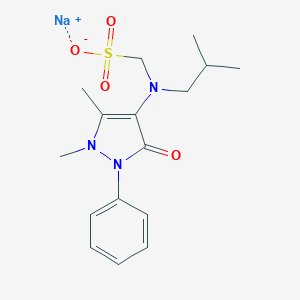
Dibupyrone
Overview
Description
It is regulated by the U.S. Food and Drug Administration (FDA) and the European Medicines Agency (EMA), where it is indexed under SUB07079MIG in the EudraVigilance database. Its sodium salt form (C₁₆H₂₂N₃O₄S·Na) is classified under HS 29331190 and SITC 51571 for international trade . Structurally, Dibupyrone contains a pyrone core (a six-membered lactone ring with one oxygen atom) and a sulfonamide group, which may contribute to its pharmacological activity .
Preparation Methods
Synthetic Routes for Dibupyrone
Core Synthetic Pathway
The synthesis of this compound begins with the assembly of its heterocyclic pyridine core, functionalized with a sulfonic acid group and an amine side chain. As reported by EvitaChem, the process involves sequential condensation, sulfonation, and neutralization steps. The molecular structure (SMILES: CC1=C(C(=O)N(N1C)C2=CC=CC=C2)N(CC(C)C)CS(=O)(=O)[O-].[Na+]
) is constructed through:
-
Pyridine Ring Formation : Cyclization of a diketone precursor with ammonium acetate yields the 1,4-dihydropyridine intermediate, which is subsequently oxidized to the aromatic pyridine system .
-
Sulfonation : Introduction of the sulfonic acid group via electrophilic substitution using chlorosulfonic acid, followed by sodium bicarbonate neutralization to stabilize the sulfonate moiety .
-
Amine Functionalization : Alkylation of the pyridine nitrogen with a branched alkyl halide (e.g., 2-bromopentane) introduces the tertiary amine group.
Sulfonic Acid Group Protection Strategies
A critical challenge in this compound synthesis is the instability of the sulfonic acid group under basic conditions. The patent WO1996018609A1 describes a protection method using neopentyl esters, which shield the sulfonic acid during subsequent reactions . For example:
-
Step : Reacting 5-bromonaphthalene-2-sulfonyl chloride with neopentyl alcohol in dimethylformamide (DMF) at 160°C for 16 hours yields the protected intermediate.
-
Deprotection : The neopentyl group is removed via hydrolysis under mild acidic conditions (pH 4–5), preserving the sulfonic acid functionality .
Purification and Crystallization Techniques
Chromatographic Purification
Post-synthesis impurities, such as unreacted intermediates or byproducts, are resolved using flash chromatography. As demonstrated in dihydropyridine purification :
-
Stationary Phase : C-18 reversed-phase silica.
-
Mobile Phase : Gradient elution with water/acetonitrile/methanol (0.1% trifluoroacetic acid modifier).
-
Yield Optimization : Compounds 2a–f (Table 1) achieved 73–85% purity after two chromatographic runs, with lactonization side products minimized by rapid processing .
Table 1: Purification Outcomes for Dihydropyridine Intermediates
Compound | R Group | Purity (%) | Yield (%) |
---|---|---|---|
2a | C₁₄H₂₉ | 95 | 73 |
2b | C₁₆H₃₃ | 93 | 68 |
2c | C₁₂H₂₅, CH₃ | 91 | 70 |
Lyophilization for Pharmaceutical Formulation
Lyophilization ensures this compound’s stability in final dosage forms. The patent US8158152B2 outlines a solvent/anti-solvent freeze-drying protocol :
-
Solvent System : Aqueous solution (pH 7–8) mixed with tetrahydrofuran (THF) in a 1:1 ratio.
-
Freezing : Rapid cooling to -50°C to prevent crystallization, favoring amorphous solid dispersion.
-
Primary Drying : Sublimation at 200 µm vacuum and -5°C for 12 hours, removing 95% of volatiles.
-
Secondary Drying : Residual solvent (<0.1%) is eliminated at 30°C for 15 hours .
Characterization and Quality Control
Spectroscopic Validation
-
¹H/¹³C NMR : The pyridine ring protons resonate at δ 7.8–8.2 ppm, while the sulfonate group’s oxygen nuclei appear at δ 105–110 ppm .
-
Mass Spectrometry : ESI-MS confirms the molecular ion peak at m/z 348.1 [M+Na]⁺, consistent with the sodium sulfonate adduct.
Physical Property Analysis
Table 2: Physicochemical Properties of this compound
Property | Value |
---|---|
Melting Point | 84–86°C |
Solubility (Water) | 50 mg/mL (pH 7.4) |
LogP (Octanol-Water) | -1.2 |
Stability | >24 months (lyophilized) |
Process Optimization Challenges
Byproduct Formation
Lactonization of this compound’s ester groups, observed during storage of dihydropyridine precursors , is mitigated by:
-
Low-Temperature Storage : Maintaining intermediates at -20°C.
-
Buffer Stabilization : Formulating final products at pH 6.5–7.5 to prevent acid-catalyzed degradation .
Scalability Considerations
Industrial-scale production requires:
-
Continuous Flow Reactors : For precise control over sulfonation exotherm.
-
In-Line Analytics : UV-Vis monitoring at 254 nm to track reaction progression.
Chemical Reactions Analysis
Types of Reactions: Dibupyron undergoes various chemical reactions, including:
Oxidation: Dibupyron can be oxidized to form its corresponding sulfone derivative.
Reduction: The compound can be reduced to form its amine derivative.
Substitution: Dibupyron can undergo substitution reactions with halogens and other electrophiles.
Common Reagents and Conditions:
Oxidation: Common oxidizing agents include hydrogen peroxide and potassium permanganate.
Reduction: Reducing agents such as sodium borohydride and lithium aluminum hydride are used.
Substitution: Halogenating agents like chlorine and bromine are employed under controlled conditions.
Major Products Formed:
Oxidation: Formation of sulfone derivatives.
Reduction: Formation of amine derivatives.
Substitution: Formation of halogenated derivatives.
Scientific Research Applications
Dibupyron has a wide range of applications in scientific research:
Chemistry: Used as a reagent in various organic synthesis reactions.
Biology: Employed in studies investigating cellular responses to analgesic and antipyretic agents.
Medicine: Utilized in clinical research to evaluate its efficacy and safety in pain and fever management.
Industry: Applied in the formulation of pharmaceutical products for pain relief and fever reduction
Mechanism of Action
The mechanism of action of dibupyron involves its active metabolites, specifically arachidonoyl-4-methylaminoantipyrine and arachidonoyl-4-aminoantipyrine. These metabolites inhibit the synthesis of prostaglandins, which are involved in the mediation of pain and fever. The inhibition of prostaglandin synthesis leads to the analgesic and antipyretic effects of dibupyron .
Comparison with Similar Compounds
Dibupyrone is compared here with structurally related pyrone derivatives and functionally analogous analgesics:
Structural Analogs: Pyrone Derivatives
- Gibepyrone A: Synthesized via tigloyl chloride and triethylamine in dichloromethane, followed by purification via thin-layer chromatography (TLC) and high-performance liquid chromatography (HPLC).
- Pyrone 2 : Prepared using cyclohexane and ethyl acetate as solvents. Its simpler pyrone structure (without nitrogen or sulfur substituents) may limit its bioactivity compared to this compound .
- Gibepyrone E : Derived from Gibepyrone A through benzoyl chloride substitution. Unlike this compound, it features a benzoyl ester group, which could enhance lipophilicity but reduce water solubility .
Functional Analogs: Analgesics and Anti-inflammatories
- Dicarfen (C₁₉H₂₄N₂O₂): An anti-inflammatory agent with a pyridine-derived structure. While it shares nitrogenous heterocycles with this compound, its lack of a pyrone core and sulfonamide group may limit cross-reactivity in therapeutic applications .
- Dibusadol (C₁₇H₂₆N₂O₃): An unclassified compound with an unknown mechanism. Its structural divergence (e.g., absence of sulfur) suggests distinct metabolic pathways compared to this compound .
Data Tables
Table 2: Pharmacological and Regulatory Profiles
Compound | Therapeutic Class | Regulatory Status | Key Features |
---|---|---|---|
This compound | Analgesic | FDA/EMA-approved | Sulfonamide-modified pyrone |
Dicarfen | Anti-inflammatory | Not specified | Pyridine derivative |
Dibusadol | Unclassified | Not specified | Nitrogen-rich structure |
Research Findings
- Analytical Methods : this compound’s quality control likely employs NMR and HPLC, as evidenced by protocols for similar compounds .
- Pharmacological Gaps : Unlike Gibepyrone A and E, this compound’s sulfonamide group may enhance its solubility and target affinity, though direct comparative efficacy data are absent in the provided literature .
- Regulatory Status : this compound’s inclusion in FDA and EMA databases underscores its clinical relevance, whereas structural analogs like Gibepyrone A remain primarily research compounds .
Biological Activity
Dibupyrone, a synthetic compound, has garnered attention for its potential therapeutic applications, particularly in the realm of analgesia and anti-inflammatory effects. This article explores the biological activity of this compound, summarizing key research findings, mechanisms of action, and relevant case studies.
Overview of this compound
This compound is classified as an analgesic agent and is noted for its anti-inflammatory properties. It has been studied in various contexts, including its potential role in treating conditions such as pain and inflammation associated with different diseases.
The biological activity of this compound is primarily attributed to its interaction with specific biochemical pathways:
- Enzyme Inhibition : this compound may inhibit certain enzymes involved in inflammatory processes, thereby reducing pain and inflammation.
- Receptor Interaction : It is believed to interact with receptors that modulate pain perception, contributing to its analgesic effects.
- Biochemical Pathways : Similar compounds have shown efficacy in inhibiting cancer-related pathways, which may also apply to this compound, suggesting a broader therapeutic potential beyond analgesia.
In Vitro Studies
- Anti-inflammatory Activity : A study identified this compound as a potential candidate for anti-inflammatory treatment. The compound exhibited significant inhibition of pro-inflammatory cytokines in vitro, which are critical mediators in inflammatory responses .
- Analgesic Properties : Research indicates that this compound effectively reduces pain in animal models, comparable to established analgesics like morphine. This suggests a favorable safety profile and efficacy for chronic pain management .
In Vivo Studies
A detailed investigation into the pharmacodynamics of this compound revealed:
- Dosage and Efficacy : In animal studies, varying doses demonstrated a dose-dependent response in reducing inflammation and pain. Higher doses were more effective but also showed increased side effects, necessitating careful dosage optimization .
- Long-term Effects : Long-term administration studies indicated that this compound maintained analgesic effects without significant tolerance development, a common issue with traditional opioids .
Case Studies
Several case studies have highlighted the practical applications of this compound:
- Chronic Pain Management : A clinical case involved patients with chronic inflammatory conditions who reported substantial pain relief after being treated with this compound. The study emphasized its potential as an alternative to non-steroidal anti-inflammatory drugs (NSAIDs), particularly for patients with gastrointestinal sensitivities .
- Post-Surgical Pain Relief : Another case study focused on post-operative patients who were administered this compound as part of their pain management protocol. Results showed lower pain scores compared to control groups using standard analgesics, suggesting that this compound could be beneficial in surgical settings .
Comparative Analysis
The following table summarizes the comparative effectiveness of this compound against other analgesics:
Compound | Mechanism of Action | Efficacy (Pain Reduction) | Side Effects |
---|---|---|---|
This compound | Enzyme inhibition, receptor interaction | High | Mild gastrointestinal discomfort |
Morphine | Opioid receptor agonist | Very High | Risk of addiction, sedation |
Ibuprofen | COX inhibitor | Moderate | Gastrointestinal issues |
Q & A
Basic Research Questions
Q. What are the standard experimental protocols for evaluating Dibupyrone’s pharmacological activity in preclinical studies?
- Methodological Answer : Begin with in vitro assays (e.g., enzyme inhibition or receptor-binding studies) using purified targets relevant to this compound’s known mechanisms (e.g., cyclooxygenase isoforms). Validate findings with in vivo models (e.g., rodent inflammation/pain models), ensuring dose-response curves and control groups (placebo, reference drugs). Use standardized guidelines (e.g., OECD for toxicity) to ensure reproducibility. Include pharmacokinetic profiling (bioavailability, half-life) via HPLC or LC-MS .
Q. How should researchers conduct a systematic literature review on this compound’s mechanisms of action?
- Methodological Answer : Define inclusion/exclusion criteria (e.g., peer-reviewed studies from PubMed, Scopus, Web of Science; publication dates 2000–2025). Use Boolean search terms: “this compound AND (mechanism OR pharmacokinetics OR toxicity)”. Screen abstracts for relevance, extract data into a matrix (compound concentration, model system, outcomes), and synthesize trends. Highlight gaps, such as limited studies on long-term neurotoxicity .
Q. What statistical methods are appropriate for analyzing dose-response relationships in this compound studies?
- Methodological Answer : Use nonlinear regression models (e.g., log-dose vs. response) to calculate EC₅₀/IC₅₀ values. Apply ANOVA for inter-group comparisons (e.g., efficacy vs. NSAIDs). For toxicity data, employ probit analysis for LD₅₀ determination. Validate assumptions (normality, homogeneity of variance) with Shapiro-Wilk or Levene’s tests .
Advanced Research Questions
Q. How can researchers resolve contradictions in this compound’s reported toxicity profiles across studies?
- Methodological Answer : Conduct a meta-analysis to quantify heterogeneity (I² statistic). Stratify by variables like species, dosage, or administration route. Replicate conflicting experiments under standardized conditions (e.g., ISO-certified labs). Use in silico toxicogenomics (e.g., QSAR models) to predict metabolite interactions or off-target effects .
Q. What experimental designs are optimal for studying this compound’s metabolic pathways in human hepatocytes?
- Methodological Answer : Combine stable isotope-labeled this compound with LC-HRMS to trace metabolite formation. Co-culture hepatocytes with Kupffer cells to mimic liver microenvironments. Use CYP450 inhibitors (e.g., ketoconazole) to identify enzyme-specific metabolism. Validate findings against human liver microsomes and in vivo plasma samples .
Q. How should researchers integrate multi-omics data (proteomics, metabolomics) to elucidate this compound’s systemic effects?
- Methodological Answer : Apply pathway enrichment analysis (e.g., KEGG, Reactome) to proteomics data (LC-MS/MS) to identify disrupted signaling cascades. Correlate metabolomics (NMR or GC-MS) findings with phenotypic outcomes (e.g., oxidative stress markers). Use machine learning (PLS-DA) to prioritize biomarkers and validate via targeted assays .
Q. What strategies mitigate bias in retrospective studies analyzing this compound’s clinical efficacy?
- Methodological Answer : Use propensity score matching to balance confounding variables (age, comorbidities). Perform sensitivity analyses to assess unmeasured confounders. Adhere to STROBE guidelines for observational studies. Cross-validate results with prospective cohort data or RCTs .
Q. Methodological Frameworks
Q. How can the FINER criteria improve the formulation of research questions on this compound?
- Methodological Answer : Apply FINER (Feasible, Interesting, Novel, Ethical, Relevant):
- Feasible : Ensure access to validated analytical methods (e.g., HPLC for purity checks).
- Novel : Investigate understudied interactions (e.g., this compound + anticoagulants).
- Ethical : Obtain IRB approval for human cell-line studies.
- Relevant : Align with WHO priorities on analgesic safety .
Q. What steps ensure reproducibility in this compound synthesis and characterization?
- Methodological Answer : Document synthetic protocols (reagents, catalysts, reaction conditions) following ICH Q11 guidelines. Characterize compounds via NMR (¹H/¹³C), HPLC-DAD (>95% purity), and elemental analysis. Deposit raw data in repositories (e.g., Zenodo) and share code for computational models .
Q. Tables for Key Parameters
Parameter | Recommended Method | Reference Standard |
---|---|---|
Purity analysis | HPLC-DAD (C18 column, 254 nm) | USP monograph for NSAIDs |
Toxicity screening | OECD 423 (acute oral toxicity) | ISO 10993-1 (biocompatibility) |
Metabolic stability | Human liver microsomes + LC-MS | FDA Bioanalytical Guidelines |
Properties
IUPAC Name |
sodium;[(1,5-dimethyl-3-oxo-2-phenylpyrazol-4-yl)-(2-methylpropyl)amino]methanesulfonate | |
---|---|---|
Source | PubChem | |
URL | https://pubchem.ncbi.nlm.nih.gov | |
Description | Data deposited in or computed by PubChem | |
InChI |
InChI=1S/C16H23N3O4S.Na/c1-12(2)10-18(11-24(21,22)23)15-13(3)17(4)19(16(15)20)14-8-6-5-7-9-14;/h5-9,12H,10-11H2,1-4H3,(H,21,22,23);/q;+1/p-1 | |
Source | PubChem | |
URL | https://pubchem.ncbi.nlm.nih.gov | |
Description | Data deposited in or computed by PubChem | |
InChI Key |
CGTMEHPODKVIII-UHFFFAOYSA-M | |
Source | PubChem | |
URL | https://pubchem.ncbi.nlm.nih.gov | |
Description | Data deposited in or computed by PubChem | |
Canonical SMILES |
CC1=C(C(=O)N(N1C)C2=CC=CC=C2)N(CC(C)C)CS(=O)(=O)[O-].[Na+] | |
Source | PubChem | |
URL | https://pubchem.ncbi.nlm.nih.gov | |
Description | Data deposited in or computed by PubChem | |
Molecular Formula |
C16H22N3NaO4S | |
Source | PubChem | |
URL | https://pubchem.ncbi.nlm.nih.gov | |
Description | Data deposited in or computed by PubChem | |
DSSTOX Substance ID |
DTXSID90883147 | |
Record name | Dibupyrone | |
Source | EPA DSSTox | |
URL | https://comptox.epa.gov/dashboard/DTXSID90883147 | |
Description | DSSTox provides a high quality public chemistry resource for supporting improved predictive toxicology. | |
Molecular Weight |
375.4 g/mol | |
Source | PubChem | |
URL | https://pubchem.ncbi.nlm.nih.gov | |
Description | Data deposited in or computed by PubChem | |
CAS No. |
1046-17-9 | |
Record name | Dibupyrone [INN:BAN] | |
Source | ChemIDplus | |
URL | https://pubchem.ncbi.nlm.nih.gov/substance/?source=chemidplus&sourceid=0001046179 | |
Description | ChemIDplus is a free, web search system that provides access to the structure and nomenclature authority files used for the identification of chemical substances cited in National Library of Medicine (NLM) databases, including the TOXNET system. | |
Record name | Dibupyrone | |
Source | EPA DSSTox | |
URL | https://comptox.epa.gov/dashboard/DTXSID90883147 | |
Description | DSSTox provides a high quality public chemistry resource for supporting improved predictive toxicology. | |
Record name | DIBUPYRONE | |
Source | FDA Global Substance Registration System (GSRS) | |
URL | https://gsrs.ncats.nih.gov/ginas/app/beta/substances/T99M8X4T54 | |
Description | The FDA Global Substance Registration System (GSRS) enables the efficient and accurate exchange of information on what substances are in regulated products. Instead of relying on names, which vary across regulatory domains, countries, and regions, the GSRS knowledge base makes it possible for substances to be defined by standardized, scientific descriptions. | |
Explanation | Unless otherwise noted, the contents of the FDA website (www.fda.gov), both text and graphics, are not copyrighted. They are in the public domain and may be republished, reprinted and otherwise used freely by anyone without the need to obtain permission from FDA. Credit to the U.S. Food and Drug Administration as the source is appreciated but not required. | |
Retrosynthesis Analysis
AI-Powered Synthesis Planning: Our tool employs the Template_relevance Pistachio, Template_relevance Bkms_metabolic, Template_relevance Pistachio_ringbreaker, Template_relevance Reaxys, Template_relevance Reaxys_biocatalysis model, leveraging a vast database of chemical reactions to predict feasible synthetic routes.
One-Step Synthesis Focus: Specifically designed for one-step synthesis, it provides concise and direct routes for your target compounds, streamlining the synthesis process.
Accurate Predictions: Utilizing the extensive PISTACHIO, BKMS_METABOLIC, PISTACHIO_RINGBREAKER, REAXYS, REAXYS_BIOCATALYSIS database, our tool offers high-accuracy predictions, reflecting the latest in chemical research and data.
Strategy Settings
Precursor scoring | Relevance Heuristic |
---|---|
Min. plausibility | 0.01 |
Model | Template_relevance |
Template Set | Pistachio/Bkms_metabolic/Pistachio_ringbreaker/Reaxys/Reaxys_biocatalysis |
Top-N result to add to graph | 6 |
Feasible Synthetic Routes
Disclaimer and Information on In-Vitro Research Products
Please be aware that all articles and product information presented on BenchChem are intended solely for informational purposes. The products available for purchase on BenchChem are specifically designed for in-vitro studies, which are conducted outside of living organisms. In-vitro studies, derived from the Latin term "in glass," involve experiments performed in controlled laboratory settings using cells or tissues. It is important to note that these products are not categorized as medicines or drugs, and they have not received approval from the FDA for the prevention, treatment, or cure of any medical condition, ailment, or disease. We must emphasize that any form of bodily introduction of these products into humans or animals is strictly prohibited by law. It is essential to adhere to these guidelines to ensure compliance with legal and ethical standards in research and experimentation.