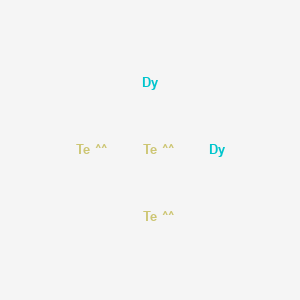
Dysprosium telluride
Overview
Description
Dysprosium telluride (DyTe) is a rare earth chalcogenide compound composed of dysprosium (Dy) and tellurium (Te). It exhibits a cubic crystal structure typical of metal tellurides and is notable for its stability under standard conditions .
Preparation Methods
Vapor Deposition Techniques
Vapor deposition stands as the most widely reported method for synthesizing dysprosium telluride, particularly for applications requiring thin films or quantum dots. This approach leverages controlled gas-phase reactions to deposit DyTe onto substrates, ensuring high purity and tailored morphologies.
Chemical Vapor Deposition (CVD)
CVD is a cornerstone technique for producing DyTe thin films with uniform thickness and minimal defects. In this process, dysprosium and tellurium precursors are vaporized in a high-temperature reactor, typically under inert atmospheres, and allowed to react on a heated substrate. Source highlights that DyTe photovoltaic cells prepared via vapor deposition exhibit high efficiency, attributed to the method’s ability to control stoichiometry and crystallite size . For instance, DyTe layers deposited at 600–800°C demonstrate optimal carrier mobility and photon absorption characteristics, critical for solar energy applications.
A key advantage of CVD is its scalability. By adjusting precursor flow rates and substrate temperatures, researchers can synthesize DyTe films over large areas, a necessity for industrial-scale production. Furthermore, the method’s compatibility with patterning techniques enables the fabrication of nanostructured DyTe for quantum-confined systems .
Physical Vapor Deposition (PVD)
PVD methods, including sputtering and thermal evaporation, are employed to produce DyTe coatings with precise thickness control. In sputtering, a dysprosium target is bombarded with argon ions in a tellurium-rich environment, leading to the co-deposition of Dy and Te atoms. This technique is favored for creating dense, adherent films suitable for optical coatings. Source notes that PVD-synthesized DyTe layers exhibit minimal oxygen contamination, a common challenge in rare-earth chalcogenide synthesis .
Solution-Phase Synthesis
While vapor deposition dominates DyTe production, solution-phase methods offer advantages in synthesizing nanostructured forms, such as quantum dots (QDs). These approaches enable fine control over particle size and surface functionalization.
Hydrothermal and Solvothermal Methods
Hydrothermal synthesis involves reacting dysprosium salts with tellurium precursors in aqueous or organic solvents at elevated temperatures (150–300°C) and pressures. This method is particularly effective for producing DyTe quantum dots, which require narrow size distributions for consistent optical properties. As reported in source , DyTe QDs synthesized hydrothermally exhibit emission spectra ranging from 490 nm to 740 nm, making them suitable for broadband light-emitting devices . The inclusion of capping agents, such as oleic acid, ensures colloidal stability and prevents aggregation.
Solvothermal methods, using non-aqueous solvents like ethylene glycol, further enhance crystallinity by slowing reaction kinetics. For example, DyTe nanorods synthesized in octadecene at 250°C demonstrate anisotropic growth along the direction, a feature advantageous for polarized optoelectronics .
Electrodeposition
Electrodeposition is a low-cost, energy-efficient method for depositing DyTe onto conductive substrates. By applying a controlled potential in an electrolyte containing Dy³⁺ and Te⁴⁻ ions, researchers can achieve layer-by-layer growth with sub-nanometer precision. Source emphasizes that electrodeposited DyTe films exhibit excellent adhesion and homogeneity, critical for electrode materials in energy storage systems . Adjusting the pH and electrolyte composition allows tuning of the Dy:Te ratio, enabling the synthesis of non-stoichiometric phases for specialized applications.
High-Pressure Solid-State Synthesis
High-pressure methods, though less common, enable the exploration of novel DyTe polymorphs with unique electronic properties. Using diamond anvil cells (DACs), dysprosium and tellurium are compressed to gigapascal-scale pressures and heated to promote diffusion-driven reactions. Source , while focused on dysprosium carbides, provides mechanistic insights relevant to telluride synthesis . For instance, at pressures above 40 GPa, rare-earth elements exhibit enhanced reactivity with chalcogens, suggesting that similar conditions could yield metastable DyTe phases.
Comparative Analysis of Synthesis Methods
The table below summarizes key parameters, advantages, and limitations of the primary DyTe preparation techniques:
Method | Temperature Range (°C) | Pressure Conditions | Morphology | Key Advantages | Limitations |
---|---|---|---|---|---|
Chemical Vapor Deposition | 600–800 | Atmospheric | Thin films, nanowires | High purity, scalability | High energy consumption |
Hydrothermal | 150–300 | Autogenous | Quantum dots, nanorods | Size control, low cost | Long reaction times |
Electrodeposition | 25–80 | Atmospheric | Uniform coatings | Energy efficiency, thickness control | Limited to conductive substrates |
High-Pressure DAC | 1000–2500 | 10–60 GPa | Bulk crystals | Access to metastable phases | Small sample volumes, high cost |
Scientific Research Applications
Magnetic Materials
Dysprosium telluride is primarily recognized for its role in enhancing the magnetic properties of materials. It is often used in the production of high-performance permanent magnets, particularly those based on neodymium-iron-boron (NdFeB). The addition of dysprosium improves the coercivity of these magnets, making them more effective at high temperatures.
Case Study: Coercivity Enhancement
Research has demonstrated that the incorporation of dysprosium into NdFeB magnets leads to significant improvements in coercivity. For instance, studies indicate that the formation of dysprosium-rich shells around the grains enhances the magnetic stability without compromising remanence. This is vital for applications in electric motors and generators where high-temperature performance is crucial .
Optical Applications
This compound also exhibits interesting optical properties, making it suitable for advanced optical applications. Its ability to absorb and emit light at specific wavelengths can be leveraged in various photonic devices.
Applications in Solar Energy
This compound is utilized in solar energy technologies, particularly in photovoltaic cells where it can enhance the efficiency of light absorption. The compound's unique electronic structure allows it to effectively convert sunlight into electricity, contributing to the development of more efficient solar panels .
Energy Storage and Conversion
Another significant application of this compound is in energy storage systems. Its properties make it a candidate for use as an electrode material in batteries and supercapacitors.
Research Findings
Recent studies have explored the use of this compound in nanostructured forms to improve charge storage capabilities. The compound's high conductivity and stability under various conditions make it an effective material for enhancing the performance of energy storage devices .
Thermoelectric Applications
This compound has potential applications in thermoelectric materials, which convert temperature differences into electrical voltage. This property can be harnessed for power generation from waste heat.
Performance Metrics
The thermoelectric efficiency of this compound has been analyzed through various performance metrics, such as Seebeck coefficient and thermal conductivity. These studies indicate that DyTe could be optimized for better performance in thermoelectric generators .
Summary Table: Applications of this compound
Application Area | Description | Key Benefits |
---|---|---|
Magnetic Materials | Enhances coercivity in NdFeB magnets | Improved high-temperature stability |
Optical Devices | Used in photovoltaic cells | Increased light absorption efficiency |
Energy Storage | Acts as an electrode material | Higher charge storage capacity |
Thermoelectric Devices | Converts temperature differences into electrical voltage | Potential for waste heat recovery |
Mechanism of Action
The mechanism by which dysprosium telluride exerts its effects is primarily related to its electronic structure. The large number of unpaired electrons in dysprosium’s 4-f electronic level allows for the formation of resonant levels, which can enhance the compound’s thermoelectric and magnetic properties. The interaction of localized magnetic moments with the spin of conduction electrons also plays a significant role in its functionality .
Comparison with Similar Compounds
Physical and Chemical Properties
- Chemical Stability: DyTe is non-explosive, non-oxidizing, and stable under normal handling conditions. However, it reacts with strong oxidizers, necessitating careful storage .
- Thermal Properties : While decomposition temperature data are unavailable, DyTe demonstrates stability in thermoelectric applications up to 200°C .
- Hazard Profile: Prolonged inhalation poses health risks, but skin/eye contact causes only temporary irritation. No significant reproductive toxicity or environmental hazards are reported .
Rare earth and transition metal tellurides share structural similarities but differ significantly in functional properties. Below is a detailed comparison:
Optical Properties
DyTe’s optical constants are less documented compared to widely studied tellurides like cadmium telluride (CdTe) and lead telluride (PbTe) . The Handbook of Optical Constants of Solids highlights CdTe and PbTe for their well-characterized infrared transparency and photoconductive properties, which are critical in solar cells and detectors . DyTe’s niche application in infrared sources suggests narrower optical utility .
Thermoelectric Performance
DyTe-doped systems (e.g., Gd₁₋ₓDyₓCuTe₂) show reduced thermal conductivity and enhanced electrical conductivity compared to undoped GdCuTe₂, achieving a ZT of ~0.45 at x = 0.05 . However, PbTe remains superior due to its optimized carrier mobility and low lattice thermal conductivity .
Stability and Reactivity
Biological Activity
Dysprosium telluride (DyTe) is a compound of interest due to its unique properties and potential applications in various fields, including materials science and biomedicine. This article reviews the biological activity of this compound, focusing on its interactions with biological systems, potential toxicity, and implications for health.
This compound is a semiconductor material that exhibits interesting electrical and optical properties. The compound is typically synthesized through solid-state reactions or chemical vapor deposition methods. Its crystal structure is characterized by the presence of dysprosium ions coordinated with tellurium atoms, forming octahedral geometries that contribute to its electronic properties .
Biological Activity Overview
The biological activity of this compound is not extensively documented in literature, but some studies have explored the effects of its constituent elements—dysprosium and tellurium—on biological systems. Understanding these effects is crucial for assessing the safety and potential therapeutic uses of DyTe.
2. Interaction with Biological Systems
Research indicates that dysprosium ions can interact with various biological molecules, potentially influencing enzymatic activities. For example, dysprosium has been shown to inhibit certain enzymes involved in metabolic pathways, which could have implications for drug development . However, specific studies focusing on this compound's interactions within biological systems remain sparse.
Case Study 1: Aquatic Toxicity
A study evaluated the effects of exposure to tellurium compounds on fish species, revealing significant mortality rates at concentrations exceeding . The findings suggest that compounds containing tellurium, including those derived from this compound, could pose risks to aquatic life if released into ecosystems .
Case Study 2: Enzymatic Inhibition
In vitro studies have demonstrated that rare earth elements can inhibit key enzymes involved in neurotransmitter synthesis. Although direct studies on this compound are lacking, the potential for similar inhibitory effects exists due to the presence of dysprosium ions .
Data Table: Summary of Biological Effects
Compound | Biological Activity | Toxicity Level | Affected Organisms |
---|---|---|---|
Dysprosium | Enzyme inhibition | Moderate | Mammalian cells |
Tellurium | Oxidative stress | High | Aquatic organisms |
This compound | Unknown | Unknown | Not directly studied |
Chemical Reactions Analysis
Reactions with Other Tellurides
Dysprosium telluride exhibits reactivity with transition-metal tellurides, forming complex ternary phases:
Reaction with Copper(II) Telluride
At high temperatures (~800–1000°C), Dy₂Te₃ reacts with CuTe to produce three distinct phases :
The specific product depends on the molar ratio of reactants and reaction duration.
Reaction with Cadmium Telluride
Dy₂Te₃ combines with CdTe under similar conditions to form CdDy₂Te₄ :
This reaction highlights Dy₂Te₃’s ability to incorporate divalent metals into its lattice structure.
Stability and Decomposition
This compound is stable under inert conditions but decomposes in oxidizing environments. While direct decomposition pathways are less documented, analogous lanthanide tellurides decompose into oxides and elemental tellurium at high temperatures in air:
This reaction is inferred from the behavior of similar compounds .
Redox Behavior
Dy₂Te₃ participates in redox reactions due to the variable oxidation states of tellurium (-2 to +6). For example, in acidic solutions, it may act as a reducing agent:
Hydrogen telluride (H₂Te) is highly unstable and rapidly decomposes into tellurium and hydrogen gas .
Data Table: Key Reactions of this compound
Mechanistic Insights
-
Solid-State Reactions : The formation of ternary phases (e.g., DyCuTe₂) involves diffusion-controlled processes where dysprosium and copper ions migrate into each other’s lattices .
-
Electronic Effects : Dysprosium’s 4f electrons influence the compound’s redox behavior, stabilizing tellurium in lower oxidation states during reactions .
Properties
InChI |
InChI=1S/2Dy.3Te | |
---|---|---|
Source | PubChem | |
URL | https://pubchem.ncbi.nlm.nih.gov | |
Description | Data deposited in or computed by PubChem | |
InChI Key |
ISSYQCZLWJTXOM-UHFFFAOYSA-N | |
Source | PubChem | |
URL | https://pubchem.ncbi.nlm.nih.gov | |
Description | Data deposited in or computed by PubChem | |
Canonical SMILES |
[Te].[Te].[Te].[Dy].[Dy] | |
Source | PubChem | |
URL | https://pubchem.ncbi.nlm.nih.gov | |
Description | Data deposited in or computed by PubChem | |
Molecular Formula |
Dy2Te3 | |
Source | PubChem | |
URL | https://pubchem.ncbi.nlm.nih.gov | |
Description | Data deposited in or computed by PubChem | |
Molecular Weight |
707.8 g/mol | |
Source | PubChem | |
URL | https://pubchem.ncbi.nlm.nih.gov | |
Description | Data deposited in or computed by PubChem | |
CAS No. |
12159-43-2 | |
Record name | Dysprosium telluride (Dy2Te3) | |
Source | ChemIDplus | |
URL | https://pubchem.ncbi.nlm.nih.gov/substance/?source=chemidplus&sourceid=0012159432 | |
Description | ChemIDplus is a free, web search system that provides access to the structure and nomenclature authority files used for the identification of chemical substances cited in National Library of Medicine (NLM) databases, including the TOXNET system. | |
Record name | Dysprosium telluride (Dy2Te3) | |
Source | EPA Chemicals under the TSCA | |
URL | https://www.epa.gov/chemicals-under-tsca | |
Description | EPA Chemicals under the Toxic Substances Control Act (TSCA) collection contains information on chemicals and their regulations under TSCA, including non-confidential content from the TSCA Chemical Substance Inventory and Chemical Data Reporting. | |
Record name | Didysprosium tritelluride | |
Source | European Chemicals Agency (ECHA) | |
URL | https://echa.europa.eu/substance-information/-/substanceinfo/100.032.068 | |
Description | The European Chemicals Agency (ECHA) is an agency of the European Union which is the driving force among regulatory authorities in implementing the EU's groundbreaking chemicals legislation for the benefit of human health and the environment as well as for innovation and competitiveness. | |
Explanation | Use of the information, documents and data from the ECHA website is subject to the terms and conditions of this Legal Notice, and subject to other binding limitations provided for under applicable law, the information, documents and data made available on the ECHA website may be reproduced, distributed and/or used, totally or in part, for non-commercial purposes provided that ECHA is acknowledged as the source: "Source: European Chemicals Agency, http://echa.europa.eu/". Such acknowledgement must be included in each copy of the material. ECHA permits and encourages organisations and individuals to create links to the ECHA website under the following cumulative conditions: Links can only be made to webpages that provide a link to the Legal Notice page. | |
Disclaimer and Information on In-Vitro Research Products
Please be aware that all articles and product information presented on BenchChem are intended solely for informational purposes. The products available for purchase on BenchChem are specifically designed for in-vitro studies, which are conducted outside of living organisms. In-vitro studies, derived from the Latin term "in glass," involve experiments performed in controlled laboratory settings using cells or tissues. It is important to note that these products are not categorized as medicines or drugs, and they have not received approval from the FDA for the prevention, treatment, or cure of any medical condition, ailment, or disease. We must emphasize that any form of bodily introduction of these products into humans or animals is strictly prohibited by law. It is essential to adhere to these guidelines to ensure compliance with legal and ethical standards in research and experimentation.