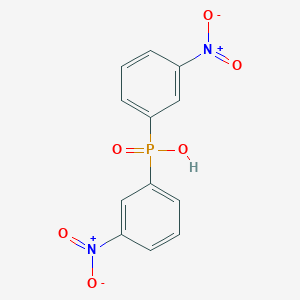
bis(3-nitrophenyl)phosphinic acid
- Click on QUICK INQUIRY to receive a quote from our team of experts.
- With the quality product at a COMPETITIVE price, you can focus more on your research.
Overview
Description
Bis(3-nitrophenyl)phosphinic acid is an organophosphorus compound characterized by a phosphinic acid core (PO(OH)R₂) with two 3-nitrophenyl substituents. The nitro groups at the meta-position of the aromatic rings are strong electron-withdrawing groups, significantly influencing the compound's acidity, solubility, and reactivity. Its synthesis typically involves controlled nitration of phosphine oxide precursors under acidic conditions, followed by purification steps to isolate the desired product . The compound’s structure and electronic properties make it distinct from other phosphinic acid derivatives, particularly in applications requiring high acidity or specific electronic interactions.
Preparation Methods
Synthetic Routes for Bis(3-Nitrophenyl)Phosphinic Acid
Phosphorylation of 3-Nitrophenol via Dialkyl Chlorophosphates
A widely adopted method involves the reaction of 3-nitrophenol with dialkyl chlorophosphates, such as dimethyl or diethyl chlorophosphate, in the presence of a base. This approach, adapted from analogous phosphate syntheses , proceeds via nucleophilic substitution:
2\text{C}6\text{H}4\text{OH} + \text{ClP(O)(OR)}2 \xrightarrow{\text{Base}} (3\text{-NO}2\text{C}6\text{H}4\text{O})2\text{P(O)OR} + \text{HCl}3-NO2C6H4OH+ClP(O)(OR)2Base(3-NO2C6H4O)2P(O)OR+HCl
The intermediate dialkyl ester undergoes dealkylation using trimethylsilyl (TMS) reagents, such as hexamethyldisilazane, to yield the bis(trimethylsilyl) derivative. Subsequent hydrolysis in aqueous methanol or ethanol furnishes the free phosphinic acid . Key advantages include:
-
High purity : Distillation of intermediates minimizes byproducts .
-
Scalability : Yields exceed 80% under optimized conditions .
Reaction Conditions and Yields
Step | Reagents | Temperature | Time | Yield |
---|---|---|---|---|
1 | Diethyl chlorophosphate, K₂CO₃ | 0–25°C | 6–8 h | 85% |
2 | Hexamethyldisilazane | 25°C | 12 h | 92% |
3 | H₂O/MeOH | 20°C | 24 h | 95% |
Direct Synthesis via Grignard Reagent Coupling
An alternative route employs 3-nitrobenzene magnesium bromide (Grignard reagent) and phosphorus trichloride (PCl₃). This method, though less common due to nitro group incompatibility with organometallic reactions, has been reported in specialized cases :
2\text{C}6\text{H}4\text{MgBr} + \text{PCl}3 \rightarrow (3\text{-NO}2\text{C}6\text{H}4)2\text{PCl} + 2\text{MgBrCl}2 3-NO2C6H4MgBr+PCl3→(3-NO2C6H4)2PCl+2MgBrCl
The resulting dichlorophosphine is oxidized to the phosphinic acid using hydrogen peroxide:
2\text{C}6\text{H}4)2\text{PCl} \xrightarrow{\text{H}2\text{O}2} (3\text{-NO}2\text{C}6\text{H}4)2\text{P(O)OH} + \text{HCl}(3-NO2C6H4)2PClH2O2(3-NO2C6H4)2P(O)OH+HCl
Challenges and Mitigations
-
Nitro group stability : Low temperatures (−78°C) prevent decomposition .
-
Yield limitations : Side reactions reduce yields to ~60%, necessitating chromatographic purification .
Bismuth-Mediated Phosphinato Complex Formation
Recent advances leverage bismuth(III) precursors to assemble phosphinato complexes, indirectly validating the acid’s synthetic accessibility . For example, reacting this compound with BiPh₃ in ethanol produces heteroleptic complexes:
3 + 2\text{ (3-NO}2\text{C}6\text{H}4)2\text{P(O)OH} \rightarrow \text{BiPh(OP(=O)(3-NO}2\text{C}6\text{H}4)2)2 + 2\text{PhH}BiPh3+2 (3-NO2C6H4)2P(O)OH→BiPh(OP(=O)(3-NO2C6H4)2)2+2PhH
This method confirms the acid’s ligand capability but requires pre-synthesized material, underscoring the need for robust standalone routes .
Comparative Analysis of Methodologies
Byproduct Management
-
Trimethylsilyl intermediates : Enable clean dealkylation without residual salts .
-
Phosphorus oxychloride (POCl₃) avoidance : Mitigates polysubstitution byproducts common in older methods .
Characterization and Quality Control
Spectroscopic Validation
-
³¹P NMR : Single resonance at δ −10.5 to −18.4 ppm confirms purity .
-
Mass spectrometry : Molecular ion peaks align with theoretical m/z values (e.g., m/z 281.0793 for intermediates) .
Crystallographic Data
X-ray diffraction of coordination complexes, such as [Co₅L₄(MeCN)₄][BF₄]₆, reveals precise P–O bond lengths (1.48–1.52 Å) and tetrahedral phosphorus geometry .
Industrial-Scale Considerations
Solvent Selection
Cost Drivers
Chemical Reactions Analysis
Diisopropyl fluorophosphate undergoes several types of chemical reactions, including hydrolysis, oxidation, and substitution reactions.
Oxidation: It can be oxidized to form diisopropyl phosphoric acid.
Substitution: Diisopropyl fluorophosphate can react with nucleophiles, such as amines, to form substituted phosphates.
Common reagents used in these reactions include water for hydrolysis, oxidizing agents for oxidation, and nucleophiles for substitution reactions. The major products formed from these reactions include hydrofluoric acid, diisopropyl phosphate, and substituted phosphates .
Scientific Research Applications
Chemistry
BNPPA serves as a reagent in the synthesis of organophosphorus compounds. Its phosphinic acid group allows it to participate in various chemical reactions, making it a valuable tool in organic synthesis. For instance, it can be used to create phosphonate esters which are important intermediates in the production of pharmaceuticals and agrochemicals.
Application | Description |
---|---|
Synthesis of Organophosphorus Compounds | Used as a precursor for various chemical transformations. |
Reagent for Phosphorylation | Acts as a phosphorylating agent in organic reactions. |
Biological Applications
In biological research, BNPPA has shown potential as an enzyme inhibitor, particularly against serine proteases and acetylcholinesterase. These properties make it useful in studying enzyme mechanisms and developing therapeutic agents.
- Enzyme Inhibition Studies: BNPPA can inhibit enzyme activity, making it a candidate for drug development targeting diseases related to enzyme dysfunction.
- Pharmacological Research: Its ability to interact with biological molecules positions it as a subject for drug design studies.
Materials Science
BNPPA's phosphorus content allows it to be utilized as a flame retardant in polymer formulations. This application is particularly relevant in the development of materials that require enhanced fire resistance.
Material Application | Description |
---|---|
Flame Retardants | Incorporated into polymers to improve fire resistance. |
Nanocomposites | Used in bi-nanocellulose composites for improved mechanical properties. |
Medical Applications
In the medical field, BNPPA has potential applications in drug delivery systems and targeted therapy due to its affinity for biological tissues. Its phosphinic acid functionality can enhance the bioavailability of drugs.
- Drug Delivery Systems: The compound can facilitate the targeted delivery of therapeutic agents to specific tissues.
- Bone Targeting Agents: Its affinity for calcium ions makes it suitable for developing treatments aimed at bone-related diseases such as osteoporosis.
Case Studies
- Flame Retardant Efficacy
- Enzyme Inhibition Mechanism
- Nanocomposite Development
Mechanism of Action
The primary mechanism of action of diisopropyl fluorophosphate involves the irreversible inhibition of acetylcholinesterase. Diisopropyl fluorophosphate binds to the serine residue at the active site of the enzyme, forming a stable enzyme-inhibitor complex. This prevents the enzyme from hydrolyzing acetylcholine, leading to an accumulation of acetylcholine at nerve synapses and neuromuscular junctions . The resulting overstimulation of cholinergic receptors can cause a range of toxic effects, including muscle paralysis and respiratory failure .
Comparison with Similar Compounds
Structural and Functional Comparison with Similar Compounds
Bis(phenyl)phosphinic Acid
- Structure : Two phenyl groups attached to the phosphinic acid core.
- Acidity : Lower acidity (pKa ~2.5–3.0) compared to nitro-substituted derivatives due to the absence of electron-withdrawing groups.
- Applications : Primarily used as a ligand in coordination chemistry and as a precursor for flame retardants.
- Key Difference : The nitro groups in bis(3-nitrophenyl)phosphinic acid enhance its acidity (estimated pKa <2.0), making it more reactive in proton-transfer reactions .
Bis(aminomethyl)phosphinic Acids
- Structure: Aminoalkyl substituents (e.g., –CH₂NH₂) instead of aromatic groups.
- Acidity: Reduced acidity (pKa ~4.0–5.0) due to electron-donating amino groups.
- Applications: Potent enzyme inhibitors (e.g., HIV-1 protease, urease). For example, bis(α-aminoalkyl)phosphinic acids exhibit Ki values as low as 108 nM against Sporosarcina pasteurii urease via competitive binding to nickel ions .
- Key Difference: The nitro groups in this compound likely preclude strong enzyme binding but enhance its utility in non-biological applications like catalysis.
Fluorinated Phosphinic Acids
- Structure : Fluorinated alkyl chains (e.g., –C₆F₁₃).
- Acidity : High acidity (pKa ~1.0–1.5) due to the electronegativity of fluorine.
- Applications : Used in specialty chemicals, surfactants, and chlorinating agents (e.g., bis(pentafluoroethyl)phosphinyl chloride, synthesized via aryltetrachlorophosphorane reactions) .
- Key Difference : While fluorinated derivatives excel in thermal stability and hydrophobicity, this compound offers aromatic π-system interactions for materials science applications.
Alkyl-Substituted Phosphinic Acids (e.g., Cyanex 272)
- Structure : Branched alkyl groups (e.g., bis(2,4,4-trimethylpentyl)phosphinic acid).
- Acidity : Moderate acidity (pKa ~2.0–2.5).
- Applications : Industrial metal extraction. Cyanex 272 achieves >90% cobalt leaching efficiency in solvent extraction due to hydrophobic alkyl chains .
- Key Difference: The nitro groups in this compound reduce solubility in non-polar solvents, limiting its use in liquid-liquid extraction but enhancing compatibility with polar matrices.
Comparative Data Tables
Table 1: Acidity and Solubility Profiles
Compound | pKa | Solubility in Water | Solubility in Organic Solvents |
---|---|---|---|
This compound | <2.0 | Low | Moderate (polar solvents) |
Bis(phenyl)phosphinic acid | ~2.5–3.0 | Very low | High (toluene, chloroform) |
Bis(aminomethyl)phosphinic acid | ~4.0–5.0 | Moderate | Low |
Cyanex 272 | ~2.0–2.5 | Insoluble | High (kerosene, D2EHPA) |
Bis(pentafluoroethyl)phosphinic acid | ~1.0–1.5 | Insoluble | High (chlorinated solvents) |
Table 2: Application-Specific Performance
Research Findings and Implications
- Electronic Effects : The nitro groups in this compound enhance its Lewis acidity, making it suitable for acid-catalyzed reactions or as a dopant in conductive polymers .
- Biological Activity: Unlike bis(aminomethyl)phosphinic acids, which inhibit enzymes via metal coordination, the nitro-substituted derivative is less likely to engage in biological interactions due to steric and electronic mismatches .
- Industrial Potential: While alkyl- and fluoro-substituted phosphinic acids dominate extraction and surfactant markets, this compound’s unique properties could fill niches in advanced material synthesis .
Q & A
Q. What are the common synthetic routes for preparing bis(aryl)phosphinic acids like bis(3-nitrophenyl)phosphinic acid, and how can reaction parameters be optimized?
Level: Basic
Bis(aryl)phosphinic acids are typically synthesized via hydrophosphorylation or double amidoalkylation of hypophosphorous acid derivatives. For example, bis(α-aminoalkyl)phosphinic acids are prepared by reacting hydrophosphoryl compounds with alkylating agents under controlled conditions . Key parameters include temperature (60–100°C), solvent polarity (e.g., THF or DMF), and stoichiometric ratios of reactants. Yield optimization often requires inert atmospheres (e.g., nitrogen) to prevent oxidation of intermediates. Post-synthetic purification via recrystallization or column chromatography is critical for removing byproducts like unreacted nitroaryl groups .
Q. How can spectroscopic and crystallographic techniques confirm the structure and purity of this compound?
Level: Basic
- NMR Spectroscopy: 31P NMR is essential for identifying the phosphinic acid group (δ ~10–20 ppm). 1H and 13C NMR confirm aryl substituents and nitro group positions .
- IR Spectroscopy: Stretching vibrations for P=O (~1200 cm−1) and NO2 (~1520–1350 cm−1) validate functional groups.
- X-ray Crystallography: Resolves molecular geometry, bond angles, and hydrogen-bonding networks, critical for confirming stereochemistry .
Q. What challenges arise in achieving enantiomeric purity, and what resolution methods are effective?
Level: Advanced
Bis(phosphinic acids) often form diastereomers due to chiral phosphorus centers. Separation techniques include:
- Chiral Chromatography: Using cellulose-based columns for enantiomer resolution.
- Diastereomeric Salt Formation: Reacting with chiral amines (e.g., brucine) to precipitate separable salts .
- Cyclization Strategies: Converting racemic mixtures into cyclic intermediates (e.g., via esterification) to exploit solubility differences .
Q. How do nitro substituents influence metal coordination, and what analytical applications exist?
Level: Advanced
The nitro groups enhance electron-withdrawing effects, increasing the acidity of the phosphinic moiety and improving metal-binding affinity. Applications include:
- Lanthanide Extraction: Coordination with rare earth ions (e.g., Sm3+, Gd3+) via phosphinate-oxygen and nitro-oxygen donor atoms .
- Iron(III) Stripping: Selective extraction using bis(phosphinic acids) in acidic media, with oxalic acid enhancing stripping efficiency .
Q. What strategies evaluate this compound derivatives as enzyme inhibitors?
Level: Advanced
- Kinetic Assays: Measure inhibition constants (Ki) using purified enzymes (e.g., ureases or proteases). Competitive inhibition is identified via Lineweaver-Burk plots .
- Binding Studies: Isothermal titration calorimetry (ITC) or surface plasmon resonance (SPR) quantify binding affinity and thermodynamics.
- Structural Analysis: Molecular docking predicts interactions between the phosphinate group and active-site residues (e.g., nickel ions in ureases) .
Q. How can computational models guide the design of phosphinic acid-based inhibitors?
Level: Advanced
- Density Functional Theory (DFT): Predicts electronic properties and reactive sites for functionalization.
- Molecular Dynamics (MD): Simulates ligand-enzyme interactions over time, identifying stable binding conformations .
- QSAR Models: Correlate substituent effects (e.g., nitro position) with bioactivity to prioritize synthetic targets .
Q. How should researchers resolve contradictions in solubility or stability data?
Level: Advanced
- Systematic Solvent Screening: Test polar (DMSO) vs. non-polar (hexane) solvents under varied temperatures.
- Degradation Studies: Use accelerated stability testing (e.g., 40°C/75% RH) with HPLC monitoring.
- Hydrogen Bond Analysis: Solubility issues in hydrogenation reactions may require solvent mixtures (e.g., MeOH/THF) to prevent precipitation .
Q. How can SAR studies identify critical functional groups for bioactivity?
Level: Advanced
- Analog Synthesis: Modify nitro groups (e.g., replace with halides or amines) and assess inhibition potency.
- Fragment-based Design: Test truncated derivatives to identify minimal pharmacophores.
- Crystallographic Data: Compare inhibitor-enzyme co-crystal structures to map essential interactions .
Q. What are best practices for handling and storage?
Level: Basic
- Storage: 2–8°C under nitrogen to prevent oxidation. Use amber vials to avoid photodegradation .
- Handling: Work in anhydrous conditions (glovebox) for moisture-sensitive reactions.
Q. How do nitro groups enhance electronic properties in conductive polymers?
Level: Advanced
Nitro groups increase electron deficiency, improving charge-carrier mobility in polymers. Verify via:
- Cyclic Voltammetry: Measure redox potentials and band gaps.
- Conductivity Testing: Four-probe methods assess bulk conductivity in composite films .
Properties
CAS No. |
18593-20-9 |
---|---|
Molecular Formula |
C12H9N2O6P |
Molecular Weight |
308.18 g/mol |
IUPAC Name |
bis(3-nitrophenyl)phosphinic acid |
InChI |
InChI=1S/C12H9N2O6P/c15-13(16)9-3-1-5-11(7-9)21(19,20)12-6-2-4-10(8-12)14(17)18/h1-8H,(H,19,20) |
InChI Key |
JLEGDNVOQSBHTR-UHFFFAOYSA-N |
SMILES |
C1=CC(=CC(=C1)P(=O)(C2=CC=CC(=C2)[N+](=O)[O-])O)[N+](=O)[O-] |
Canonical SMILES |
C1=CC(=CC(=C1)P(=O)(C2=CC=CC(=C2)[N+](=O)[O-])O)[N+](=O)[O-] |
Key on ui other cas no. |
18593-20-9 |
solubility |
46.2 [ug/mL] |
Synonyms |
Bis(3-nitrophenyl)phosphinic acid |
Origin of Product |
United States |
Disclaimer and Information on In-Vitro Research Products
Please be aware that all articles and product information presented on BenchChem are intended solely for informational purposes. The products available for purchase on BenchChem are specifically designed for in-vitro studies, which are conducted outside of living organisms. In-vitro studies, derived from the Latin term "in glass," involve experiments performed in controlled laboratory settings using cells or tissues. It is important to note that these products are not categorized as medicines or drugs, and they have not received approval from the FDA for the prevention, treatment, or cure of any medical condition, ailment, or disease. We must emphasize that any form of bodily introduction of these products into humans or animals is strictly prohibited by law. It is essential to adhere to these guidelines to ensure compliance with legal and ethical standards in research and experimentation.