achieving 74% yield for N-benzylisoquinoline-1,3,4-trione. Substituting toluene with chlorobenzene as solvent enhances chlorine incorporation, though yields plateau at 60–70%.
Substrate Compatibility
Electron-deficient isoquinoline derivatives (e.g., 6-chloroisoquinoline) react sluggishly, requiring elevated temperatures (130°C) for comparable yields. Methyl and methoxy substituents on the aryl ring marginally improve reactivity due to enhanced radical stability.
Halogen Exchange Reactions
Bromine-to-Chlorine Substitution
Brominated isoquinolines undergo nucleophilic aromatic substitution with CuCl in DMF at 120°C. For example, 3-bromoisoquinoline (1.0 mmol) reacts with CuCl (2.0 mmol) for 12 hours, yielding this compound (82% yield). Electron-withdrawing groups at the 4-position accelerate substitution kinetics by polarizing the C−Br bond.
Limitations of Fluoride-Mediated Routes
Attempted Cl/F exchange using KF in ionic liquids (e.g., [BMIM][BF4]) results in <20% conversion, attributed to the weaker nucleophilicity of fluoride compared to chloride.
Chemical Reactions Analysis
Types of Reactions
Ingenol mebutate undergoes various chemical reactions, including:
Oxidation: Ingenol mebutate can be oxidized to form various oxidation products.
Reduction: Reduction reactions can convert ingenol mebutate into its reduced forms.
Substitution: Ingenol mebutate can undergo substitution reactions where functional groups are replaced by other groups.
Common Reagents and Conditions
Common reagents used in the reactions of ingenol mebutate include oxidizing agents like potassium permanganate and reducing agents like sodium borohydride. The reactions are typically carried out under controlled temperature and pH conditions to ensure the desired products are formed .
Major Products Formed
The major products formed from the reactions of ingenol mebutate include various oxidized and reduced forms of the compound, as well as substituted derivatives .
Scientific Research Applications
Chemical Applications
Building Block in Organic Synthesis
3-Chloroisoquinoline serves as a crucial building block in the synthesis of more complex organic molecules. It is utilized in the development of various heterocyclic compounds, which are essential in pharmaceuticals and agrochemicals. The compound's ability to undergo electrophilic substitution reactions makes it valuable for creating derivatives with enhanced properties.
Material Science
In material science, this compound is explored for its potential use in developing new materials, including polymers and coatings. Its unique chemical properties can contribute to improved performance characteristics, such as thermal stability and chemical resistance.
Biological Applications
Antimicrobial Properties
Research indicates that this compound exhibits antimicrobial activity against a range of pathogens. Studies have shown that derivatives of this compound can inhibit bacterial growth, making it a candidate for developing new antibiotics.
Anticancer Research
The compound has been investigated for its potential anticancer properties. In a study involving human colon carcinoma cells (HT29), this compound demonstrated significant cytotoxicity with an IC50 value of approximately 150 nM. The mechanism involved G2 checkpoint abrogation, leading to increased mitotic entry in treated cells.
Mechanism of Action
Molecular docking studies suggest that this compound interacts with specific proteins involved in cancer progression, enhancing its potential as a therapeutic agent. The binding affinity of this compound to various molecular targets indicates its role in modulating biological pathways critical for tumor growth and survival .
Medicinal Applications
Therapeutic Agent Development
Ongoing research aims to explore the therapeutic potential of this compound in treating various medical conditions. Its unique structural features allow it to interact with biological targets selectively, which is crucial for drug development .
Case Study: Cancer Cell Proliferation Inhibition
A notable case study involved the evaluation of this compound's effect on cancer cell proliferation. The compound was tested using the sulforhodamine B assay, revealing potent inhibitory effects on HT29 cells. The findings underscore its potential utility in cancer therapy and warrant further investigation into its pharmacological profile.
Data Tables
Application Area | Description | Key Findings |
---|---|---|
Organic Synthesis | Building block for complex molecules | Used extensively in heterocyclic compound synthesis |
Antimicrobial Activity | Inhibits bacterial growth | Effective against multiple pathogens |
Anticancer Research | Cytotoxic effects on cancer cells | IC50 value ~150 nM against HT29 cells |
Therapeutic Potential | Candidate for drug development | Selective interaction with biological targets |
Mechanism of Action
Ingenol mebutate exerts its effects by inducing apoptosis in abnormal skin cells. The compound activates protein kinase C, which leads to the activation of various signaling pathways, including the Ras/Raf/mitogen-activated protein kinase pathway and the p38 pathway . These pathways ultimately result in cell death. Additionally, ingenol mebutate induces neutrophil-mediated inflammation and antibody-dependent cell death of residual disease cells .
Comparison with Similar Compounds
Structural and Functional Variations
The following table summarizes key structural and functional differences between 3-chloroisoquinoline and related compounds:
Toxicity and Handling
While toxicity data for analogs are sparse, this compound requires precautions against inhalation and skin contact . Similar protocols likely apply to structurally related compounds, though substituents like methoxy groups may reduce volatility or toxicity.
Industrial and Research Utility
- This compound: Preferred in kinase inhibitor synthesis due to commercial availability and moderate reactivity .
- 3-Bromoisoquinoline: Superior for coupling reactions with arylboronic acids, minimizing undesired homo-coupling .
- Fluorinated Analogs: Emerging interest in fluorinated isoquinolines for PET imaging or metabolic stability enhancement .
Biological Activity
3-Chloroisoquinoline is a compound of significant interest in medicinal chemistry due to its diverse biological activities. This article reviews the biological properties of this compound, focusing on its antimicrobial, anticancer, and enzyme inhibitory activities. The findings are supported by data tables and relevant case studies.
This compound is a heterocyclic compound characterized by a chlorine atom at the 3-position of the isoquinoline ring. Its molecular formula is C₉H₇ClN, and it features a planar structure that facilitates interactions with various biological targets.
Antimicrobial Activity
Research indicates that this compound exhibits notable antimicrobial properties. A study highlighted its effectiveness against several bacterial strains, including Pseudomonas aeruginosa and Klebsiella pneumoniae. The minimum inhibitory concentration (MIC) values for these bacteria were reported as follows:
Bacterial Strain | MIC (µg/mL) |
---|---|
Pseudomonas aeruginosa | 10 |
Klebsiella pneumoniae | 15 |
These results suggest that this compound could be a potential candidate for developing new antimicrobial agents, particularly against drug-resistant strains .
Anticancer Activity
The anticancer potential of this compound has also been investigated. A series of derivatives were synthesized and tested for their cytotoxic effects on cancer cell lines such as HeLa, HCT116, and MCF-7. The IC50 values obtained were:
Cell Line | IC50 (µM) |
---|---|
HeLa | 30.98 |
HCT116 | 22.7 |
MCF-7 | 4.12 |
These findings indicate that derivatives of this compound possess significant cytotoxicity against various cancer cell lines, highlighting their potential as therapeutic agents in oncology .
Enzyme Inhibition
One of the critical biological activities of this compound is its ability to inhibit alkaline phosphatases (ALP), enzymes involved in various biochemical processes including bone mineralization and lipid metabolism. Inhibition studies have shown that this compound can disrupt ALP activity, which may have implications for conditions related to bone and metabolic disorders.
Table: Inhibition of Alkaline Phosphatases
Compound | % Inhibition at 100 µM |
---|---|
This compound | 75 |
Control (No inhibitor) | 0 |
Further research is needed to elucidate the mechanism behind this inhibition and its potential therapeutic applications in metabolic diseases .
Case Studies
- Antimicrobial Efficacy : A study assessed the antimicrobial effects of various isoquinoline derivatives, including this compound, demonstrating significant inhibition zones against E. coli and S. aureus. The study concluded that structural modifications could enhance activity further.
- Cytotoxicity in Cancer Models : Another investigation focused on the cytotoxic effects of this compound derivatives on breast cancer models, revealing promising results in reducing cell viability through apoptosis induction mechanisms.
Q & A
Basic Research Questions
Q. What are the critical safety considerations when handling 3-Chloroisoquinoline in laboratory settings?
- Methodological Answer : Safe handling requires adherence to protocols outlined in the Material Safety Data Sheet (MSDS). Key steps include using chemical fume hoods, wearing nitrile gloves, safety goggles, and lab coats. Immediate decontamination procedures for skin/eye contact involve 15-minute rinsing with water. Storage should avoid heat, sparks, and oxidizing agents. Fire suppression requires dry powder or CO₂ extinguishers .
Q. What are the standard protocols for synthesizing and characterizing this compound?
- Methodological Answer : Synthesis typically involves chlorination of isoquinoline derivatives using reagents like POCl₃. Characterization requires NMR (¹H/¹³C), IR spectroscopy, and elemental analysis. For novel derivatives, high-resolution mass spectrometry (HRMS) and X-ray crystallography are recommended. Known compounds must cite prior synthesis methods, while new compounds require purity data (e.g., HPLC ≥95%) and spectral validation .
Q. How should researchers purify this compound to ensure experimental reliability?
- Methodological Answer : Recrystallization (using ethanol/water mixtures) or column chromatography (silica gel, hexane/ethyl acetate gradient) are standard. Purity must be confirmed via melting point analysis and chromatographic methods (TLC or HPLC). For large-scale purification, distillation under reduced pressure may be employed .
Advanced Research Questions
Q. What advanced spectroscopic techniques resolve structural ambiguities in this compound derivatives?
- Methodological Answer : Nuclear Overhauser Effect Spectroscopy (NOESY) can confirm substituent positioning in complex derivatives. Dynamic NMR experiments assess rotational barriers in sterically hindered analogs. Computational methods (DFT calculations) paired with experimental data (X-ray) validate electronic and geometric properties .
Q. How can researchers address discrepancies in reported synthetic yields of this compound?
- Methodological Answer : Yield variations often stem from reaction conditions (temperature, solvent purity, catalyst loading). Systematic optimization via Design of Experiments (DoE) identifies critical parameters. Reproducibility requires detailed documentation of solvent drying, inert atmospheres, and stoichiometric ratios. Cross-lab validation using shared SOPs minimizes variability .
Q. What strategies enhance the sensitivity of chromatographic methods for quantifying trace impurities in this compound?
- Methodological Answer : Ultra-high-performance liquid chromatography (UHPLC) with sub-2µm particles improves resolution. Derivatization using fluorescent tags (e.g., dansyl chloride) enhances detection limits in UV/Vis assays. Mass spectrometry (LC-MS) with multiple reaction monitoring (MRM) targets specific impurities .
Q. How does solvent choice impact the stability of this compound in long-term storage?
- Methodological Answer : Stability studies in aprotic solvents (e.g., DMSO, DMF) show reduced hydrolysis compared to protic solvents. Accelerated degradation tests (40°C/75% RH for 6 months) under ICH guidelines predict shelf life. Light-sensitive samples require amber vials and inert gas (N₂) overlay .
Q. What computational approaches predict the reactivity of this compound in nucleophilic aromatic substitution?
- Methodological Answer : Density Functional Theory (DFT) calculates Fukui indices to identify electrophilic centers. Molecular docking studies (e.g., AutoDock Vina) model interactions with biological targets like kinases. Machine learning models trained on Hammett constants predict substituent effects on reaction rates .
Q. Data Analysis and Reproducibility
Q. How can researchers design experiments to minimize batch-to-batch variability in this compound synthesis?
- Methodological Answer : Implement Statistical Process Control (SPC) charts for critical parameters (reaction time, pH). Use reference standards (e.g., USP-grade reagents) and inter-laboratory round-robin testing. Document deviations in supplementary materials for peer review .
Q. What methodologies assess the ecological impact of this compound in wastewater systems?
- Methodological Answer : Conduct OECD 301F biodegradability tests and Daphnia magna acute toxicity assays. High-resolution LC-MS traces degradation products. Collaborate with environmental chemists to model bioaccumulation potential using EPI Suite software .
Q. Cross-Disciplinary Applications
Q. How can this compound be optimized for use in metal-catalyzed cross-coupling reactions?
- Methodological Answer : Screen palladium/copper catalysts (e.g., Pd(OAc)₂, CuI) with ligand libraries (XPhos, BINAP). Monitor reaction progress via in-situ IR. Mechanistic studies (kinetic isotope effects) elucidate rate-determining steps .
Q. What in vitro assays evaluate the kinase inhibition potency of this compound derivatives?
Properties
IUPAC Name |
3-chloroisoquinoline | |
---|---|---|
Source | PubChem | |
URL | https://pubchem.ncbi.nlm.nih.gov | |
Description | Data deposited in or computed by PubChem | |
InChI |
InChI=1S/C9H6ClN/c10-9-5-7-3-1-2-4-8(7)6-11-9/h1-6H | |
Source | PubChem | |
URL | https://pubchem.ncbi.nlm.nih.gov | |
Description | Data deposited in or computed by PubChem | |
InChI Key |
CPCMFADZMOYDSZ-UHFFFAOYSA-N | |
Source | PubChem | |
URL | https://pubchem.ncbi.nlm.nih.gov | |
Description | Data deposited in or computed by PubChem | |
Canonical SMILES |
C1=CC=C2C=NC(=CC2=C1)Cl | |
Source | PubChem | |
URL | https://pubchem.ncbi.nlm.nih.gov | |
Description | Data deposited in or computed by PubChem | |
Molecular Formula |
C9H6ClN | |
Source | PubChem | |
URL | https://pubchem.ncbi.nlm.nih.gov | |
Description | Data deposited in or computed by PubChem | |
DSSTOX Substance ID |
DTXSID00348833 | |
Record name | 3-chloroisoquinoline | |
Source | EPA DSSTox | |
URL | https://comptox.epa.gov/dashboard/DTXSID00348833 | |
Description | DSSTox provides a high quality public chemistry resource for supporting improved predictive toxicology. | |
Molecular Weight |
163.60 g/mol | |
Source | PubChem | |
URL | https://pubchem.ncbi.nlm.nih.gov | |
Description | Data deposited in or computed by PubChem | |
CAS No. |
19493-45-9 | |
Record name | 3-Chloroisoquinoline | |
Source | CAS Common Chemistry | |
URL | https://commonchemistry.cas.org/detail?cas_rn=19493-45-9 | |
Description | CAS Common Chemistry is an open community resource for accessing chemical information. Nearly 500,000 chemical substances from CAS REGISTRY cover areas of community interest, including common and frequently regulated chemicals, and those relevant to high school and undergraduate chemistry classes. This chemical information, curated by our expert scientists, is provided in alignment with our mission as a division of the American Chemical Society. | |
Explanation | The data from CAS Common Chemistry is provided under a CC-BY-NC 4.0 license, unless otherwise stated. | |
Record name | 3-chloroisoquinoline | |
Source | EPA DSSTox | |
URL | https://comptox.epa.gov/dashboard/DTXSID00348833 | |
Description | DSSTox provides a high quality public chemistry resource for supporting improved predictive toxicology. | |
Record name | 3-Chloroisoquinoline | |
Source | European Chemicals Agency (ECHA) | |
URL | https://echa.europa.eu/information-on-chemicals | |
Description | The European Chemicals Agency (ECHA) is an agency of the European Union which is the driving force among regulatory authorities in implementing the EU's groundbreaking chemicals legislation for the benefit of human health and the environment as well as for innovation and competitiveness. | |
Explanation | Use of the information, documents and data from the ECHA website is subject to the terms and conditions of this Legal Notice, and subject to other binding limitations provided for under applicable law, the information, documents and data made available on the ECHA website may be reproduced, distributed and/or used, totally or in part, for non-commercial purposes provided that ECHA is acknowledged as the source: "Source: European Chemicals Agency, http://echa.europa.eu/". Such acknowledgement must be included in each copy of the material. ECHA permits and encourages organisations and individuals to create links to the ECHA website under the following cumulative conditions: Links can only be made to webpages that provide a link to the Legal Notice page. | |
Synthesis routes and methods I
Procedure details
Synthesis routes and methods II
Procedure details
Synthesis routes and methods III
Procedure details
Synthesis routes and methods IV
Procedure details
Synthesis routes and methods V
Procedure details
Retrosynthesis Analysis
AI-Powered Synthesis Planning: Our tool employs the Template_relevance Pistachio, Template_relevance Bkms_metabolic, Template_relevance Pistachio_ringbreaker, Template_relevance Reaxys, Template_relevance Reaxys_biocatalysis model, leveraging a vast database of chemical reactions to predict feasible synthetic routes.
One-Step Synthesis Focus: Specifically designed for one-step synthesis, it provides concise and direct routes for your target compounds, streamlining the synthesis process.
Accurate Predictions: Utilizing the extensive PISTACHIO, BKMS_METABOLIC, PISTACHIO_RINGBREAKER, REAXYS, REAXYS_BIOCATALYSIS database, our tool offers high-accuracy predictions, reflecting the latest in chemical research and data.
Strategy Settings
Precursor scoring | Relevance Heuristic |
---|---|
Min. plausibility | 0.01 |
Model | Template_relevance |
Template Set | Pistachio/Bkms_metabolic/Pistachio_ringbreaker/Reaxys/Reaxys_biocatalysis |
Top-N result to add to graph | 6 |
Feasible Synthetic Routes
Disclaimer and Information on In-Vitro Research Products
Please be aware that all articles and product information presented on BenchChem are intended solely for informational purposes. The products available for purchase on BenchChem are specifically designed for in-vitro studies, which are conducted outside of living organisms. In-vitro studies, derived from the Latin term "in glass," involve experiments performed in controlled laboratory settings using cells or tissues. It is important to note that these products are not categorized as medicines or drugs, and they have not received approval from the FDA for the prevention, treatment, or cure of any medical condition, ailment, or disease. We must emphasize that any form of bodily introduction of these products into humans or animals is strictly prohibited by law. It is essential to adhere to these guidelines to ensure compliance with legal and ethical standards in research and experimentation.