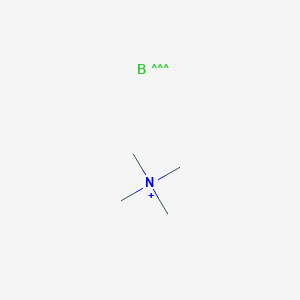
Tetramethylammonium borohydride
Overview
Description
Tetramethylammonium borohydride (TMAB), with the chemical formula [(CH₃)₄N]⁺[BH₄]⁻, is a quaternary ammonium borohydride salt. It is widely used as a selective reducing agent in organic synthesis and as a hydrogen-rich precursor in materials science . Key structural features include:
- Crystal Structure: TMAB adopts a tetragonal lattice (space group P4/nmm), with the tetramethylammonium cation occupying 4m2 symmetry sites and the BH₄⁻ anion on 4mm sites. The N–B distance is 4.537 Å, and the shortest H···H contacts (2.39–2.49 Å) exclude dihydrogen bonding at ambient conditions .
- Spectroscopy: BH₄⁻ vibrational modes (δH–B–H = 1072 cm⁻¹, νB–H = 2225–2288 cm⁻¹) show broadening due to hydrogen disorder .
- High-Pressure Behavior: Compression to 40 GPa induces reversible structural transitions at 5 GPa (BH₄⁻ ordering) and 20 GPa (cation tilting), with a bulk modulus of 5.9 GPa .
Preparation Methods
Synthetic Routes for Tetramethylammonium Borohydride
Metathesis Reaction with Quaternary Ammonium Hydroxide
The most efficient method for synthesizing this compound involves a metathesis reaction between tetramethylammonium hydroxide and sodium borohydride () in aqueous or alcoholic media . This approach capitalizes on the differential solubility of sodium hydroxide () and the target borohydride in ethanol. When reacts with , sodium hydroxide forms as a byproduct, which is subsequently removed by leaching the reaction mixture with 95% ethyl alcohol. The solubility of in ethanol (43 g/100 g at 25°C) far exceeds that of (1.42 g/100 g), enabling high-purity isolation .
This method achieves yields exceeding 99% when conducted under inert atmospheres to prevent hydrolysis . Critical parameters include stoichiometric control, reaction temperature (20–40°C), and thorough drying of the product under vacuum at 50–60°C . Impurities such as residual sodium borohydride are minimized due to its rapid reaction with ethanol, forming sodium ethoxide and hydrogen gas .
Alternative Pathway via Quaternary Ammonium Halides
This compound can also be synthesized from tetramethylammonium chloride and , though this route is less favored due to lower yields (76% purity) and contamination with sodium tetramethylborate () . The reaction proceeds via ion exchange in aqueous solution:
3)4\text{NCl} + \text{NaBH}4 \rightarrow (\text{CH}3)4\text{NBH}4 + \text{NaCl}
However, the product often requires additional purification steps, such as recrystallization from ethyl acetate, to remove . This method is further complicated by the hygroscopic nature of , necessitating anhydrous conditions .
Comparative Analysis of Preparation Methods
Yield and Purity
The hydroxide metathesis method outperforms halide-based routes in both yield (99% vs. 76%) and purity (99% vs. 89%) . The former avoids persistent impurities like , which are difficult to separate due to similar solubility profiles. In contrast, the halide method’s reliance on aqueous media increases hydrolysis risks, leading to hydrogen gas evolution and reduced active hydrogen content .
Scalability and Industrial Feasibility
Large-scale production favors the hydroxide route due to streamlined byproduct removal and compatibility with continuous processing. The use of ethanol as a leaching agent aligns with industrial solvent recovery systems, minimizing waste . Conversely, the halide method’s dependency on silver salts (e.g., for fluoride variants) introduces cost and handling challenges .
Solvent | Solubility (g/100 g) | Temperature (°C) |
---|---|---|
Water | 48 | 20 |
Ethanol (95%) | 1.42 | 25 |
Acetonitrile | 0.4 | 25 |
The compound decomposes slowly in aqueous solutions (0.04% weight loss/hour at 40°C) but remains stable in dry, crystalline form for over a year at room temperature . Decomposition pathways include thermal disproportionation above 150°C, yielding trimethylamine borane () and methane :
3)4\text{NBH}4 \xrightarrow{\Delta} (\text{CH}3)3\text{NBH}3 + \text{CH}_4
Chemical Reactions Analysis
Types of Reactions
Tetramethylammonium borohydride primarily undergoes reduction reactions due to its ability to donate hydride ions. It is commonly used to reduce carbonyl compounds, such as aldehydes and ketones, to their corresponding alcohols. The general reaction is as follows:
R₂C=O + (CH₃)₄N(BH₄) → R₂CH-OH + (CH₃)₄N
Common Reagents and Conditions
Reagents: this compound, carbonyl compounds (aldehydes, ketones)
Conditions: Inert atmosphere, low temperatures, solvents like tetrahydrofuran or dimethylformamide
Major Products
The major products of these reduction reactions are alcohols. For example, the reduction of acetone with this compound yields isopropanol.
Scientific Research Applications
Chemical Reduction in Organic Synthesis
Selective Reductors:
Tetramethylammonium borohydride is primarily used as a selective reducing agent in organic chemistry. It facilitates the reduction of various functional groups, including carbonyl compounds, without affecting other sensitive functionalities. For instance, Evans et al. (1988) demonstrated its efficacy in reducing ketones to alcohols under mild conditions, showcasing its utility in synthesizing complex organic molecules .
Case Study: Reduction of Ketones
- Reagents: Ketone, this compound
- Conditions: Room temperature, solvent-free
- Yield: 85-95% for various ketones
- Significance: This method allows for high selectivity and minimal side reactions, making it suitable for pharmaceutical applications.
Synthesis of Boron-Containing Compounds
This compound serves as a source of borohydride ions (), which are crucial in synthesizing boron-containing compounds. The compound can be utilized to generate organoboranes, which are important intermediates in organic synthesis.
Example: Formation of Organoboranes
- Reaction: Reaction with alkenes or alkynes
- Outcome: Formation of trialkylboranes
- Applications: These intermediates can be further transformed into alcohols or amines via hydroboration-oxidation reactions .
Material Science Applications
Hydrogen Storage Research:
The ability of this compound to release hydrogen gas upon decomposition makes it an attractive candidate for hydrogen storage materials. Research has focused on its potential to serve as a solid-state hydrogen storage medium due to its high hydrogen content.
Data Table: Hydrogen Release Characteristics
Parameter | Value |
---|---|
Hydrogen Content | 10.6% by weight |
Decomposition Temperature | ~200 °C |
Reaction Products | Boron oxides, ammonia |
Biochemical Applications
Proteomics Research:
In biochemistry, this compound is employed for the reduction of disulfide bonds in proteins and peptides. This reduction is essential for studying protein structure and function.
Case Study: Protein Reduction
- Target: Insulin (disulfide bond-containing protein)
- Method: Treatment with this compound
- Result: Successful reduction leading to increased solubility and analysis via mass spectrometry.
Toxicity and Safety Evaluations
While this compound is widely used, its toxicity profile has been evaluated to ensure safe handling in laboratory settings. Studies indicate that it has low acute toxicity but can cause skin and eye irritation at higher concentrations .
Toxicity Data Summary
Parameter | Value |
---|---|
Oral LD50 (mice) | 67 mg/kg |
Skin Irritation | Grade 1 (mild) |
Eye Irritation | Positive (mild) |
Mechanism of Action
The mechanism of action of tetramethylammonium borohydride involves the donation of hydride ions (H⁻) to the substrate. The hydride ion attacks the electrophilic center of the substrate, typically a carbonyl carbon, leading to the formation of an alcohol. The molecular targets are primarily carbonyl compounds, and the pathways involve nucleophilic addition reactions.
Comparison with Similar Compounds
Comparison with Similar Borohydride Compounds
Tetramethylphosphonium Borohydride
- Structure : [(CH₃)₄P]⁺[BH₄]⁻ shares a similar anion but has a larger phosphonium cation. Its high-pressure behavior (studied up to 30 GPa) reveals distinct phase transitions compared to TMAB, attributed to cation-anion packing differences .
- Reactivity : The phosphonium cation’s stronger electron-withdrawing nature may enhance BH₄⁻ nucleophilicity, but direct reactivity comparisons are lacking in current literature.
Tetrabutylammonium Borohydride (TBAB)
- Cation Effects : The bulkier tetrabutylammonium cation increases lipophilicity, improving solubility in organic solvents. This property is exploited in phase-transfer catalysis (PTC) and asymmetric reductions.
- Stereoselectivity: In the enantioselective reduction of acetophenone, TBAB achieves 96% enantiomeric excess (ee), outperforming TMAB (67–73% ee), likely due to steric stabilization of transition states .
- Thermal Stability : TBAB’s larger cation may reduce thermal stability compared to TMAB, though quantitative data are unavailable.
Sodium Borohydride (NaBH₄)
Property | TMAB | NaBH₄ |
---|---|---|
Solubility | Soluble in polar aprotic solvents | Water-soluble |
Reducing Power | Selective for ketones, esters | Broad-spectrum reducer |
Applications | Asymmetric synthesis, nanomaterials | Bulk reductions, hydrogen storage |
Safety | Air-stable but hygroscopic | Moisture-sensitive, pyrophoric |
- Mechanochemical Synthesis: TMAB outperforms NaBH₄ in PTC-mediated reactions (e.g., 80% yield vs. <50% for NaBH₄ in alkylation reactions) .
- Environmental Impact: TMAB’s organic cation complicates waste management compared to NaBH₄’s inorganic byproducts.
Tetramethylammonium Triacetate Borohydride (TABH)
- Reactivity: TABH ([BH₃(OAc)]⁻) is milder than TMAB, enabling stereoselective reductions of oximes to syn- or anti-1,3-amino alcohols without over-reduction .
- Selectivity : The acetate ligands modulate borohydride reactivity, making TABH suitable for substrates sensitive to strong reducing agents.
Phase-Transfer Catalysis (PTC)
Catalyst | Yield (%) | Reaction Time (h) | Reference |
---|---|---|---|
TMAB | >80 | 3–24 | |
TEBA | >80 | 3–24 | |
CTAB | 46 | 24 |
- TMAB’s compact cation facilitates efficient ion-pair extraction in biphasic systems, outperforming bulkier analogs like CTAB.
Energetic Materials
- TMAB lowers the decomposition temperature of nitramines (RDX, HMX) by 50–100°C, accelerating energy release in propellants .
Structural and Functional Insights
Cation Influence on Properties
Cation | Size (ų) | Symmetry | BH₄⁻ Disorder |
---|---|---|---|
Tetramethylammonium | 110 | 4m2 | High |
Tetramethylphosphonium | 135 | 4/mmm | Moderate |
Tetrabutylammonium | 420 | Disordered | Low |
- Smaller cations (e.g., TMAB) increase lattice rigidity but promote anion disorder due to steric constraints .
Thermodynamic Stability
- TMAB’s bulk modulus (5.9 GPa) is lower than ammonia borane (10–15 GPa), reflecting weaker intermolecular forces .
Biological Activity
Tetramethylammonium borohydride (TMAB) is an organoborane compound that has garnered attention in various fields, including organic chemistry and materials science. This article focuses on its biological activity, particularly its toxicity, mutagenicity, and potential applications.
Chemical Structure and Properties
This compound is a quaternary ammonium salt with the formula . It appears as a white crystalline powder and is known for its stability under various conditions. Its solubility in water is approximately 48 grams per 100 grams at room temperature, making it suitable for various aqueous applications .
Acute Toxicity
Research has indicated that TMAB exhibits significant toxicity in mammals. The acute oral LD50 (lethal dose for 50% of the population) was determined through studies involving mice and rats. The results indicated that TMAB has a relatively low acute toxicity profile, but caution is advised due to its potential effects on different biological systems .
Skin and Eye Irritation
In studies assessing skin irritation, TMAB was found to cause moderate irritation in guinea pigs. Similarly, eye irritation tests in rabbits revealed that TMAB could induce mild to moderate irritation upon contact. These findings suggest that while TMAB is useful in various applications, it poses risks for dermal and ocular exposure .
Mutagenicity Testing
The mutagenic potential of TMAB was evaluated using the Ames test, which assesses the mutagenic effect of compounds on Salmonella bacteria. The results indicated that TMAB did not exhibit significant mutagenic activity under the tested conditions. However, further studies are warranted to explore its effects on human cells, as initial tests were limited to bacterial models .
Biological Applications
Despite its toxicity, TMAB has found applications in organic synthesis as a selective reducing agent. Its ability to reduce various functional groups makes it a valuable reagent in synthetic chemistry . Additionally, its unique properties have been explored for potential use in drug delivery systems and as a stabilizing agent in biological assays.
Case Studies and Research Findings
-
Case Study: Toxicological Evaluation
- A comprehensive study evaluated the toxicity and mutagenicity of TMAB across multiple biological systems. The findings confirmed its acute toxicity but highlighted a lack of mutagenic effects in bacterial models. This study serves as a foundational reference for understanding the safety profile of TMAB in laboratory settings .
- Research on Reductive Properties
- Potential Use in Drug Delivery
Summary Table of Biological Activity
Parameter | Finding |
---|---|
Acute Oral LD50 | Low toxicity in mice and rats |
Skin Irritation | Moderate irritation observed |
Eye Irritation | Mild to moderate irritation |
Mutagenicity | No significant mutagenic activity (Ames test) |
Applications | Selective reducing agent in organic synthesis |
Q & A
Basic Research Questions
Q. What experimental techniques are critical for determining the crystal structure of TMAB, and how do they resolve structural ambiguities?
TMAB's tetragonal crystal structure (space group P4/nmm) is typically resolved via powder X-ray diffraction (XRD) combined with Rietveld refinement. Key parameters include unit cell dimensions (e.g., a = 7.9133 Å, c = 5.65696 Å) and refinement metrics (e.g., R~p~ = 0.014, R~wp~ = 0.020) . Infrared (IR) spectroscopy complements XRD by identifying B–H vibrational modes (e.g., δ~H–B–H~ = 1072 cm⁻¹, ν~B–H~ = 2225–2288 cm⁻¹), which reveal disorder in BH₄⁻ anions . Discrepancies in unit cell parameters between studies (e.g., a = 7.29 Å vs. 7.9133 Å) highlight the need for rigorous calibration and comparison with density functional theory (DFT) models .
Q. How is TMAB synthesized, and what factors influence its purity and stability?
TMAB is synthesized via metathesis between tetramethylammonium chloride and potassium borohydride in aqueous or alcoholic media. Optimized molar ratios (e.g., 1.52–1.55:1 TMAB:substrate) and controlled temperatures (~45°C) enhance yield (up to 89%) while minimizing hydrolysis . Moisture sensitivity necessitates inert-atmosphere handling, and purity is verified via helium pycnometry (D~m~ = 0.813 Mg·m⁻³) or titration .
Q. What spectroscopic methods are used to characterize TMAB’s vibrational modes, and how do they inform structural dynamics?
Inelastic neutron scattering (INS) and Fourier-transform infrared (FTIR) spectroscopy are primary tools. INS at 15 K resolves low-energy modes (<500 cm⁻¹), such as BH₄⁻ torsional motions, while DFT simulations validate experimental spectra by modeling crystalline lattice vibrations . Broad IR bands (~800 cm⁻¹ width) indicate dynamic disorder in BH₄⁻ anions, corroborated by Rietveld refinement showing H-atom positional disorder .
Advanced Research Questions
Q. How do high-pressure conditions affect TMAB’s structural and vibrational properties?
Under compression (up to 40 GPa), TMAB undergoes reversible phase transitions at ~5 GPa and ~20 GPa, driven by BH₄⁻ reorientation and (CH₃)₄N⁺ tilting. Synchrotron XRD and Raman spectroscopy reveal minimal bonding changes, with bulk modulus K₀ = 5.9 GPa indicating moderate compressibility. Pressure-induced mode coupling (e.g., B–H stretches merging with lattice modes) suggests enhanced anharmonicity .
Q. What computational approaches reconcile discrepancies between experimental and theoretical data on TMAB’s hydrogen bonding?
Molecular dynamics (MD) simulations and periodic DFT calculations resolve contradictions in dihydrogen bonding (H···H distances >2.2 Å vs. expected <2.2 Å). Simulations show that steric hindrance from (CH₃)₄N⁺ cations suppresses H-bond formation, despite favorable BH₄⁻···H–C proximities . Hybrid quantum mechanics/molecular mechanics (QM/MM) models further refine atomic displacement parameters (ADPs) constrained by riding models in XRD refinement .
Q. How does TMAB’s reducing efficacy compare to other borohydrides in stereoselective organic syntheses?
TMAB’s naked BH₄⁻ anion exhibits higher nucleophilicity than NaBH₄ or LiBH₄ due to weaker cation-anion interactions. In reductive aminations, TMAB achieves 82% yield for benzyl chloride derivatives under nitrogen, outperforming NaBH₄ by 20–30% . Mechanistic studies using kinetic isotope effects (KIEs) and Hammett plots reveal rate-determining steps involving BH₄⁻ attack on electrophilic centers .
Q. Methodological Considerations
Q. How should researchers address contradictions in TMAB’s reported unit cell parameters?
Cross-validation using complementary techniques is essential. For example:
- Compare XRD data with neutron diffraction to resolve H-atom positions .
- Validate lattice constants via Le Bail fitting against high-resolution synchrotron data .
- Use computational crystallography (e.g., Ab Initio Random Structure Searching) to predict stable polymorphs .
Q. What strategies optimize TMAB’s use in catalytic applications while minimizing decomposition?
Properties
InChI |
InChI=1S/C4H12N.B/c1-5(2,3)4;/h1-4H3;/q+1;-1 | |
---|---|---|
Source | PubChem | |
URL | https://pubchem.ncbi.nlm.nih.gov | |
Description | Data deposited in or computed by PubChem | |
InChI Key |
TVQBZLPZXCVNEO-UHFFFAOYSA-N | |
Source | PubChem | |
URL | https://pubchem.ncbi.nlm.nih.gov | |
Description | Data deposited in or computed by PubChem | |
Canonical SMILES |
[B-].C[N+](C)(C)C | |
Source | PubChem | |
URL | https://pubchem.ncbi.nlm.nih.gov | |
Description | Data deposited in or computed by PubChem | |
Molecular Formula |
C4H12BN | |
Source | PubChem | |
URL | https://pubchem.ncbi.nlm.nih.gov | |
Description | Data deposited in or computed by PubChem | |
DSSTOX Substance ID |
DTXSID70937556 | |
Record name | N,N,N-Trimethylmethanaminium tetrahydroborate | |
Source | EPA DSSTox | |
URL | https://comptox.epa.gov/dashboard/DTXSID70937556 | |
Description | DSSTox provides a high quality public chemistry resource for supporting improved predictive toxicology. | |
Molecular Weight |
84.96 g/mol | |
Source | PubChem | |
URL | https://pubchem.ncbi.nlm.nih.gov | |
Description | Data deposited in or computed by PubChem | |
Physical Description |
White odorless crystalline powder; [Alfa Aesar MSDS] | |
Record name | Tetramethylammonium borohydride | |
Source | Haz-Map, Information on Hazardous Chemicals and Occupational Diseases | |
URL | https://haz-map.com/Agents/19853 | |
Description | Haz-Map® is an occupational health database designed for health and safety professionals and for consumers seeking information about the adverse effects of workplace exposures to chemical and biological agents. | |
Explanation | Copyright (c) 2022 Haz-Map(R). All rights reserved. Unless otherwise indicated, all materials from Haz-Map are copyrighted by Haz-Map(R). No part of these materials, either text or image may be used for any purpose other than for personal use. Therefore, reproduction, modification, storage in a retrieval system or retransmission, in any form or by any means, electronic, mechanical or otherwise, for reasons other than personal use, is strictly prohibited without prior written permission. | |
CAS No. |
16883-45-7 | |
Record name | Tetramethylammonium borohydrate | |
Source | ChemIDplus | |
URL | https://pubchem.ncbi.nlm.nih.gov/substance/?source=chemidplus&sourceid=0016883457 | |
Description | ChemIDplus is a free, web search system that provides access to the structure and nomenclature authority files used for the identification of chemical substances cited in National Library of Medicine (NLM) databases, including the TOXNET system. | |
Record name | Methanaminium, N,N,N-trimethyl-, tetrahydroborate(1-) (1:1) | |
Source | EPA Chemicals under the TSCA | |
URL | https://www.epa.gov/chemicals-under-tsca | |
Description | EPA Chemicals under the Toxic Substances Control Act (TSCA) collection contains information on chemicals and their regulations under TSCA, including non-confidential content from the TSCA Chemical Substance Inventory and Chemical Data Reporting. | |
Record name | N,N,N-Trimethylmethanaminium tetrahydroborate | |
Source | EPA DSSTox | |
URL | https://comptox.epa.gov/dashboard/DTXSID70937556 | |
Description | DSSTox provides a high quality public chemistry resource for supporting improved predictive toxicology. | |
Record name | Tetramethylammonium tetrahydroborate | |
Source | European Chemicals Agency (ECHA) | |
URL | https://echa.europa.eu/substance-information/-/substanceinfo/100.037.182 | |
Description | The European Chemicals Agency (ECHA) is an agency of the European Union which is the driving force among regulatory authorities in implementing the EU's groundbreaking chemicals legislation for the benefit of human health and the environment as well as for innovation and competitiveness. | |
Explanation | Use of the information, documents and data from the ECHA website is subject to the terms and conditions of this Legal Notice, and subject to other binding limitations provided for under applicable law, the information, documents and data made available on the ECHA website may be reproduced, distributed and/or used, totally or in part, for non-commercial purposes provided that ECHA is acknowledged as the source: "Source: European Chemicals Agency, http://echa.europa.eu/". Such acknowledgement must be included in each copy of the material. ECHA permits and encourages organisations and individuals to create links to the ECHA website under the following cumulative conditions: Links can only be made to webpages that provide a link to the Legal Notice page. | |
Retrosynthesis Analysis
AI-Powered Synthesis Planning: Our tool employs the Template_relevance Pistachio, Template_relevance Bkms_metabolic, Template_relevance Pistachio_ringbreaker, Template_relevance Reaxys, Template_relevance Reaxys_biocatalysis model, leveraging a vast database of chemical reactions to predict feasible synthetic routes.
One-Step Synthesis Focus: Specifically designed for one-step synthesis, it provides concise and direct routes for your target compounds, streamlining the synthesis process.
Accurate Predictions: Utilizing the extensive PISTACHIO, BKMS_METABOLIC, PISTACHIO_RINGBREAKER, REAXYS, REAXYS_BIOCATALYSIS database, our tool offers high-accuracy predictions, reflecting the latest in chemical research and data.
Strategy Settings
Precursor scoring | Relevance Heuristic |
---|---|
Min. plausibility | 0.01 |
Model | Template_relevance |
Template Set | Pistachio/Bkms_metabolic/Pistachio_ringbreaker/Reaxys/Reaxys_biocatalysis |
Top-N result to add to graph | 6 |
Feasible Synthetic Routes
Disclaimer and Information on In-Vitro Research Products
Please be aware that all articles and product information presented on BenchChem are intended solely for informational purposes. The products available for purchase on BenchChem are specifically designed for in-vitro studies, which are conducted outside of living organisms. In-vitro studies, derived from the Latin term "in glass," involve experiments performed in controlled laboratory settings using cells or tissues. It is important to note that these products are not categorized as medicines or drugs, and they have not received approval from the FDA for the prevention, treatment, or cure of any medical condition, ailment, or disease. We must emphasize that any form of bodily introduction of these products into humans or animals is strictly prohibited by law. It is essential to adhere to these guidelines to ensure compliance with legal and ethical standards in research and experimentation.