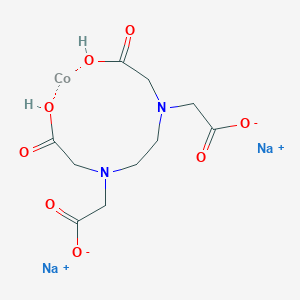
Cobalt disodium ethylenediaminetetraacetate
Overview
Description
Cobalt disodium ethylenediaminetetraacetate is a compound where cobalt(2+) ions are bound to the ethylenediaminetetraacetate (EDTA) molecule . It’s a variant of EDTA where cobalt replaces the hydrogen atoms .
Chemical Reactions Analysis
In an electrochemical investigation, it was found that the addition of EDTA to a chloride electrolyte significantly shifts the reduction reaction potential of cobalt in a more negative direction while slightly altering the discharging potential of manganese . This is crucial in promoting the deposition of the Co-Mn alloy .Scientific Research Applications
Application 1: Electrochemical Investigation
- Summary of Application: Cobalt disodium ethylenediaminetetraacetate is used in the electrodeposition process of cobalt-manganese (Co-Mn) alloys. These alloys are potential protective and conductive coatings on ferritic stainless steel interconnects in solid oxide fuel cells .
- Methods of Application: The addition of EDTA to the chloride electrolyte significantly shifts the reduction reaction potential of cobalt in a more negative direction while slightly altering the discharging potential of manganese. It’s crucial to control the [Co2+]/[EDTA] ratio to form completely complexed cobalt ions and a very small amount of complexed manganese ions, thus promoting the deposition of the Co-Mn alloy .
- Results or Outcomes: The addition of EDTA to the chloride electrolyte is a promising way to prepare a Co-Mn alloy coating via electrodeposition .
Application 2: Separation Mechanisms of Co(III) Complexes
- Summary of Application: Cobalt disodium ethylenediaminetetraacetate is used in the separation mechanism of Co(III) complexes during salting-out thin-layer chromatography on silica gel .
- Methods of Application: The sorbent is impregnated with five poly (ethylene glycol)s with different molecular masses, and ammonium sulphate solutions are used as mobile phase .
- Results or Outcomes: Salting-out and salting-in effects were observed depending on salt nature. The combined retention mechanism of specific H-bonding and nonspecific hydrophobic interactions is proposed .
Application 3: Textiles and Paper Industry
- Summary of Application: In the textile industry, Cobalt disodium ethylenediaminetetraacetate is used to sequester (bind or confine) metal ions in aqueous solution. It prevents metal ion impurities from modifying colors of dyed products .
- Methods of Application: The EDTA binds to metal ions in the solution, forming water-soluble complexes even at neutral pH. This process helps to remove metal ion impurities that could otherwise interfere with the dyeing process .
- Results or Outcomes: The use of EDTA in the textile industry helps to maintain the integrity of the colors in dyed products .
Application 4: Nuclear Reactors
- Summary of Application: Cobalt disodium ethylenediaminetetraacetate is used to remove crud (corroded metals) from fuel rods in nuclear reactors .
- Methods of Application: The EDTA is used to bind to iron (Fe 2+ /Fe 3+) and calcium ions (Ca 2+ ), forming water-soluble complexes. This process helps to dissolve Fe- and Ca-containing scale .
- Results or Outcomes: The use of EDTA in nuclear reactors helps to maintain the efficiency and safety of the reactor by removing crud from the fuel rods .
Application 5: Laboratory Applications
- Summary of Application: In the laboratory, EDTA is widely used for scavenging metal ions. It is commonly used to deactivate metal-dependent enzymes, either as an assay for their reactivity or to suppress damage to DNA, proteins, and polysaccharides .
- Methods of Application: The EDTA binds to metal ions in the solution, forming water-soluble complexes even at neutral pH. This process helps to remove metal ion impurities that could otherwise interfere with the biochemical reactions .
- Results or Outcomes: The use of EDTA in the laboratory helps to maintain the integrity of biochemical reactions by removing metal ion impurities .
Application 6: Environmental Concerns
- Summary of Application: EDTA is used in environmental remediation to mobilize heavy metals in the soil. It forms water-soluble complexes with heavy metals, which can then be removed from the soil .
- Methods of Application: The EDTA is applied to the soil, where it binds to heavy metals, forming water-soluble complexes. These complexes can then be washed out of the soil .
- Results or Outcomes: The use of EDTA in environmental remediation helps to remove heavy metals from contaminated soils, improving the quality of the soil .
Future Directions
properties
IUPAC Name |
disodium;2-[2-[carboxylatomethyl(carboxymethyl)amino]ethyl-(carboxymethyl)amino]acetate;cobalt | |
---|---|---|
Source | PubChem | |
URL | https://pubchem.ncbi.nlm.nih.gov | |
Description | Data deposited in or computed by PubChem | |
InChI |
InChI=1S/C10H16N2O8.Co.2Na/c13-7(14)3-11(4-8(15)16)1-2-12(5-9(17)18)6-10(19)20;;;/h1-6H2,(H,13,14)(H,15,16)(H,17,18)(H,19,20);;;/q;;2*+1/p-2 | |
Source | PubChem | |
URL | https://pubchem.ncbi.nlm.nih.gov | |
Description | Data deposited in or computed by PubChem | |
InChI Key |
ULJJVEZLQWBJLT-UHFFFAOYSA-L | |
Source | PubChem | |
URL | https://pubchem.ncbi.nlm.nih.gov | |
Description | Data deposited in or computed by PubChem | |
Canonical SMILES |
C(CN(CC(=O)O)CC(=O)[O-])N(CC(=O)O)CC(=O)[O-].[Na+].[Na+].[Co] | |
Source | PubChem | |
URL | https://pubchem.ncbi.nlm.nih.gov | |
Description | Data deposited in or computed by PubChem | |
Molecular Formula |
C10H14CoN2Na2O8 | |
Source | PubChem | |
URL | https://pubchem.ncbi.nlm.nih.gov | |
Description | Data deposited in or computed by PubChem | |
Related CAS |
14931-83-0 (Parent) | |
Record name | Disodium cobalt EDTA | |
Source | ChemIDplus | |
URL | https://pubchem.ncbi.nlm.nih.gov/substance/?source=chemidplus&sourceid=0015137094 | |
Description | ChemIDplus is a free, web search system that provides access to the structure and nomenclature authority files used for the identification of chemical substances cited in National Library of Medicine (NLM) databases, including the TOXNET system. | |
Molecular Weight |
395.14 g/mol | |
Source | PubChem | |
URL | https://pubchem.ncbi.nlm.nih.gov | |
Description | Data deposited in or computed by PubChem | |
Product Name |
Disodium cobalt edetate | |
CAS RN |
15137-09-4 | |
Record name | Disodium cobalt EDTA | |
Source | ChemIDplus | |
URL | https://pubchem.ncbi.nlm.nih.gov/substance/?source=chemidplus&sourceid=0015137094 | |
Description | ChemIDplus is a free, web search system that provides access to the structure and nomenclature authority files used for the identification of chemical substances cited in National Library of Medicine (NLM) databases, including the TOXNET system. | |
Record name | Cobalt disodium ethylenediaminetetraacetate | |
Source | European Chemicals Agency (ECHA) | |
URL | https://echa.europa.eu/substance-information/-/substanceinfo/100.035.620 | |
Description | The European Chemicals Agency (ECHA) is an agency of the European Union which is the driving force among regulatory authorities in implementing the EU's groundbreaking chemicals legislation for the benefit of human health and the environment as well as for innovation and competitiveness. | |
Explanation | Use of the information, documents and data from the ECHA website is subject to the terms and conditions of this Legal Notice, and subject to other binding limitations provided for under applicable law, the information, documents and data made available on the ECHA website may be reproduced, distributed and/or used, totally or in part, for non-commercial purposes provided that ECHA is acknowledged as the source: "Source: European Chemicals Agency, http://echa.europa.eu/". Such acknowledgement must be included in each copy of the material. ECHA permits and encourages organisations and individuals to create links to the ECHA website under the following cumulative conditions: Links can only be made to webpages that provide a link to the Legal Notice page. | |
Retrosynthesis Analysis
AI-Powered Synthesis Planning: Our tool employs the Template_relevance Pistachio, Template_relevance Bkms_metabolic, Template_relevance Pistachio_ringbreaker, Template_relevance Reaxys, Template_relevance Reaxys_biocatalysis model, leveraging a vast database of chemical reactions to predict feasible synthetic routes.
One-Step Synthesis Focus: Specifically designed for one-step synthesis, it provides concise and direct routes for your target compounds, streamlining the synthesis process.
Accurate Predictions: Utilizing the extensive PISTACHIO, BKMS_METABOLIC, PISTACHIO_RINGBREAKER, REAXYS, REAXYS_BIOCATALYSIS database, our tool offers high-accuracy predictions, reflecting the latest in chemical research and data.
Strategy Settings
Precursor scoring | Relevance Heuristic |
---|---|
Min. plausibility | 0.01 |
Model | Template_relevance |
Template Set | Pistachio/Bkms_metabolic/Pistachio_ringbreaker/Reaxys/Reaxys_biocatalysis |
Top-N result to add to graph | 6 |
Feasible Synthetic Routes
Citations
Disclaimer and Information on In-Vitro Research Products
Please be aware that all articles and product information presented on BenchChem are intended solely for informational purposes. The products available for purchase on BenchChem are specifically designed for in-vitro studies, which are conducted outside of living organisms. In-vitro studies, derived from the Latin term "in glass," involve experiments performed in controlled laboratory settings using cells or tissues. It is important to note that these products are not categorized as medicines or drugs, and they have not received approval from the FDA for the prevention, treatment, or cure of any medical condition, ailment, or disease. We must emphasize that any form of bodily introduction of these products into humans or animals is strictly prohibited by law. It is essential to adhere to these guidelines to ensure compliance with legal and ethical standards in research and experimentation.