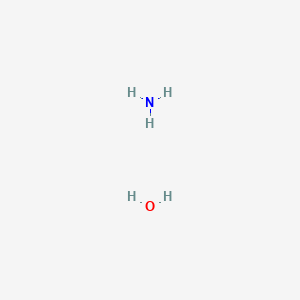
Azane;hydrate
Overview
Description
Azane (NH₃), systematically named ammonia, is a colorless gas with a pungent odor. This ambiguity necessitates a comparative analysis with structurally or functionally related compounds, such as methane clathrate hydrates, hydrazine hydrate (N₂H₄·H₂O), and coordination complexes containing ammonia ligands .
Preparation Methods
High-Purity Ammonium Hydroxide Synthesis
For applications requiring ultra-high purity (e.g., semiconductor manufacturing), specialized methods are employed to minimize contaminants such as metal ions and organic residues. Patent US5746993A outlines a continuous process for producing ammonium hydroxide with purity levels exceeding 99.9999% .
Reactor Design and Sparging Techniques
The process utilizes a perfluoralkoxy (PFA) sparge tube immersed in deionized water within a pressurized reactor . Key features include:
-
Perforated sparge tubes to maximize gas-liquid contact.
-
Baffle plates (Figure 2) to enhance mixing and prevent channeling.
-
Cooling jackets maintaining temperatures below 10°C to stabilize the exothermic dissolution .
Process Optimization
-
Pressure Regulation : Operating at 0.1–3 MPa ensures efficient gas dissolution while avoiding aerosol formation .
-
Flow Dynamics : A water-to-ammonia molar ratio of 3:7 optimizes reaction kinetics, achieving near-quantitative conversion .
-
Two-Stage Reactors : Secondary reactors further concentrate the solution to 45% w/w NH₃ by reintroducing ammonia into partially saturated solutions .
Comparative Analysis of Synthesis Methods
The Haber-Bosch method prioritizes scalability and cost-efficiency , whereas high-purity processes focus on contaminant control and precision .
Emerging Technologies and Innovations
Solvent-Free Hydrazine Recovery
Innovations in ketazine hydrolysis (e.g., US5986134A) recover hydrazine hydrate without solvents, though these methods are tangential to ammonium hydroxide synthesis .
Chemical Reactions Analysis
Types of Reactions
Ammonium hydroxide undergoes various types of chemical reactions, including:
Neutralization: Reacts with acids to form ammonium salts.
Precipitation: Forms precipitates with certain metal ions.
Complexation: Acts as a ligand to form complex ions with transition metals.
Common Reagents and Conditions
Acids: Reacts with acids like hydrochloric acid (HCl) to form ammonium chloride (NH4Cl).
Metal Ions: Reacts with metal ions like copper(II) ions (Cu2+) to form complex ions such as tetraamminecopper(II) ([Cu(NH3)4]2+).
Major Products
Ammonium Salts: Formed by neutralization reactions with acids.
Complex Ions: Formed by reaction with transition metal ions.
Scientific Research Applications
Ammonium hydroxide solution has a wide range of applications in scientific research:
Chemistry: Used as a reagent in various chemical syntheses and analytical procedures.
Biology: Employed in the preparation of biological samples and as a buffer solution.
Medicine: Utilized in the formulation of certain pharmaceuticals and as a cleaning agent in medical laboratories.
Mechanism of Action
Ammonium hydroxide exerts its effects primarily through its basic nature. It can donate hydroxide ions (OH-) in aqueous solutions, which can neutralize acids and participate in various chemical reactions. The molecular targets and pathways involved include:
Neutralization: Reacts with hydrogen ions (H+) to form water.
Complexation: Forms coordination complexes with metal ions.
Comparison with Similar Compounds
Comparative Analysis of Clathrate Hydrates: Methane vs. Potential Ammonia Hydrate
Clathrate hydrates are crystalline compounds where gas molecules (e.g., methane) occupy cages formed by water molecules. Key properties include:
Methane hydrate decomposes adiabatically, releasing gas and water (Figure 3, ).
Ammonium Hydroxide: Aqueous Solution vs. Solid Hydrate
Ammonium hydroxide (NH₄OH) is a weak base formed when NH₃ dissolves in water. Unlike solid hydrates, it exists as an equilibrium mixture of NH₃, NH₄⁺, and OH⁻. Key distinctions from clathrates:
Ammonium hydroxide’s role in meat spoilage (producing NH₃ from proteins ) highlights its reactivity compared to inert methane in clathrates.
Coordination Complexes Involving Azane and Hydrate
Ammonia acts as a ligand in coordination chemistry, forming hydrates with metals. Examples include:
- Tetraammineplatinum(II) chloride monohydrate ([Pt(NH₃)₄]Cl₂·H₂O): Used in catalysis, with a melting point of 250°C (dec.) .
- Tetraammineplatinum(II) hydroxide hydrate (Pt(NH₃)₄₂·xH₂O): Stabilized by hydrogen bonding between NH₃ and H₂O .
These complexes contrast with gas hydrates, as their "hydrate" component refers to crystalline water rather than clathrate structures.
Q & A
Basic Research Questions
Q. What are the recommended methods for synthesizing azane hydrates in laboratory settings?
Azane hydrates (e.g., hydrazine hydrate) can be synthesized by reacting hydrochlorides of amino acid esters with excess hydrazine hydrate in ethanol under reflux, followed by recrystallization from methanol . For metal-ammine complexes (e.g., tetraammineplatinum(II) chloride monohydrate), synthesis involves controlled ammoniation of metal salts in aqueous solutions, with stoichiometric water incorporation confirmed via thermogravimetric analysis (TGA) . Key parameters include molar ratios (e.g., 1:1.2 for hydrazine reactions) and solvent selection to optimize yield and purity.
Q. Which analytical techniques are essential for characterizing the stoichiometry and stability of azane hydrates?
- Thermogravimetric Analysis (TGA): Determines hydration state by measuring mass loss upon heating (e.g., distinguishing monohydrate vs. hexahydrate forms) .
- X-ray Diffraction (XRD): Identifies crystalline structure and confirms hydrate formation via lattice parameter comparisons .
- Nuclear Magnetic Resonance (NMR): Resolves proton environments in hydrated ammonia complexes (e.g., distinguishing NH₃·H₂O from NH₄⁺ in solution) .
- Elemental Analysis: Validates empirical formulas (e.g., MgNH₄PO₄·6H₂O in magnesium ammonium phosphate hexahydrate) .
Q. How can researchers safely handle azane hydrates in experimental workflows?
- Ventilation: Use fume hoods to mitigate ammonia vapor exposure, as azane hydrates release NH₃ upon decomposition .
- Personal Protective Equipment (PPE): Wear nitrile gloves and goggles to prevent skin/eye contact, especially with corrosive derivatives like phosphomolybdic acid hydrate .
- Storage: Keep samples in airtight containers with desiccants to prevent unintended hydration/dehydration .
Advanced Research Questions
Q. How can computational modeling resolve contradictions in hydration state predictions for azane-containing complexes?
Molecular dynamics (MD) simulations can model water-ammonia interactions under varying temperatures and pressures, predicting stable hydration states (e.g., monohydrate vs. trihydrate). Density Functional Theory (DFT) calculations further optimize hydrogen-bonding networks in crystal lattices . Experimental validation via in situ Raman spectroscopy is critical to reconcile discrepancies between predicted and observed hydration states .
Q. What advanced detection methods enable real-time tracking of azane (ammonia) dimers in microbial metabolic studies?
MCC-IMS (Multicapillary Column-Ion Mobility Spectrometry): Detects volatile ammonia dimers (2NH₃ → (NH₃)₂) in pathogen headspace samples. Dimers form preferentially during late bacterial growth phases (e.g., Pseudomonas aeruginosa), with ion mobility drift times calibrated against known standards . This method achieves ppm-level sensitivity, enabling dynamic monitoring of microbial ammonia production.
Q. How can researchers optimize catalytic hydration reactions involving azane hydrates?
- Catalyst Screening: Test transition-metal complexes (e.g., Ni/phosphine) for alkene hydration, monitoring conversion rates via GC-MS .
- Kinetic Studies: Vary reaction parameters (pH, temperature) to distinguish between acid-catalyzed (H₃O⁺-mediated) and metal-catalyzed mechanisms .
- In Situ Spectroscopy: Use FTIR to track intermediate species (e.g., NH₄⁺ or NH₃·H₂O) during catalytic cycles .
Q. What strategies address reproducibility challenges in synthesizing Schweizer's reagent (a copper-azane hydrate complex)?
- Stoichiometric Control: Maintain a 4:1 NH₃:Cu²⁺ ratio to prevent over-ammoniation, which destabilizes the complex .
- pH Monitoring: Adjust to pH 10–12 using NH₄OH to stabilize tetraamminecopper(II) ions .
- Purification: Employ dialysis to remove unreacted ammonia and byproducts, ensuring reagent consistency for cellulose dissolution studies .
Q. Methodological Considerations
- Data Contradiction Analysis: Compare TGA-derived hydration states with XRD results to identify artifacts from incomplete dehydration or atmospheric rehydration .
- Experimental Design: For microbial studies, include negative controls (e.g., sterile media) to isolate azane dimer signals from background ammonia .
- Literature Review: Prioritize primary sources (e.g., Journal of Economic, Administrative and Legal Sciences) over commercial databases to avoid biased data .
Properties
CAS No. |
16393-49-0 |
---|---|
Molecular Formula |
NH4OH H5NO |
Molecular Weight |
35.046 g/mol |
IUPAC Name |
azane;hydrate |
InChI |
InChI=1S/H3N.H2O/h1H3;1H2 |
InChI Key |
VHUUQVKOLVNVRT-UHFFFAOYSA-N |
SMILES |
N.O |
Canonical SMILES |
[NH4+].[OH-] |
boiling_point |
38 °C (25%) |
Color/Form |
Colorless liquid |
density |
About 0.90 @ 25 °C/25 °C Relative density (water = 1): 0.9 (25%) |
melting_point |
-58 °C (25%) |
Key on ui other cas no. |
1336-21-6 |
physical_description |
Ammonium hydroxide appears as a colorless aqueous solution. Concentration of ammonia ranges up to approximately 30%. Ammonia vapors (which arise from the solution) irritate the eyes. DryPowder; Liquid; WetSolid Clear, colourless solution, having an exceedingly pungent, characteristic odour VERY VOLATILE COLOURLESS AMMONIA SOLUTION IN WATER WITH PUNGENT ODOUR. |
Pictograms |
Corrosive; Environmental Hazard |
solubility |
Exists only in solution Solubility in water: miscible |
Synonyms |
ammonium hydroxide Hydroxide, Ammonium |
vapor_density |
Relative vapor density (air = 1): 0.6 |
vapor_pressure |
2160 mm Hg @ 25 °C Vapor pressure, kPa at 20 °C: 48 (25%) |
Origin of Product |
United States |
Synthesis routes and methods I
Procedure details
Synthesis routes and methods II
Procedure details
Synthesis routes and methods III
Procedure details
Synthesis routes and methods IV
Procedure details
Synthesis routes and methods V
Procedure details
Retrosynthesis Analysis
AI-Powered Synthesis Planning: Our tool employs the Template_relevance Pistachio, Template_relevance Bkms_metabolic, Template_relevance Pistachio_ringbreaker, Template_relevance Reaxys, Template_relevance Reaxys_biocatalysis model, leveraging a vast database of chemical reactions to predict feasible synthetic routes.
One-Step Synthesis Focus: Specifically designed for one-step synthesis, it provides concise and direct routes for your target compounds, streamlining the synthesis process.
Accurate Predictions: Utilizing the extensive PISTACHIO, BKMS_METABOLIC, PISTACHIO_RINGBREAKER, REAXYS, REAXYS_BIOCATALYSIS database, our tool offers high-accuracy predictions, reflecting the latest in chemical research and data.
Strategy Settings
Precursor scoring | Relevance Heuristic |
---|---|
Min. plausibility | 0.01 |
Model | Template_relevance |
Template Set | Pistachio/Bkms_metabolic/Pistachio_ringbreaker/Reaxys/Reaxys_biocatalysis |
Top-N result to add to graph | 6 |
Feasible Synthetic Routes
Disclaimer and Information on In-Vitro Research Products
Please be aware that all articles and product information presented on BenchChem are intended solely for informational purposes. The products available for purchase on BenchChem are specifically designed for in-vitro studies, which are conducted outside of living organisms. In-vitro studies, derived from the Latin term "in glass," involve experiments performed in controlled laboratory settings using cells or tissues. It is important to note that these products are not categorized as medicines or drugs, and they have not received approval from the FDA for the prevention, treatment, or cure of any medical condition, ailment, or disease. We must emphasize that any form of bodily introduction of these products into humans or animals is strictly prohibited by law. It is essential to adhere to these guidelines to ensure compliance with legal and ethical standards in research and experimentation.