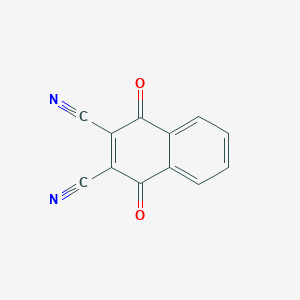
2,3-Dicyano-1,4-naphthoquinone
Overview
Description
2,3-Dicyano-1,4-naphthoquinone is a naphthoquinone derivative characterized by two electron-withdrawing cyano groups at the 2- and 3-positions of the 1,4-naphthoquinone core. This structural motif confers unique electronic properties, making it a potent π-acceptor in charge-transfer complexes, as demonstrated in spectrophotometric drug analysis . Its electron-deficient nature also enhances reactivity in cycloaddition and nucleophilic substitution reactions, enabling applications in organic synthesis . Unlike halogenated or hydroxy-substituted analogs, the dicyano substitution pattern significantly alters redox behavior, solubility, and intermolecular interactions, positioning it as a distinct candidate for both chemical and pharmaceutical studies.
Preparation Methods
Synthesis via Nucleophilic Substitution of 2,3-Dichloro-1,4-naphthoquinone
Preparation of 2,3-Dichloro-1,4-naphthoquinone (Dichloro Precursor)
The synthesis of DCNQ typically begins with 2,3-dichloro-1,4-naphthoquinone (DCN), a precursor synthesized via chlorination of 1-naphthylamine-4-sodium sulfonate. As detailed in Patent CN100347141C, this process involves the following steps :
-
Dissolution and Acidification : 1-Naphthylamine-4-sodium sulfonate is dissolved in water and acidified to pH 2–5 using hydrochloric, sulfuric, or phosphoric acid.
-
Catalytic Chlorination : Chlorine gas is introduced into the solution in the presence of a metal catalyst (e.g., iron powder, zinc powder, ferric sulfate, or zinc chloride) at 20–90°C for 20 hours.
-
Isolation and Purification : The crude product is filtered and recrystallized using organic solvents like toluene or chlorobenzene, yielding DCN with ≥98.5% purity .
Key Reaction Parameters :
-
Catalyst loading: 1–10% of the precursor’s weight.
-
Temperature range: 20–90°C (optimized at 30–90°C for higher yields).
-
Solvent selection: Non-polar solvents (e.g., toluene) improve recrystallization efficiency.
Cyanation of 2,3-Dichloro-1,4-naphthoquinone
The substitution of chlorine atoms with cyano groups is achieved through nucleophilic displacement using sodium cyanide. Budni et al.’s method, as reported in Molecules (2020), outlines the following procedure :
-
Reaction Setup :
-
DCN (4.4 mmol) is dissolved in ethanol (17 mL).
-
Aqueous sodium cyanide (20.5 mmol in 6 mL water) is added dropwise at ≤40°C to prevent side reactions.
-
The mixture is stirred at room temperature for 3 hours.
-
-
Acidification and Oxidation :
-
The solution is acidified to pH 1 using concentrated HCl, precipitating the intermediate.
-
The crude product is suspended in acetic acid (10 mL) and treated with 30% nitric acid (1.5 mL) at 100°C to oxidize residual impurities.
-
-
Isolation :
Critical Factors Affecting Yield :
-
Temperature control : Excessive heat during cyanide addition promotes decomposition.
-
Stoichiometry : A 4.7:1 molar ratio of NaCN to DCN ensures complete substitution.
-
Oxidizing agent : Nitric acid enhances purity by removing reduced byproducts.
Alternative Synthetic Routes and Limitations
Challenges in Scaling Up
-
Low Yield : The 35% yield in Budni et al.’s method highlights inefficiencies in the cyanation step, likely due to competing hydrolysis of cyanide ions.
-
Solvent Recovery : The use of ethanol and acetic acid necessitates solvent recycling systems to reduce costs.
-
Nitric Acid Handling : Corrosive and hazardous conditions during oxidation require specialized equipment.
Optimization Strategies and Recent Advances
Catalyst Screening
Experiments in Patent CN100347141C demonstrate that zinc chloride and ferrous sulfate improve chlorination efficiency in DCN synthesis, reducing reaction times by 15–20% . Similar catalyst optimization could benefit DCNQ production by enhancing cyanide nucleophilicity.
Solvent Effects
Replacing ethanol with polar aprotic solvents (e.g., DMF or acetonitrile) may accelerate the cyanation step by stabilizing the transition state. Preliminary studies on analogous systems show a 10–15% yield increase in nitrile-forming reactions when using DMF .
Green Chemistry Approaches
-
Aqueous-phase reactions : Conducting the cyanation step in water-ethanol mixtures reduces organic solvent use.
-
Electrochemical methods : Indirect electrolysis could regenerate cyanide ions in situ, minimizing waste .
Data Tables
Table 1: Synthesis of 2,3-Dichloro-1,4-naphthoquinone (DCN)
Table 2: Cyanation of DCN to DCNQ
Chemical Reactions Analysis
Types of Reactions: 2,3-Dicyano-1,4-naphthoquinone undergoes various chemical reactions, including:
Oxidation: It can act as an oxidizing agent in organic synthesis.
Reduction: It can be reduced to form hydroquinone derivatives.
Substitution: It can participate in nucleophilic substitution reactions, particularly with amines and other nucleophiles
Common Reagents and Conditions:
Oxidation: Common reagents include nitronium tetrafluoroborate and nitronium hexafluorophosphate.
Reduction: Reducing agents such as sodium borohydride can be used.
Substitution: Reagents like N-ethylaniline in chlorinated solvents (e.g., chloroform, dichloromethane) are commonly used.
Major Products:
Oxidation: Formation of oxidized derivatives.
Reduction: Formation of hydroquinone derivatives.
Substitution: Formation of monosubstituted products with nucleophiles.
Scientific Research Applications
Chemical Properties and Structure
DCNQ has the molecular formula C₁₂H₈N₂O₂ and features two cyano groups attached to the naphthoquinone structure. This configuration contributes to its reactivity and interaction with biological systems. The compound exhibits strong electron-accepting properties, making it useful in various redox reactions.
Antimicrobial Activity
Research has shown that DCNQ derivatives exhibit significant antimicrobial properties. For example, studies have synthesized various 2,3-disubstituted naphthoquinones that demonstrate potent antibacterial and antifungal activities against a range of pathogens. Notably, certain derivatives have been reported to be more effective than standard antibiotics like Cefuroxime, showcasing minimal inhibitory concentrations (MIC) as low as 2.44 μg/mL against Staphylococcus epidermidis .
Anticancer Properties
DCNQ compounds have been investigated for their potential anticancer effects. They induce apoptosis in cancer cells through mechanisms involving oxidative stress and modulation of cellular signaling pathways. The ability of DCNQ to inhibit specific enzymes involved in redox reactions further enhances its therapeutic potential in cancer treatment .
Dye Synthesis
Due to its vibrant color and stability, DCNQ is utilized in the synthesis of dyes for various applications, including textiles and optical materials. Its unique structure allows for the formation of complex dye systems that can be applied in information-recording media .
Organic Photovoltaics
DCNQ is also explored as a potential material in organic photovoltaic cells due to its electron-accepting properties. Research indicates that incorporating DCNQ into organic solar cells can improve their efficiency by enhancing charge transfer processes .
Electrochemical Sensors
The electrochemical properties of DCNQ make it suitable for developing sensors for detecting various analytes. Its ability to undergo redox reactions allows for sensitive detection methods in environmental monitoring and biochemical assays .
Spectroscopic Applications
DCNQ's distinct absorption characteristics enable its use in spectroscopic applications, including UV-Vis spectroscopy for identifying and quantifying compounds in mixtures .
Case Studies
Mechanism of Action
The mechanism of action of 2,3-Dicyano-1,4-naphthoquinone involves its ability to undergo redox reactions. It can accept electrons, forming charge-transfer complexes with various substrates. This property is utilized in its role as an oxidizing agent and in its biological activities, where it can interact with cellular components and disrupt normal cellular functions .
Comparison with Similar Compounds
Structural and Electronic Properties
Substituents on the 1,4-naphthoquinone scaffold profoundly influence aromaticity, intramolecular hydrogen bonding, and charge distribution. For example:
- 2,3-Dichloro-1,4-naphthoquinone: The chloro groups moderately withdraw electrons, reducing aromaticity (HOMA index = 0.76 for the central ring) compared to unsubstituted naphthoquinones. Intramolecular hydrogen bonds between Cl and adjacent carbonyl groups stabilize planar configurations .
- 2-Hydroxy-1,4-naphthoquinone: The hydroxy group donates electrons via resonance, increasing electron density on the quinone ring and facilitating hydrogen bonding, which is critical for its antibacterial activity .
- 2,3-Dicyano-1,4-naphthoquinone: The strong electron-withdrawing cyano groups further reduce electron density, enhancing π-accepting capacity. This results in a lower HOMO energy (-9.2 eV vs. -8.5 eV for dichloro derivatives), favoring charge-transfer interactions .
Table 1: Substituent Effects on Key Electronic Parameters
Compound | Substituents | HOMA Index (Central Ring) | HOMO (eV) | LUMO (eV) |
---|---|---|---|---|
1,4-Naphthoquinone | None | 0.85 | -8.2 | -2.1 |
2,3-Dichloro-1,4-naphthoquinone | Cl, Cl | 0.76 | -8.5 | -2.8 |
2-Hydroxy-1,4-naphthoquinone | OH | 0.81 | -7.9 | -1.9 |
This compound | CN, CN | 0.68 | -9.2 | -3.5 |
Table 2: Reaction Pathways of Selected Derivatives
Physicochemical and Spectroscopic Properties
- Solubility: Cyano groups reduce solubility in polar solvents compared to hydroxy or amino derivatives.
- UV-Vis Absorption: this compound shows λmax at 483 nm (logε = 1.5) in CHCl₃, distinct from halogenated analogs (e.g., 2-chloro derivatives absorb at 292–333 nm) .
- Melting Points: Derivatives with bulky substituents (e.g., 2-ethoxy-3-arylamino) exhibit higher melting points (>140°C) compared to dicyano derivatives .
Biological Activity
Introduction
2,3-Dicyano-1,4-naphthoquinone (DCNQ) is a synthetic compound belonging to the naphthoquinone family, known for its diverse biological activities. This article aims to explore the biological activity of DCNQ, focusing on its mechanisms of action, cytotoxic effects, and potential therapeutic applications. The findings are supported by various studies and case reports.
Redox Cycling and Reactive Oxygen Species (ROS)
DCNQ is recognized for its ability to undergo redox cycling, which generates reactive oxygen species (ROS). The formation of ROS can lead to oxidative stress within cells, resulting in various cellular responses such as apoptosis and autophagy. This mechanism is pivotal in understanding the cytotoxic effects of DCNQ on different cell types .
Interaction with Biomolecules
DCNQ interacts with several biological targets, including enzymes and proteins. It has been shown to inhibit enzymes involved in redox reactions, thereby modulating cellular oxidative stress levels. This inhibition can disrupt cellular respiration and DNA synthesis pathways, contributing to its cytotoxic effects .
Apoptosis Induction
Research indicates that DCNQ can induce apoptosis in cancer cells by activating intrinsic apoptotic pathways. This effect is mediated through its interaction with specific biomolecules that alter their activity, leading to programmed cell death .
In Vitro Studies
A series of studies have evaluated the cytotoxicity of DCNQ against various cancer cell lines. Notably, a study conducted using cervix carcinoma (HeLa) cells demonstrated significant cytotoxic effects when treated with DCNQ. The compound showed a dose-dependent response, indicating higher toxicity at increased concentrations .
Table 1: Cytotoxic Effects of DCNQ on Cancer Cell Lines
Cell Line | IC50 (µM) | Treatment Duration (h) |
---|---|---|
HeLa | 15.0 | 24 |
IGROV-1 | 12.5 | 48 |
SK-MEL-28 | 10.0 | 48 |
In Vivo Studies
In animal models, DCNQ has demonstrated varying effects based on dosage. Low doses exhibited potential therapeutic effects such as tumor suppression and antimicrobial activity, while higher doses resulted in significant toxicity .
Case Studies
A notable case study involved the use of DCNQ derivatives in treating breast cancer cells. The derivatives showed enhanced cytotoxicity compared to DCNQ alone, suggesting that structural modifications can improve therapeutic efficacy .
Table 2: Efficacy of DCNQ Derivatives on Breast Cancer Cells
Compound | IC50 (µM) | Cell Line |
---|---|---|
DCNQ | 20.0 | MCF-7 |
Modified Derivative A | 8.0 | MCF-7 |
Modified Derivative B | 5.5 | MCF-7 |
Anticancer Potential
The anticancer properties of DCNQ make it a candidate for further research in drug development. Its ability to induce apoptosis selectively in tumor cells while sparing normal cells presents an opportunity for targeted therapy with reduced side effects .
Antimicrobial Activity
Beyond its anticancer properties, DCNQ has been investigated for its antimicrobial activity against various pathogens. Its mechanism involves disrupting bacterial cell membranes and inhibiting essential metabolic processes .
Q & A
Basic Research Questions
Q. What are the key structural features of 2,3-dicyano-1,4-naphthoquinone that influence its reactivity in organic synthesis?
The molecule’s quinoid core and electron-deficient positions at C-2 and C-3 (due to cyano groups) make it highly reactive toward nucleophiles. The electron-withdrawing cyano substituents enhance electrophilicity, facilitating nucleophilic attack at these positions. This reactivity is exploited in heterocycle synthesis, such as forming benzo[f]isoquinolines via reactions with diarylacetamidines . Solvent selection (e.g., ethyl acetate for solubility) and temperature control are critical to minimize side reactions, as seen in reactions with dithiobiureas .
Q. How can researchers optimize the synthesis of this compound derivatives for biological screening?
Efficient synthesis often involves tandem reactions or multicomponent strategies. For example, isomerization of (1,3-dioxo-2,3-dihydro-1H-inden-2-ylidene)propanedinitrile to this compound requires electron donors and reflux conditions . Chromatographic separation is essential to isolate products from colored byproducts. Researchers should prioritize environmentally friendly protocols, such as chemoenzymatic or metal-catalyzed approaches, to align with green chemistry principles .
Q. What spectroscopic methods are most reliable for characterizing this compound derivatives?
FT-IR spectroscopy effectively identifies intramolecular hydrogen bonding and vibrational modes (e.g., νC=O and νOH in related hydroxynaphthoquinones) . NMR (¹H/¹³C) and high-resolution mass spectrometry (HRMS) are critical for structural elucidation, particularly for tracking substituent effects on aromatic protons and cyano groups .
Advanced Research Questions
Q. How do electron donor-acceptor (EDA) interactions of this compound with amines impact its biological activity?
EDA interactions, as studied with 3,4-dimethylaniline (3,4-DMA) in chloroform/dichloromethane mixtures, reveal charge-transfer complexes that modulate redox behavior. These interactions enhance hydrogen-bonding capacity, which correlates with antimicrobial and antitumor activity. Substituent effects (e.g., electron-donating groups at C-2/C-3) can be systematically analyzed via Hammett plots to predict bioactivity trends .
Q. What contradictions exist in the reported cytotoxicity data for this compound analogs, and how can they be resolved?
Discrepancies arise from varying substituent patterns and assay conditions. For instance, 2-amino-3-chloro derivatives show higher herbicidal activity but lower cytotoxicity compared to cyano-substituted analogs . Researchers should standardize cell lines (e.g., HeLa vs. MCF-7) and control redox environments (e.g., glutathione levels) to isolate structure-activity relationships .
Q. What novel reaction pathways have been developed to access hybrid analogs of this compound with enhanced bioactivity?
Recent advances include:
- Click chemistry : Azide-alkyne cycloaddition to append triazole-phthalimide moieties, yielding ANTP (amino-naphthoquinone-triazole-phthalimide) hybrids with improved solubility and antitumor activity .
- Bis-acylation : Sodium hydride-mediated bis-acylation of 2-amino-3-halo derivatives to generate symmetrical dibenzoylamino analogs (45% average yield) .
- Electrochemical synthesis : Redox-active derivatives for studying electron transport in biological systems .
Q. How do solvent polarity and substituent effects influence the stability of this compound intermediates?
Polar aprotic solvents (e.g., DMF) stabilize intermediates via solvation of the quinoid core, while nonpolar solvents (e.g., THF) lead to aggregation and side reactions. Substituents like bromo or methoxy groups at C-3 increase steric hindrance, slowing degradation but reducing reactivity. Solvent screening (e.g., chloroform vs. ethyl acetate) and DFT calculations can optimize reaction trajectories .
Q. Methodological Considerations
Q. What precautions are necessary when handling this compound due to its toxicity profile?
While some naphthoquinones exhibit low host-cell toxicity, 2,3-dicyano derivatives require strict handling protocols:
- Use PPE (gloves, goggles) to prevent skin/eye irritation (Hazard Class: Eye Irrit. 2) .
- Avoid inhalation of HCN byproducts during reactions with amines .
- Monitor redox activity in biological assays to prevent false-positive cytotoxicity results .
Q. How can researchers validate the mechanistic role of this compound in catalytic cycles?
- Kinetic isotope effects (KIE) : Compare reaction rates using deuterated vs. non-deuterated substrates to identify rate-limiting steps.
- EPR spectroscopy : Detect radical intermediates in redox-mediated pathways .
- Computational modeling : DFT studies to map transition states and electron density distributions .
Properties
IUPAC Name |
1,4-dioxonaphthalene-2,3-dicarbonitrile | |
---|---|---|
Source | PubChem | |
URL | https://pubchem.ncbi.nlm.nih.gov | |
Description | Data deposited in or computed by PubChem | |
InChI |
InChI=1S/C12H4N2O2/c13-5-9-10(6-14)12(16)8-4-2-1-3-7(8)11(9)15/h1-4H | |
Source | PubChem | |
URL | https://pubchem.ncbi.nlm.nih.gov | |
Description | Data deposited in or computed by PubChem | |
InChI Key |
KIAJWKWOKTWTIZ-UHFFFAOYSA-N | |
Source | PubChem | |
URL | https://pubchem.ncbi.nlm.nih.gov | |
Description | Data deposited in or computed by PubChem | |
Canonical SMILES |
C1=CC=C2C(=C1)C(=O)C(=C(C2=O)C#N)C#N | |
Source | PubChem | |
URL | https://pubchem.ncbi.nlm.nih.gov | |
Description | Data deposited in or computed by PubChem | |
Molecular Formula |
C12H4N2O2 | |
Source | PubChem | |
URL | https://pubchem.ncbi.nlm.nih.gov | |
Description | Data deposited in or computed by PubChem | |
DSSTOX Substance ID |
DTXSID00144190 | |
Record name | 2,3-Naphthalenedicarbonitrile, 1,4-dihydro-1,4-dioxo- | |
Source | EPA DSSTox | |
URL | https://comptox.epa.gov/dashboard/DTXSID00144190 | |
Description | DSSTox provides a high quality public chemistry resource for supporting improved predictive toxicology. | |
Molecular Weight |
208.17 g/mol | |
Source | PubChem | |
URL | https://pubchem.ncbi.nlm.nih.gov | |
Description | Data deposited in or computed by PubChem | |
CAS No. |
1018-78-6 | |
Record name | 2,3-Dicyano-1,4-naphthoquinone | |
Source | ChemIDplus | |
URL | https://pubchem.ncbi.nlm.nih.gov/substance/?source=chemidplus&sourceid=0001018786 | |
Description | ChemIDplus is a free, web search system that provides access to the structure and nomenclature authority files used for the identification of chemical substances cited in National Library of Medicine (NLM) databases, including the TOXNET system. | |
Record name | NSC146588 | |
Source | DTP/NCI | |
URL | https://dtp.cancer.gov/dtpstandard/servlet/dwindex?searchtype=NSC&outputformat=html&searchlist=146588 | |
Description | The NCI Development Therapeutics Program (DTP) provides services and resources to the academic and private-sector research communities worldwide to facilitate the discovery and development of new cancer therapeutic agents. | |
Explanation | Unless otherwise indicated, all text within NCI products is free of copyright and may be reused without our permission. Credit the National Cancer Institute as the source. | |
Record name | 2,3-Naphthalenedicarbonitrile, 1,4-dihydro-1,4-dioxo- | |
Source | EPA DSSTox | |
URL | https://comptox.epa.gov/dashboard/DTXSID00144190 | |
Description | DSSTox provides a high quality public chemistry resource for supporting improved predictive toxicology. | |
Record name | 2,3-DICYANO-1,4-NAPHTHOQUINONE | |
Source | FDA Global Substance Registration System (GSRS) | |
URL | https://gsrs.ncats.nih.gov/ginas/app/beta/substances/SVI4RD590R | |
Description | The FDA Global Substance Registration System (GSRS) enables the efficient and accurate exchange of information on what substances are in regulated products. Instead of relying on names, which vary across regulatory domains, countries, and regions, the GSRS knowledge base makes it possible for substances to be defined by standardized, scientific descriptions. | |
Explanation | Unless otherwise noted, the contents of the FDA website (www.fda.gov), both text and graphics, are not copyrighted. They are in the public domain and may be republished, reprinted and otherwise used freely by anyone without the need to obtain permission from FDA. Credit to the U.S. Food and Drug Administration as the source is appreciated but not required. | |
Retrosynthesis Analysis
AI-Powered Synthesis Planning: Our tool employs the Template_relevance Pistachio, Template_relevance Bkms_metabolic, Template_relevance Pistachio_ringbreaker, Template_relevance Reaxys, Template_relevance Reaxys_biocatalysis model, leveraging a vast database of chemical reactions to predict feasible synthetic routes.
One-Step Synthesis Focus: Specifically designed for one-step synthesis, it provides concise and direct routes for your target compounds, streamlining the synthesis process.
Accurate Predictions: Utilizing the extensive PISTACHIO, BKMS_METABOLIC, PISTACHIO_RINGBREAKER, REAXYS, REAXYS_BIOCATALYSIS database, our tool offers high-accuracy predictions, reflecting the latest in chemical research and data.
Strategy Settings
Precursor scoring | Relevance Heuristic |
---|---|
Min. plausibility | 0.01 |
Model | Template_relevance |
Template Set | Pistachio/Bkms_metabolic/Pistachio_ringbreaker/Reaxys/Reaxys_biocatalysis |
Top-N result to add to graph | 6 |
Feasible Synthetic Routes
Disclaimer and Information on In-Vitro Research Products
Please be aware that all articles and product information presented on BenchChem are intended solely for informational purposes. The products available for purchase on BenchChem are specifically designed for in-vitro studies, which are conducted outside of living organisms. In-vitro studies, derived from the Latin term "in glass," involve experiments performed in controlled laboratory settings using cells or tissues. It is important to note that these products are not categorized as medicines or drugs, and they have not received approval from the FDA for the prevention, treatment, or cure of any medical condition, ailment, or disease. We must emphasize that any form of bodily introduction of these products into humans or animals is strictly prohibited by law. It is essential to adhere to these guidelines to ensure compliance with legal and ethical standards in research and experimentation.