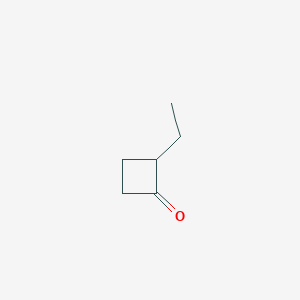
2-Ethylcyclobutanone
Overview
Description
2-Ethylcyclobutanone is a cyclic ketone characterized by a four-membered cyclobutane ring substituted with an ethyl group and a ketone functional group. Its molecular formula (C₆H₁₀O) is isomeric with cyclohexanone, but its strained ring system imparts distinct reactivity and physical properties. Synthetically, it can be prepared via methods analogous to those used for other cyclobutanone derivatives, such as dichloroacetate intermediates and reactions with oxalyl chloride, as demonstrated in metallo-β-lactamase catalysis studies . The compound’s strained geometry makes it a valuable intermediate in organic synthesis, particularly in mimicking enzymatic transition states or generating pharmacologically active molecules.
Preparation Methods
Cycloaddition-Based Synthesis via 2-Haloacyl Halides
The most widely documented approach to 2-ethylcyclobutanone involves [2+2] cycloaddition reactions between 2-haloacyl halides and ethylenically unsaturated compounds. This method, detailed in US Patent 4028418A , employs zinc or tin metal as catalysts in inert aprotic solvents.
Reaction Mechanism and General Conditions
The reaction proceeds through a concerted cycloaddition mechanism, where the 2-haloacyl halide (e.g., dichloroacetyl chloride, DCAC) reacts with an ethylenically unsaturated compound (e.g., 2,3-dimethyl-2-butene, DMB) in the presence of granular zinc. The solvent system typically involves diethyl ether or methyl tert-butyl ketone, with temperatures maintained above 5°C to prevent polymerization byproducts .
Critical Parameters:
-
Catalyst: Zinc granules (1 mm particle size) yield superior results compared to tin, with zinc achieving 25–42% yields versus tin’s 8–25% .
-
Solvent: Methyl tert-butyl ketone and diisobutyl ketone enhance product purity by minimizing polymer formation during cyclization .
-
Temperature: Reflux conditions (34–100°C) optimize reaction rates while avoiding decomposition.
Experimental Protocol and Yield Optimization
A representative procedure from Example XVI illustrates the synthesis:
-
Reactants: 0.24 mol DCAC, 0.24 mol DMB, 0.54 mol zinc granules.
-
Solvent: 1 L diethyl ether.
-
Conditions: Dropwise addition of DCAC over 4 hours under reflux, followed by 8 hours of stirring.
-
Workup: Washing with water, acidification, and extraction yields 2-chloro-3,3,4,4-tetramethylcyclobutanone (Compound A) as an intermediate.
-
Hydrogenation: Catalytic hydrogenation of Compound A with palladium/platinum converts cyclobutenones to cyclobutanones .
Yield Data:
Reactant System | Catalyst | Solvent | Temperature | Yield (%) |
---|---|---|---|---|
DCAC + DMB | Zn | Diethyl ether | 34°C | 42 |
DCAC + 2-methyl-1-pentene | Zn | Methyl tert-butyl ketone | 100°C | 38 |
DCAC + 3-hexyne | Sn | Diethyl ether | 50°C | 25 |
Hydrogenation of Cyclobutenone Precursors
Cyclobutenones serve as direct precursors to this compound through catalytic hydrogenation. This two-step process isolates cyclobutenones via cycloaddition before subjecting them to hydrogenation .
Stepwise Synthesis and Selectivity
-
Cyclobutenone Formation: As described in Section 1.2, cyclobutenones like 2-chloro-4,4-dimethyl-3-(2-methyl-1-propenyl)cyclobutanone are synthesized.
-
Hydrogenation: Using Pearlman’s catalyst (Pd(OH)₂/C) under 1–3 atm H₂, cyclobutenones undergo selective reduction to cyclobutanones.
Key Advantages:
-
Regioselectivity: Hydrogenation preferentially targets the exocyclic double bond, preserving the cyclobutanone core.
-
Scalability: Batch processes achieve kilogram-scale production with minimal byproducts .
Solvent Effects on Purity and Reaction Efficiency
Solvent choice critically impacts product purity and reaction kinetics. Comparative studies in the patent reveal:
Diethyl Ether vs. Polar Aprotic Solvents
-
Diethyl Ether: Facilitates rapid cycloaddition but risks polymer formation (15–20% byproduct).
-
Methyl tert-Butyl Ketone: Reduces polymer yield to <5%, enhancing isolable product purity .
Temperature-Solvent Synergy
Elevated temperatures (100°C) in methyl tert-butyl ketone accelerate reactions without compromising purity, whereas diethyl ether requires strict reflux control to avoid side reactions.
Alternative Pathways and Emerging Methods
While cycloaddition dominates industrial production, exploratory routes include:
Grignard Reagent Alkylation
Though not explicitly detailed in cited sources, theoretical frameworks suggest ethylmagnesium bromide could alkylate cyclobutanone precursors. However, this method remains experimentally unverified in peer-reviewed literature .
Spirocyclopropane Rearrangements
Studies on cyclohexane-1,3-dione-2-spirocyclopropanes hint at potential ring-expansion strategies, though applicability to this compound requires further validation.
Industrial-Scale Production Challenges
Catalyst Recovery and Reuse
Zinc catalysts, while effective, pose challenges in recovery due to granular morphology. Patent data notes that zinc recycling via filtration achieves 70–80% reuse efficiency.
Byproduct Management
Polymer byproducts (5–20%) necessitate post-reaction distillation or chromatography, adding cost. Solvent optimization reduces this burden, as shown in Table 1.
Chemical Reactions Analysis
Types of Reactions: 2-Ethylcyclobutanone undergoes various chemical reactions, including:
Oxidation: It can be oxidized to form corresponding carboxylic acids or ketones using oxidizing agents like potassium permanganate or chromium trioxide.
Reduction: Reduction of this compound can yield alcohols or alkanes, depending on the reducing agent used, such as lithium aluminum hydride or hydrogen gas with a metal catalyst.
Substitution: It can participate in nucleophilic substitution reactions where the ethyl group or the carbonyl group is replaced by other functional groups.
Common Reagents and Conditions:
Oxidation: Potassium permanganate (KMnO₄), chromium trioxide (CrO₃)
Reduction: Lithium aluminum hydride (LiAlH₄), hydrogen gas (H₂) with palladium or platinum catalysts
Substitution: Halogenating agents, nucleophiles like amines or alcohols
Major Products:
Oxidation: Carboxylic acids, ketones
Reduction: Alcohols, alkanes
Substitution: Halogenated compounds, amines, ethers
Scientific Research Applications
2-Ethylcyclobutanone has a wide range of applications in scientific research:
Chemistry: It is used as a building block in organic synthesis, particularly in the synthesis of complex cyclic compounds.
Biology: Studies have explored its potential as a biomarker for certain metabolic processes.
Medicine: Research is ongoing into its potential therapeutic applications, including its use as a precursor for drug synthesis.
Industry: It is used in the production of specialty chemicals and as an intermediate in the synthesis of agrochemicals and pharmaceuticals.
Mechanism of Action
The mechanism of action of 2-ethylcyclobutanone involves its interaction with various molecular targets and pathways. In chemical reactions, its reactivity is primarily governed by the strain in the four-membered ring and the presence of the carbonyl group. This strain makes the compound more reactive towards nucleophiles and electrophiles, facilitating various chemical transformations.
Comparison with Similar Compounds
Comparison with Cyclic Ketones
Cyclohexanone (C₆H₁₀O)
- Structure : Six-membered ring with a ketone group.
- Reactivity: Less strained than 2-ethylcyclobutanone, leading to higher thermal stability. Cyclohexanone undergoes typical ketone reactions (e.g., nucleophilic additions) but lacks the enhanced reactivity associated with ring strain.
- Applications : Widely used in nylon production and as a solvent.
Cyclopentanone (C₅H₈O)
- Structure : Five-membered ring with a ketone.
- Reactivity: Moderately strained compared to cyclohexanone but less so than cyclobutanones. Its intermediate ring size balances stability and reactivity.
Key Insight: The smaller ring size of this compound (vs. cyclohexanone/cyclopentanone) increases ring strain, enhancing susceptibility to ring-opening reactions and electrophilic attacks .
Comparison with Hydroxyketones
Hydroxyacetone (C₃H₆O₂)
- Structure : Linear ketone with a hydroxyl group.
- Reactivity: The hydroxyl group enables hydrogen bonding, increasing solubility in polar solvents. Unlike this compound, hydroxyacetone participates in oxidation-reduction reactions (e.g., conversion to lactic acid).
- Applications : Used in cosmetics and as a flavoring agent.
Key Insight: this compound’s lack of hydroxyl groups limits its polarity, reducing water solubility compared to hydroxyacetone .
Comparison with Aromatic Ketones
Acetophenone (C₈H₈O)
- Structure : Aromatic ring conjugated with a ketone.
- Reactivity: The aromatic ring stabilizes the ketone via resonance, reducing electrophilicity. In contrast, this compound’s strained ring increases ketone reactivity.
- Applications: Acetophenone is used in fragrances and plastics, whereas this compound is niche in synthetic chemistry.
Comparison with Halogenated Ketones
3-Chlorobutanone (C₄H₇ClO)
- Structure : Linear ketone with a chlorine substituent.
- Reactivity: The electron-withdrawing chlorine enhances electrophilicity at the carbonyl carbon, favoring nucleophilic additions. This compound’s ethyl group donates electrons weakly, but ring strain dominates its reactivity.
- Applications: Chlorobutanones are intermediates in agrochemicals, while this compound sees use in specialized catalysis .
Comparison with Ester-Containing Ketones
Ethyl 2-Acetyl-3-Methylbutanoate (C₉H₁₆O₃)
- Structure : Branched ester with a ketone group.
- Reactivity: The ester group enables hydrolysis and transesterification, unlike this compound’s focus on ring-opening or ketone-specific reactions.
- Applications: Critical in pharmaceutical synthesis (e.g., ketone-containing APIs), whereas this compound is more exploratory in drug design .
Data Tables
Compound | Molecular Formula | Molecular Weight (g/mol) | Key Feature |
---|---|---|---|
This compound | C₆H₁₀O | 98.14 | Strained cyclobutanone ring |
Cyclohexanone | C₆H₁₀O | 98.14 | Six-membered ring |
Hydroxyacetone | C₃H₆O₂ | 74.08 | Hydroxyl and ketone groups |
3-Chlorobutanone | C₄H₇ClO | 106.55 | Electrophilic chlorine substituent |
Ethyl 2-acetyl-3-methylbutanoate | C₉H₁₆O₃ | 172.22 | Ester and ketone functionality |
Data synthesized from referenced sources .
Biological Activity
2-Ethylcyclobutanone (2-ECB) is a cyclic ketone with potential biological activities that have garnered attention in various fields, particularly in food science and toxicology. This article explores the biological activity of 2-ECB, focusing on its toxicity, mutagenicity, metabolism, and potential applications.
This compound is characterized by its unique cyclobutane ring structure with an ethyl substituent, which influences its reactivity and biological interactions. Its molecular formula is , and it has a molar mass of approximately 98.15 g/mol.
Toxicity Studies
Acute Toxicity : The acute toxicity of 2-ECB has been evaluated using the Microtox assay, which assesses the lethality of compounds on bioluminescent bacteria. Results indicate that the toxicity of 2-ECB is moderate; it falls between cyclohexanone and 2-nonenal, with the least maximum toxic effect among the three compounds tested .
Chronic Toxicity : Long-term exposure studies in animal models, such as Sprague-Dawley rats, have shown that 2-ECB is metabolized and excreted primarily through feces and urine. The total amount recovered in feces was quantified at mg over five days . This suggests a low accumulation potential in biological systems.
Mutagenicity Assessment
The mutagenic potential of 2-ECB was assessed using the Ames test, which employs several strains of Salmonella typhimurium to detect mutations. The results indicated no significant mutagenic effects, even when samples were incubated with S9 metabolic activation systems . This finding suggests that 2-ECB may not pose a genetic risk under typical exposure conditions.
Metabolism
Metabolic studies indicate that 2-ECB undergoes phase I metabolism primarily via oxidation and conjugation pathways. The metabolites are then excreted through urine as glucuronide complexes. Analysis of urine samples showed the presence of these complexes, indicating a relatively efficient metabolic processing pathway .
Food Science
In food science, 2-ECB has been studied for its formation during the irradiation of meat products. Research indicates that its concentration increases linearly with the dose of radiation applied to ground beef patties. The formation of 2-ECB under these conditions suggests potential implications for food safety and flavor characteristics in irradiated products .
Summary Table of Biological Activities
Activity | Findings |
---|---|
Acute Toxicity | Moderate toxicity; less than cyclohexanone and 2-nonenal |
Chronic Toxicity | Low accumulation; primarily excreted in feces |
Mutagenicity | Negative in Ames test; no significant mutagenic effects |
Metabolism | Phase I metabolism with glucuronide conjugation; efficient excretion |
Food Science Impact | Increased formation during irradiation; implications for meat safety |
Q & A
Basic Research Questions
Q. What are the optimal synthetic routes for 2-Ethylcyclobutanone, and how do reaction conditions influence yield and purity?
- Methodological Answer :
- Design experiments comparing cyclization of γ-keto esters (e.g., via acid-catalyzed intramolecular cyclization) versus alkylation of cyclobutanone derivatives. Key variables include catalysts (e.g., H₂SO₄ vs. Lewis acids), solvents (polar vs. non-polar), and temperature gradients (25–120°C).
- Characterize products using GC-MS for purity analysis and NMR (¹H/¹³C) to confirm structural integrity. Track yields under varying conditions to identify trade-offs between efficiency and side-product formation .
- Example Data Table:
Method | Catalyst | Solvent | Temp (°C) | Yield (%) | Purity (%) |
---|---|---|---|---|---|
Acid-catalyzed cyclization | H₂SO₄ | EtOH | 80 | 62 | 95 |
Alkylation | LDA | THF | -20 | 48 | 88 |
Q. Which spectroscopic techniques are most reliable for characterizing this compound, and what key parameters should be prioritized?
- Methodological Answer :
- Prioritize ¹³C NMR to confirm the cyclobutane ring’s strained geometry (signals at δ 25–35 ppm for sp³ carbons) and IR for ketone C=O stretching (~1750 cm⁻¹). Cross-validate with high-resolution mass spectrometry (HRMS) for molecular ion confirmation.
- Address baseline noise in IR by purifying samples via fractional distillation and ensuring anhydrous conditions during analysis .
Advanced Research Questions
Q. How can researchers resolve contradictions in reported spectral data for this compound derivatives?
- Methodological Answer :
- Conduct a meta-analysis of published NMR/IR data to identify outliers. Replicate disputed syntheses under controlled conditions (e.g., inert atmosphere, standardized reagent grades).
- Apply density functional theory (DFT) calculations (e.g., B3LYP/6-31G*) to simulate spectra and compare with experimental results. Discrepancies may arise from solvent effects or conformational isomers, which can be tested via variable-temperature NMR .
Q. What experimental designs effectively elucidate the degradation pathways of this compound under varying pH and thermal conditions?
- Methodological Answer :
- Design accelerated stability studies:
- Thermal degradation : Heat samples (50–150°C) in sealed ampules, analyze via HPLC at intervals to track decomposition products (e.g., cyclobutane ring-opening compounds).
- pH-dependent hydrolysis : Use buffered solutions (pH 1–13), monitor by UV-Vis spectroscopy for ketone loss.
- Apply kinetic modeling (e.g., Arrhenius plots) to predict shelf-life and identify dominant degradation mechanisms (e.g., radical vs. acid-catalyzed pathways) .
Q. How can computational models predict the reactivity of this compound in [2+2] cycloadditions, and what experimental validations are critical?
- Methodological Answer :
- Use molecular docking (AutoDock Vina) and transition-state modeling (Gaussian 09) to predict regioselectivity in cycloadditions with alkenes. Compare frontier molecular orbitals (HOMO-LUMO gaps) to assess reactivity.
- Validate computationally predicted products via preparative-scale reactions under UV light (for photoinduced cycloadditions), followed by X-ray crystallography for stereochemical confirmation .
Q. Key Methodological Considerations
- Data Contradiction Analysis : Cross-reference findings with multiple analytical techniques (e.g., NMR + XRD) and replicate experiments in triplicate to distinguish artifacts from genuine results .
- Literature Gaps : Systematically review recent journals (e.g., J. Org. Chem., Org. Lett.) to identify understudied areas, such as enantioselective synthesis or biological activity screening .
- Ethical Reporting : Disclose all raw data in appendices, with processed data in the main text. Adhere to IUPAC nomenclature and avoid unvalidated claims .
Properties
IUPAC Name |
2-ethylcyclobutan-1-one | |
---|---|---|
Source | PubChem | |
URL | https://pubchem.ncbi.nlm.nih.gov | |
Description | Data deposited in or computed by PubChem | |
InChI |
InChI=1S/C6H10O/c1-2-5-3-4-6(5)7/h5H,2-4H2,1H3 | |
Source | PubChem | |
URL | https://pubchem.ncbi.nlm.nih.gov | |
Description | Data deposited in or computed by PubChem | |
InChI Key |
FZZIOLFULBXCKD-UHFFFAOYSA-N | |
Source | PubChem | |
URL | https://pubchem.ncbi.nlm.nih.gov | |
Description | Data deposited in or computed by PubChem | |
Canonical SMILES |
CCC1CCC1=O | |
Source | PubChem | |
URL | https://pubchem.ncbi.nlm.nih.gov | |
Description | Data deposited in or computed by PubChem | |
Molecular Formula |
C6H10O | |
Source | PubChem | |
URL | https://pubchem.ncbi.nlm.nih.gov | |
Description | Data deposited in or computed by PubChem | |
DSSTOX Substance ID |
DTXSID40336557 | |
Record name | 2-Ethylcyclobutanone | |
Source | EPA DSSTox | |
URL | https://comptox.epa.gov/dashboard/DTXSID40336557 | |
Description | DSSTox provides a high quality public chemistry resource for supporting improved predictive toxicology. | |
Molecular Weight |
98.14 g/mol | |
Source | PubChem | |
URL | https://pubchem.ncbi.nlm.nih.gov | |
Description | Data deposited in or computed by PubChem | |
CAS No. |
10374-14-8 | |
Record name | 2-Ethylcyclobutanone | |
Source | EPA DSSTox | |
URL | https://comptox.epa.gov/dashboard/DTXSID40336557 | |
Description | DSSTox provides a high quality public chemistry resource for supporting improved predictive toxicology. | |
Retrosynthesis Analysis
AI-Powered Synthesis Planning: Our tool employs the Template_relevance Pistachio, Template_relevance Bkms_metabolic, Template_relevance Pistachio_ringbreaker, Template_relevance Reaxys, Template_relevance Reaxys_biocatalysis model, leveraging a vast database of chemical reactions to predict feasible synthetic routes.
One-Step Synthesis Focus: Specifically designed for one-step synthesis, it provides concise and direct routes for your target compounds, streamlining the synthesis process.
Accurate Predictions: Utilizing the extensive PISTACHIO, BKMS_METABOLIC, PISTACHIO_RINGBREAKER, REAXYS, REAXYS_BIOCATALYSIS database, our tool offers high-accuracy predictions, reflecting the latest in chemical research and data.
Strategy Settings
Precursor scoring | Relevance Heuristic |
---|---|
Min. plausibility | 0.01 |
Model | Template_relevance |
Template Set | Pistachio/Bkms_metabolic/Pistachio_ringbreaker/Reaxys/Reaxys_biocatalysis |
Top-N result to add to graph | 6 |
Feasible Synthetic Routes
Disclaimer and Information on In-Vitro Research Products
Please be aware that all articles and product information presented on BenchChem are intended solely for informational purposes. The products available for purchase on BenchChem are specifically designed for in-vitro studies, which are conducted outside of living organisms. In-vitro studies, derived from the Latin term "in glass," involve experiments performed in controlled laboratory settings using cells or tissues. It is important to note that these products are not categorized as medicines or drugs, and they have not received approval from the FDA for the prevention, treatment, or cure of any medical condition, ailment, or disease. We must emphasize that any form of bodily introduction of these products into humans or animals is strictly prohibited by law. It is essential to adhere to these guidelines to ensure compliance with legal and ethical standards in research and experimentation.