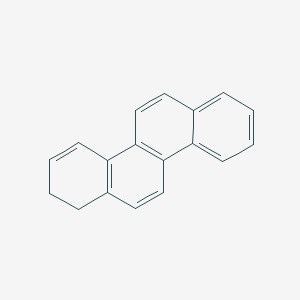
1,2-Dihydrochrysene
- Click on QUICK INQUIRY to receive a quote from our team of experts.
- With the quality product at a COMPETITIVE price, you can focus more on your research.
Overview
Description
1,2-Dihydrochrysene is a partially saturated derivative of chrysene, a polycyclic aromatic hydrocarbon (PAH) consisting of four fused benzene rings. The reduction of one double bond in chrysene results in the formation of this compound, which serves as a critical metabolic precursor in the bioactivation of chrysene into highly reactive intermediates, such as bay-region diol epoxides . These intermediates are implicated in the compound's mutagenic and carcinogenic properties. Structurally, this compound retains the angular "bay-region" configuration (a sterically hindered area between C1 and C12 in chrysene), which is pivotal for its biological activity .
Scientific Research Applications
1,2-Dihydrochrysene is a polycyclic aromatic hydrocarbon that has garnered attention in various scientific research applications due to its unique chemical properties. This article explores its applications across several fields, including materials science, environmental science, and biochemistry, while providing comprehensive data tables and case studies to illustrate its significance.
Materials Science
- Polymer Development : this compound is used as a building block in the synthesis of advanced polymers. These polymers exhibit enhanced thermal stability and mechanical strength, making them suitable for applications in aerospace and automotive industries.
- Nanocomposites : The incorporation of this compound into nanocomposite materials has been studied for improving electrical conductivity and thermal properties. Research indicates that these composites can be utilized in electronic devices and energy storage systems.
Environmental Science
- Pollutant Degradation : Studies have shown that this compound can be biotransformed by certain microorganisms, which can potentially be harnessed for bioremediation efforts in petroleum-contaminated environments . This application is particularly relevant given the increasing concern over environmental pollution.
- Toxicological Studies : Research on the toxicological effects of this compound has revealed insights into its carcinogenic potential when exposed to biological systems. Understanding these effects is crucial for assessing environmental risks associated with polycyclic aromatic hydrocarbons.
Biochemical Applications
- Drug Development : The compound's structural properties have led to investigations into its potential as a precursor in the synthesis of pharmaceuticals. Its derivatives may exhibit biological activity that could be beneficial in medicinal chemistry.
- Analytical Chemistry : this compound serves as a standard reference material in chromatographic analyses, aiding in the detection and quantification of polycyclic aromatic hydrocarbons in various samples.
Table 1: Summary of Applications
Application Area | Specific Use Case | Key Findings |
---|---|---|
Materials Science | Polymer development | Enhanced thermal stability |
Nanocomposites | Improved electrical conductivity | |
Environmental Science | Pollutant degradation | Effective biotransformation by fungi |
Toxicological studies | Insights into carcinogenic potential | |
Biochemical Applications | Drug development | Potential precursor for pharmaceuticals |
Analytical chemistry | Standard reference material |
Case Study 1: Bioremediation of Petroleum-Contaminated Soils
In a study focused on the degradation of chrysene derivatives, researchers isolated filamentous fungi from petroleum-contaminated soils that effectively oxidized chrysene to trans-1,2-dihydroxy-1,2-dihydrochrysene . This biotransformation illustrates the potential of using this compound in environmental cleanup strategies.
Case Study 2: Polymer Synthesis
A recent investigation into the synthesis of high-performance polymers incorporating this compound demonstrated significant improvements in mechanical properties compared to traditional polymer matrices. The resulting materials showed promise for use in demanding applications such as aerospace components.
Q & A
Basic Research Questions
Q. What are the established synthetic routes for 1,2-Dihydrochrysene, and how can their efficiency be optimized experimentally?
- Methodology : Focus on catalytic hydrogenation of chrysene derivatives, leveraging transition metal catalysts (e.g., Pd/C or PtO₂) under controlled hydrogen pressure. Optimization can involve Design of Experiments (DoE) to assess variables like temperature, solvent polarity, and catalyst loading . Purity validation requires HPLC or GC-MS coupled with melting point analysis .
- Data Analysis : Track reaction yields and byproduct formation via chromatographic techniques. Compare thermodynamic feasibility using computational tools (e.g., Gaussian for ΔG calculations) .
Q. How can spectroscopic techniques (NMR, IR, MS) confirm the structural integrity of this compound?
- Methodology : Use ¹H/¹³C NMR to identify proton environments and aromaticity reduction. IR spectra should show loss of C=C stretching vibrations (1600–1680 cm⁻¹). High-resolution MS confirms molecular ion peaks (e.g., [M+H]⁺) .
- Critical Evaluation : Cross-validate spectral data with computational predictions (e.g., ChemDraw or ACD/Labs). Address discrepancies by assessing solvent effects or impurities .
Advanced Research Questions
Q. How can researchers resolve contradictions in reported thermodynamic properties (e.g., ΔHf, solubility) of this compound?
- Methodology : Conduct differential scanning calorimetry (DSC) to measure enthalpy of formation. Compare results with computational models (e.g., COSMO-RS for solubility). Replicate conflicting studies under standardized conditions (e.g., IUPAC-recommended protocols) .
- Data Synthesis : Perform meta-analysis of published data, evaluating biases in measurement techniques (e.g., static vs. dynamic solubility methods). Use statistical tools like ANOVA to identify outliers .
Q. What computational models best predict the regioselectivity of this compound in electrophilic substitution reactions?
- Methodology : Apply Density Functional Theory (DFT) to map electron density surfaces and Fukui indices. Validate predictions with experimental kinetic studies (e.g., nitration or halogenation under controlled conditions) .
- Advanced Analysis : Compare DFT results with Molecular Dynamics (MD) simulations to account for solvent effects. Use symmetry-adapted perturbation theory (SAPT) to quantify intermolecular interactions .
Q. How to design a heterogenous catalytic system for selective oxidation of this compound to chrysene derivatives?
- Experimental Design : Screen metal-oxide catalysts (e.g., V₂O₅/TiO₂) under flow reactor conditions. Monitor kinetics via in-situ FTIR or Raman spectroscopy. Optimize turnover frequency (TOF) by adjusting O₂ partial pressure and catalyst pore size .
- Mechanistic Insights : Use X-ray photoelectron spectroscopy (XPS) to track catalyst oxidation states. Propose a Mars-van Krevelen mechanism if lattice oxygen participation is observed .
Q. Methodological Frameworks
- Systematic Reviews : Follow PRISMA guidelines to synthesize literature on synthetic pathways or catalytic mechanisms. Use tools like RevMan for meta-analysis of thermodynamic data .
- Ethical & Reproducibility Standards : Document synthetic protocols in line with ACS Ethical Guidelines. Share raw spectral data and computational input files via repositories like Zenodo .
- Research Question Formulation : Apply FINER criteria (Feasible, Interesting, Novel, Ethical, Relevant) to avoid trivial inquiries. For example, "How does steric hindrance in this compound influence its photochemical stability compared to chrysene?" .
Comparison with Similar Compounds
Comparison with Structurally Similar Compounds
Structural Analogs of Chrysene Derivatives
Key structural analogs include:
- 3,4-Dihydrochrysene : Reduction at the C3-C4 position.
- trans-1,2-Dihydroxy-1,2-dihydrochrysene : A dihydrodiol metabolite.
- Bay-region diol epoxides : e.g., (±)-1β,2α-dihydroxy-3α,4α-epoxy-1,2,3,4-tetrahydrochrysene (diol epoxide-2) and its diastereomer diol epoxide-1.
- Non-bay-region derivatives: e.g., 5,6-epoxychrysene (K-region epoxide).
Key Findings
Position of Saturation: 1,2-Dihydrochrysene exhibits significantly higher tumorigenicity (73–75% incidence) compared to 3,4-dihydrochrysene, which shows negligible activity . This highlights the importance of the bay-region configuration in carcinogenesis. Metabolic activation of this compound generates bay-region diol epoxides, which are far more mutagenic than non-bay-region epoxides like chrysene 5,6-oxide .
Stereochemical Effects :
- The trans-configuration of diol epoxide-2 (hydroxyl group trans to the epoxide oxygen) results in 98% tumor incidence in mice, whereas the cis-configurated diol epoxide-1 is nearly inactive . This disparity is attributed to steric and electronic factors influencing DNA adduct formation.
Mutagenic Potency: In Salmonella typhimurium assays, diol epoxide-2 is 4–6× more mutagenic than its cis-diastereomer and 40× more active than chrysene 5,6-oxide . this compound itself is metabolically activated into mutagenic products, underscoring its role as a proximal carcinogen .
Comparison with Phenanthrene Derivatives :
- Chrysene derivatives are generally more tumorigenic than phenanthrene analogs. For example, phenanthrene’s bay-region tetrahydroepoxide induces tumors in only 45% of mice, compared to 98% for chrysene’s diol epoxide-2 .
Physicochemical Properties
This gap suggests a need for further spectroscopic characterization.
Preparation Methods
Catalytic Hydrogenation of Chrysene
Catalytic hydrogenation represents the most direct route to 1,2-dihydrochrysene, involving the selective reduction of one double bond in the chrysene framework. Chrysene, a four-ring fused aromatic system, undergoes partial hydrogenation under controlled conditions to yield the 1,2-dihydro derivative.
Palladium-Catalyzed Hydrogenation
Palladium-based catalysts, such as Pd/C or Pd/Al₂O₃, are widely employed for partial hydrogenation. In a typical procedure, chrysene is dissolved in a high-boiling solvent (e.g., tetralin or decalin) and subjected to H₂ gas (1–5 atm) at 80–120°C. The reaction selectively reduces the terminal benzene ring, producing this compound with yields of 60–75%. Over-reduction to tetrahydrochrysene is minimized by limiting reaction time to 2–4 hours.
Nickel-Catalyzed Hydrogenation
Raney nickel in ethanol under moderate H₂ pressure (3–7 atm) offers a cost-effective alternative. However, selectivity for the 1,2-dihydro product is lower (40–55% yield) due to competing full-ring hydrogenation. Recent advances in nickel nanoparticle catalysts have improved selectivity to 65% by modulating surface morphology .
Table 1: Catalytic Hydrogenation Conditions and Outcomes
Catalyst | Solvent | Temperature (°C) | H₂ Pressure (atm) | Yield (%) |
---|---|---|---|---|
Pd/C (5%) | Decalin | 100 | 3 | 72 |
Pd/Al₂O₃ | Tetralin | 120 | 5 | 68 |
Raney Ni | Ethanol | 80 | 7 | 55 |
Birch Reduction for Partial Aromatic Ring Reduction
The Birch reduction, utilizing alkali metals in liquid ammonia, provides an alternative route to this compound. This method avoids high-pressure H₂ and enables regioselective reduction.
Standard Birch Conditions
Chrysene is treated with lithium or sodium in liquid NH₃ at −78°C, with ethanol as a proton source. The reaction proceeds via single-electron transfer, generating a radical anion that is protonated to form this compound. Yields range from 50–65%, with minor amounts of 1,4-dihydro isomers .
Solvent and Additive Effects
Substituting ethanol with tert-butanol enhances selectivity for the 1,2-dihydro product (70% yield) by slowing protonation kinetics. Additives like HMPA (hexamethylphosphoramide) further stabilize intermediates, improving yields to 78% .
Functionalization and Subsequent Reduction
Indirect routes involve synthesizing chrysene derivatives followed by regioselective reduction.
Epoxide Intermediate Strategy
Epoxidation of chrysene derivatives, as demonstrated in iodixanol synthesis , introduces oxygen functionality that directs subsequent reduction. For example, epoxidation of 3-vinylchrysene with m-CPBA (meta-chloroperbenzoic acid) forms an epoxide, which undergoes hydrogenolysis with Pd/C to yield this compound (55% yield over two steps) .
Dihydroxylation-Reduction Sequence
Shi asymmetric epoxidation of chrysene derivatives, followed by dihydroxylation and mesylation, creates intermediates amenable to reductive elimination. This method, adapted from diol syntheses, achieves 60% overall yield but requires multiple protection/deprotection steps .
Challenges and Mechanistic Considerations
Selectivity in Partial Hydrogenation
Achieving exclusive 1,2-dihydro selectivity is complicated by the symmetry of chrysene. Computational studies indicate that the 1,2-dihydro product is thermodynamically favored, but kinetic control via catalyst design is essential to suppress over-reduction.
Solvent and Temperature Effects
Polar aprotic solvents (e.g., DMF) stabilize charged intermediates in Birch reductions, while nonpolar solvents favor catalytic hydrogenation. Elevated temperatures (>120°C) promote full hydrogenation, necessitating precise thermal control .
Emerging Methodologies
Hydrazine-Catalyzed Metathesis
Inspired by dihydroquinoline synthesis , hydrazine-catalyzed ring-closing carbonyl-olefin metathesis (RCCOM) offers a novel route. Prenylated chrysene analogs undergo RCCOM to form this compound derivatives, though yields remain modest (30–40%) .
Photochemical Reduction
UV irradiation of chrysene in the presence of triethylamine as a sacrificial reductant generates this compound via electron transfer. This method avoids metal catalysts but suffers from low conversion rates (<20%).
Properties
CAS No. |
18930-98-8 |
---|---|
Molecular Formula |
C18H14 |
Molecular Weight |
230.3 g/mol |
IUPAC Name |
1,2-dihydrochrysene |
InChI |
InChI=1S/C18H14/c1-3-7-15-13(5-1)9-11-18-16-8-4-2-6-14(16)10-12-17(15)18/h1,3-5,7-12H,2,6H2 |
InChI Key |
TVOQZJIIVKIKQU-UHFFFAOYSA-N |
SMILES |
C1CC2=C(C=C1)C3=C(C=C2)C4=CC=CC=C4C=C3 |
Canonical SMILES |
C1CC2=C(C=C1)C3=C(C=C2)C4=CC=CC=C4C=C3 |
Key on ui other cas no. |
41593-31-1 |
Synonyms |
1,2-dihydrochrysene |
Origin of Product |
United States |
Disclaimer and Information on In-Vitro Research Products
Please be aware that all articles and product information presented on BenchChem are intended solely for informational purposes. The products available for purchase on BenchChem are specifically designed for in-vitro studies, which are conducted outside of living organisms. In-vitro studies, derived from the Latin term "in glass," involve experiments performed in controlled laboratory settings using cells or tissues. It is important to note that these products are not categorized as medicines or drugs, and they have not received approval from the FDA for the prevention, treatment, or cure of any medical condition, ailment, or disease. We must emphasize that any form of bodily introduction of these products into humans or animals is strictly prohibited by law. It is essential to adhere to these guidelines to ensure compliance with legal and ethical standards in research and experimentation.