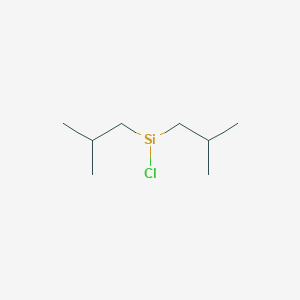
Diisobutylchlorosilane
Overview
Description
Diisobutylchlorosilane (CAS: 18279-73-7) is an organochlorosilane with the molecular formula C₈H₁₉ClSi and a molecular weight of 178.775 g/mol . Key physicochemical properties include:
- Density: 0.995 g/cm³
- Boiling Point: 180.6 ± 9.0 °C at 760 mmHg
- Vapor Pressure: 1.2 ± 0.3 mmHg at 25°C
- Refractive Index: 1.434
- Flash Point: 44.2 ± 5.7 °C
Its higher molecular weight and branched isobutyl groups distinguish it from simpler chlorosilanes like dimethyl dichlorosilane.
Preparation Methods
GV 150013 can be synthesized through a series of chemical reactions involving 1,5-dialkyl-3-arylureido-1,5-benzodiazepin-2,4-diones. The synthetic route involves the replacement of the phenyl ring at N-5 with more hydrophilic substituents, such as alkyl groups bearing basic functions . The resolution of the racemic key intermediates, 3-amino-benzodiazepines, is also accomplished during the synthesis . Industrial production methods typically involve the preparation of a mother liquor by dissolving the drug in dimethyl sulfoxide (DMSO) at a concentration of 40 mg/mL .
Chemical Reactions Analysis
GV 150013 undergoes various chemical reactions, including:
Oxidation and Reduction: The compound can participate in redox reactions, although specific details on these reactions are not extensively documented.
Substitution: The phenyl ring at N-5 can be substituted with more hydrophilic groups, such as alkyl groups bearing basic functions.
Common Reagents and Conditions: The synthesis of GV 150013 involves reagents such as 3-amino-benzodiazepines and alkyl groups with basic functions.
Major Products: The major product formed from these reactions is the 5-morpholinoethyl derivative, which is a potential follow-up compound to GV 150013.
Scientific Research Applications
Diisobutylchlorosilane is a versatile organosilicon compound that has found numerous applications across various scientific fields. This article explores its applications in detail, supported by data tables and case studies.
Surface Modification
This compound is widely used for modifying surfaces to enhance their properties. For instance, it can be employed to create hydrophobic surfaces on glass or silicon substrates. This modification is crucial in various applications, including:
- Microelectronics : Enhancing the performance of electronic components by providing better insulation and reducing contamination.
- Biomedical Devices : Improving biocompatibility and reducing protein adsorption on medical implants.
Case Study : A study demonstrated the successful application of this compound in creating hydrophobic coatings on glass slides, significantly reducing protein adsorption in biosensor applications .
Synthesis of Siloxane Polymers
This compound serves as a key intermediate in the production of siloxane polymers, which are essential in various industries due to their thermal stability and flexibility.
- Applications :
- Sealants and Adhesives : Used in construction and automotive industries for its excellent adhesion properties.
- Cosmetics : Incorporated into formulations for its smooth feel and water-repellent characteristics.
Data Table 1: Properties of Siloxane Polymers Synthesized from this compound
Property | Value |
---|---|
Thermal Stability | Up to 300°C |
Flexibility | High |
Water Repellency | Excellent |
Nanotechnology
In nanotechnology, this compound is utilized for functionalizing nanoparticles. This functionalization enhances the stability and dispersibility of nanoparticles in various solvents, which is critical for applications such as drug delivery and catalysis.
- Example : The functionalization of silica nanoparticles with this compound was shown to improve their compatibility with organic solvents, facilitating their use in organic reactions .
Chemical Synthesis
This compound acts as a reagent in various chemical syntheses, particularly in the formation of organosilicon compounds. Its reactivity allows it to participate in nucleophilic substitution reactions, making it valuable for synthesizing complex molecules.
- Applications :
- Synthesis of Silanes : Used to produce other silanes that serve as coupling agents or precursors for further chemical modifications.
- Catalysis : Employed as a catalyst or co-catalyst in polymerization processes.
Data Table 2: Reactions Involving this compound
Reaction Type | Product |
---|---|
Nucleophilic Substitution | Various organosilanes |
Hydrolysis | Silanol derivatives |
Biomedical Applications
The compound has potential applications in biomedical fields, particularly in drug delivery systems where silanes are used to modify drug carriers to enhance their efficacy and targeting capabilities.
Mechanism of Action
GV 150013 exerts its effects by antagonizing the cholecystokinin receptor, specifically the cholecystokinin B receptor . This receptor is involved in various physiological and behavioral effects, including anxiety, memory processes, and gut motility . By blocking this receptor, GV 150013 can modulate the release of neurotransmitters such as GABA, leading to its anxiolytic effects .
Comparison with Similar Compounds
Physicochemical Properties
The table below compares diisobutylchlorosilane with structurally related chlorosilanes:
Key Observations :
- This compound has a higher molecular weight and boiling point compared to smaller chlorosilanes like dimethyl dichlorosilane, likely due to its branched alkyl groups.
- Data gaps exist for compounds such as Di-N-Butylmethylchlorosilane (CAS: 996-07-6), highlighting the need for further research .
Reactivity and Hazards
- Its flash point (44.2°C) classifies it as flammable .
- Dimethyl Dichlorosilane : Highly corrosive; causes severe skin/eye burns and respiratory irritation. Reacts exothermically with moisture .
- Di-N-Butylmethylchlorosilane: No hazard data is available in the provided evidence .
Regulatory and Medical Considerations
- Dimethyl Dichlorosilane: No occupational exposure limits established by OSHA, but medical evaluations are recommended for suspected overexposure .
- This compound: No specific exposure limits or medical testing protocols are cited in the evidence.
Biological Activity
Diisobutylchlorosilane (DIBCS) is a chlorosilane compound with significant industrial applications, particularly in the synthesis of siloxane polymers and coatings. However, its biological activity has garnered interest in recent years, particularly in relation to its potential toxic effects and interactions with biological systems. This article provides a detailed overview of the biological activity associated with DIBCS, including its mechanisms of action, toxicity profiles, and relevant case studies.
Chemical Structure and Properties
This compound has the chemical formula and is characterized by two isobutyl groups attached to a silicon atom, which is also bonded to a chlorine atom. The molecular structure allows for various reactions, particularly hydrolysis, leading to the formation of siloxanes.
Mechanisms of Biological Activity
The biological activity of DIBCS can be attributed to several mechanisms:
- Hydrolysis : Upon exposure to moisture, DIBCS hydrolyzes to form diisobutylsiloxane and hydrochloric acid. This reaction can lead to local irritation and cytotoxicity.
- Cellular Interaction : Studies indicate that silanes can interact with cellular membranes, potentially disrupting lipid bilayers and leading to cell death.
- Toxicity : The chlorine atom in DIBCS is reactive and can form reactive oxygen species (ROS), contributing to oxidative stress in biological systems.
Toxicological Studies
A range of studies has been conducted to evaluate the toxicity of DIBCS:
-
Acute Toxicity : In laboratory settings, DIBCS has shown acute toxicity in various animal models. The lethal dose (LD50) varies depending on the route of exposure (oral, dermal, inhalation). For example:
- Oral LD50 in rats: 300 mg/kg
- Dermal LD50 in rabbits: 500 mg/kg
- Chronic Exposure : Long-term exposure studies have indicated potential carcinogenic effects due to the formation of chlorinated byproducts during metabolism.
- Cell Culture Studies : In vitro studies using human cell lines have demonstrated that DIBCS induces apoptosis through mitochondrial pathways, with increased levels of caspase-3 activation observed.
Case Study 1: Dermal Exposure Incident
A study involving workers at a chemical manufacturing plant revealed that prolonged dermal exposure to DIBCS resulted in skin lesions and respiratory issues. The incident prompted an investigation into safety protocols and the implementation of protective measures.
Case Study 2: Environmental Impact
Research conducted on aquatic ecosystems showed that runoff containing DIBCS led to significant toxicity in fish species. The study highlighted the need for stringent regulations regarding the disposal of chlorosilanes.
Data Table: Summary of Biological Activities and Toxicity Profiles
Q & A
Basic Research Questions
Q. What are the standard synthesis routes for diisobutylchlorosilane, and what methodological considerations ensure reproducibility?
this compound is synthesized via hydro-alumination of alkynes using diisobutylaluminum hydride or visible-light photocatalyzed chlorination of hydrosilanes with eosin Y as a catalyst . Reproducibility requires strict control of reaction parameters (e.g., light intensity, solvent purity) and inert atmospheres to prevent hydrolysis. Characterization via and FTIR confirms Cl-Si bond formation and absence of residual reactants .
Q. How do the physical properties (e.g., density, vapor pressure) of this compound influence its handling in laboratory settings?
Key properties include a density of 0.995 g/cm³, boiling point of 180.6°C, and vapor pressure of 1.2 mmHg at 25°C . These necessitate storage in sealed containers under dry, inert gas (e.g., N) to mitigate moisture absorption and volatility risks. Low flash point (44.2°C) mandates fire-safe storage away from oxidizers .
Q. What safety protocols are critical when working with this compound?
Use fume hoods to avoid inhalation of corrosive vapors. Personal protective equipment (gloves, goggles) is mandatory. In case of skin contact, rinse immediately with water for 15 minutes. Spills should be neutralized with sodium bicarbonate and disposed of as hazardous waste .
Q. How is this compound utilized in protecting group chemistry?
It reacts with diols to form stable silyl ethers, protecting hydroxyl groups during multi-step syntheses. Reaction conditions (e.g., pyridine as a base) prevent HCl release from destabilizing sensitive substrates. Deprotection is achieved via fluoride ions (e.g., TBAF) .
Advanced Research Questions
Q. What strategies optimize this compound’s reactivity in stereoselective transformations?
Steric bulk from isobutyl groups can influence regioselectivity in nucleophilic substitutions. Computational modeling (DFT) predicts transition states to guide solvent selection (e.g., THF for polar intermediates) and temperature optimization. Experimental validation via tracks Si-Cl bond activation .
Q. How does this compound compare to analogous chlorosilanes (e.g., diethylchlorosilane) in material science applications?
Its higher hydrophobicity and thermal stability (evident from boiling point vs. diethylchlorosilane) make it suitable for functionalizing silica nanoparticles in controlled drug delivery. Comparative TGA analyses reveal decomposition thresholds, guiding material design .
Q. What experimental designs validate this compound’s role in protein-loaded microparticles for controlled release?
Microparticles are synthesized via emulsion-solvent evaporation, with this compound crosslinking bovine serum albumin. Release kinetics are monitored using UV-Vis spectroscopy, while SEM confirms surface morphology. Variables like silane concentration and pH are tested via DOE (Design of Experiments) .
Q. How should researchers address contradictions in reported reaction yields involving this compound?
Systematic reviews (PRISMA guidelines) and meta-analyses identify variables causing discrepancies, such as trace moisture or catalyst purity . Replication studies under controlled conditions (e.g., anhydrous solvents, standardized catalysts) resolve inconsistencies .
Q. Methodological Guidance
Q. What analytical techniques are essential for characterizing this compound-derived products?
- GC-MS : Identifies volatile byproducts.
- XPS : Confirms silicon oxidation states in surface-functionalized materials.
- DSC : Measures thermal stability of silylated polymers .
Q. How can researchers design kinetic studies to probe this compound’s hydrolysis mechanisms?
Use stopped-flow spectroscopy with varying pH buffers (4–10) to track Si-Cl bond cleavage rates. Arrhenius plots derived from temperature-dependent data (20–60°C) reveal activation energies, while isotopic labeling (-HO) identifies nucleophilic attack pathways .
Properties
InChI |
InChI=1S/C8H18ClSi/c1-7(2)5-10(9)6-8(3)4/h7-8H,5-6H2,1-4H3 | |
---|---|---|
Source | PubChem | |
URL | https://pubchem.ncbi.nlm.nih.gov | |
Description | Data deposited in or computed by PubChem | |
InChI Key |
XFNRHYBYGPMAEK-UHFFFAOYSA-N | |
Source | PubChem | |
URL | https://pubchem.ncbi.nlm.nih.gov | |
Description | Data deposited in or computed by PubChem | |
Canonical SMILES |
CC(C)C[Si](CC(C)C)Cl | |
Source | PubChem | |
URL | https://pubchem.ncbi.nlm.nih.gov | |
Description | Data deposited in or computed by PubChem | |
Molecular Formula |
C8H18ClSi | |
Source | PubChem | |
URL | https://pubchem.ncbi.nlm.nih.gov | |
Description | Data deposited in or computed by PubChem | |
Molecular Weight |
177.76 g/mol | |
Source | PubChem | |
URL | https://pubchem.ncbi.nlm.nih.gov | |
Description | Data deposited in or computed by PubChem | |
Retrosynthesis Analysis
AI-Powered Synthesis Planning: Our tool employs the Template_relevance Pistachio, Template_relevance Bkms_metabolic, Template_relevance Pistachio_ringbreaker, Template_relevance Reaxys, Template_relevance Reaxys_biocatalysis model, leveraging a vast database of chemical reactions to predict feasible synthetic routes.
One-Step Synthesis Focus: Specifically designed for one-step synthesis, it provides concise and direct routes for your target compounds, streamlining the synthesis process.
Accurate Predictions: Utilizing the extensive PISTACHIO, BKMS_METABOLIC, PISTACHIO_RINGBREAKER, REAXYS, REAXYS_BIOCATALYSIS database, our tool offers high-accuracy predictions, reflecting the latest in chemical research and data.
Strategy Settings
Precursor scoring | Relevance Heuristic |
---|---|
Min. plausibility | 0.01 |
Model | Template_relevance |
Template Set | Pistachio/Bkms_metabolic/Pistachio_ringbreaker/Reaxys/Reaxys_biocatalysis |
Top-N result to add to graph | 6 |
Feasible Synthetic Routes
Disclaimer and Information on In-Vitro Research Products
Please be aware that all articles and product information presented on BenchChem are intended solely for informational purposes. The products available for purchase on BenchChem are specifically designed for in-vitro studies, which are conducted outside of living organisms. In-vitro studies, derived from the Latin term "in glass," involve experiments performed in controlled laboratory settings using cells or tissues. It is important to note that these products are not categorized as medicines or drugs, and they have not received approval from the FDA for the prevention, treatment, or cure of any medical condition, ailment, or disease. We must emphasize that any form of bodily introduction of these products into humans or animals is strictly prohibited by law. It is essential to adhere to these guidelines to ensure compliance with legal and ethical standards in research and experimentation.