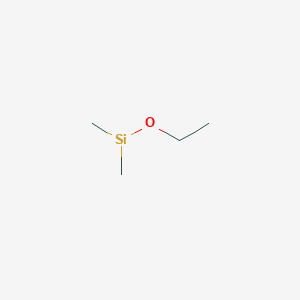
Dimethylethoxysilane
Overview
Description
Dimethylethoxysilane (DMES), with the molecular formula C₄H₁₂OSi and a molecular weight of 104.22 g/mol, is a monofunctional organosilane characterized by one ethoxy (-OCH₂CH₃) and two methyl (-CH₃) groups attached to a central silicon atom. This structure confers unique reactivity and stability properties. DMES is a low-viscosity liquid with a boiling point of 54°C, making it highly volatile and suitable for vapor-phase applications. A key industrial application of DMES is the waterproofing of silica surfaces, notably utilized by NASA to re-waterproof space shuttle tiles (HRSI) due to its ability to react with silica (SiO₂) to form stable Si-O-Si bonds while releasing ethanol as a benign byproduct . Its hydrolytic stability and controlled reactivity also make it valuable in catalytic hydrosilylation reactions, surface functionalization, and the synthesis of silicon-based nanomaterials .
Preparation Methods
Traditional Direct Alcoholysis Method
Reaction Mechanism and Process Overview
The conventional approach involves reacting dimethyldichlorosilane (DMCS) with ethanol under heated conditions. The reaction proceeds via nucleophilic substitution, where ethoxy groups replace chlorine atoms:
3)2\text{SiCl}2 + 2\text{CH}3\text{CH}2\text{OH} \rightarrow (\text{CH}3)2\text{Si(OCH}2\text{CH}3)2 + 2\text{HCl}
This exothermic process typically operates at elevated temperatures (60–80°C) to accelerate ethanol reactivity . However, HCl accumulation promotes secondary hydrolysis reactions, generating siloxane by-products such as hexamethyldisiloxane .
Challenges and By-product Formation
The absence of HCl scavengers in traditional methods leads to:
-
Siloxane formation : Hydrolysis of unreacted DMCS or product via HCl-catalyzed condensation:
-
Reduced yields : Uncontrolled HCl levels drive equilibrium toward reactant recovery, limiting yields to 65–70% .
-
Corrosive conditions : High HCl concentration necessitates expensive corrosion-resistant equipment.
Urea-Assisted Synthesis Method
Reaction Protocol and Optimization
The urea-mediated method (CN102718792A) enhances yield and purity through three stages: reaction , neutralization , and rectification .
Reaction Stage
-
Reagents : DMCS, urea, and ethanol.
-
Conditions : Sub-ambient pressure (-200 to 0 mmHg), temperature <60°C .
-
Mechanism : Urea absorbs HCl, shifting equilibrium toward product formation:
This prevents HCl-induced side reactions and stabilizes the reaction medium.
Neutralization and Rectification
-
Neutralization : Excess neutralizing agent (e.g., NaOH) ensures system neutrality, minimizing residual HCl .
-
Rectification : Batch distillation isolates this compound (b.p. 114°C) from ethanol (78.4°C) and high-boiling residues .
Yield and Scalability Data
Parameter | Embodiment 1 | Embodiment 2 | Embodiment 3 |
---|---|---|---|
DMCS (kg) | 500 | 500 | 500 |
Urea (kg) | 500 | 500 | 700 |
Ethanol (kg) | 315 | 315 | 500 |
Yield (%) | 82.2 | 83.2 | 83.4 |
Increasing urea content improves HCl absorption, boosting yield marginally (83.2% to 83.4%) .
Comparative Analysis of Preparation Methods
Performance Metrics
Parameter | Traditional Method | Urea-Assisted Method |
---|---|---|
Reaction Temperature | 60–80°C | <60°C |
HCl Management | None | Urea absorption |
Yield (%) | 65–70 | 82–83 |
By-products | Siloxanes | <5% impurities |
Energy Consumption | High | Moderate |
Economic and Environmental Impact
-
Urea method : Reduces waste HCl by 40%, lowering neutralization costs .
-
Traditional method : Requires post-reaction HCl scrubbing, increasing operational complexity.
Precursor Synthesis: Dimethyldichlorosilane Production
Dimethyldichlorosilane (DMCS), the primary precursor, is synthesized via methyltrichlorosilane disproportionation (US4552973A) :
3\text{SiCl}3 \rightarrow (\text{CH}3)2\text{SiCl}2 + \text{SiCl}4
Key Process Features :
Industrial-Scale Considerations
Process Optimization
Chemical Reactions Analysis
Types of Reactions
Dimethylethoxysilane undergoes several types of chemical reactions, including:
Hydrolysis: Reacts with water to form dimethylsilanol and ethanol.
Reduction: The silicon-hydrogen bond in this compound acts as a mild reducing agent.
Silanization: Reacts with silica surfaces to form a strong bond, making it useful for surface modification.
Common Reagents and Conditions
Water: Used in hydrolysis reactions to produce silanol and ethanol.
Silica: Used in silanization reactions to modify surface properties.
Bases: Can catalyze the formation of various products from this compound.
Major Products Formed
Dimethylsilanol: Formed during hydrolysis reactions.
Ethanol: A common by-product in many reactions involving this compound.
Scientific Research Applications
Surface Modification
Applications:
- DMES is extensively used for the modification of surfaces, particularly in creating hydrophobic coatings on glass and silicon substrates. This property is crucial for applications in biosensors and optoelectronic devices.
Case Study:
A comparative study highlighted that DMES can be utilized in both solution-phase and vapor-phase deposition methods to create uniform silane layers on hydroxyl-bearing surfaces like silicon dioxide. The resulting films exhibited high hydrolytic stability, making them ideal for sensor applications .
Surface Type | Deposition Method | Hydrophilicity | Stability |
---|---|---|---|
Silicon Dioxide | Solution Phase | Low | High |
Glass | Vapor Phase | Moderate | Moderate |
Nanotechnology
Applications:
- In nanotechnology, DMES is used as a precursor in synthesizing silica nanoparticles. These nanoparticles find applications in drug delivery systems and as catalysts.
Case Study:
Research demonstrated that DMES can be employed as a macroinitiator for the polymerization of methyl methacrylate, leading to the formation of polymer-coated quantum dots. These structures are beneficial for selective ion recognition in aqueous solutions .
Waterproofing Agents
Applications:
- DMES serves as an effective waterproofing agent for various substrates, including thermal tiles used in aerospace applications.
Case Study:
NASA utilized DMES in developing waterproof coatings for space shuttle thermal tiles. The compound's ability to form high-boiling polymeric by-products enhances the durability and effectiveness of these coatings under extreme conditions .
Environmental Applications
Applications:
- The compound is also explored for its potential use in environmental remediation processes, particularly in treating contaminated water sources.
Case Study:
Inhalation exposure studies conducted on Fischer 344 rats indicated that DMES vapor can be monitored for safety during industrial applications, ensuring that environmental exposure levels remain within safe limits .
Industrial Coatings
Applications:
- DMES is employed in formulating industrial coatings that require enhanced durability and chemical resistance.
Case Study:
A study on multifunctional smart coatings highlighted the use of DMES to accelerate reactions in single-component systems, thereby improving the performance of protective coatings against environmental factors .
Mechanism of Action
The mechanism of action of dimethylethoxysilane involves the formation of strong bonds with silica surfaces through the silanization reaction. The silicon-hydrogen bond in the compound is polarized, making the hydrogen slightly hydridic and allowing it to act as a mild reducing agent . This property is exploited in various chemical reactions and surface modification processes.
Comparison with Similar Compounds
To contextualize DMES’s properties and applications, a comparative analysis with structurally related organosilanes is provided below.
Functional Group Reactivity and Hydrolytic Stability
DMES’s monofunctional ethoxy group contrasts with trialkoxysilanes like (3-aminopropyl)triethoxysilane (APTES) (three ethoxy groups) and bifunctional derivatives like (3-aminopropyl)dimethylethoxysilane (APDMES) (one ethoxy, two methyl groups). Key differences include:
- APTES: With three ethoxy groups, APTES exhibits higher cross-linking capacity on silica surfaces, making it a staple in biosensor fabrication and nanoparticle functionalization. However, its rapid hydrolysis can lead to uncontrolled polymerization in humid environments .
- APDMES: The substitution of two ethoxy groups with methyl groups in APDMES reduces hydrolysis rates while maintaining sufficient reactivity for surface anchoring. Studies demonstrate that APDMES-modified silicon nanowires (SiNWs@Al₂O₃) exhibit enhanced chemical stability under physiological conditions, critical for long-term implantable biosensors .
- DMES : The single ethoxy group limits cross-linking but improves hydrolytic control. DMES-modified surfaces show intermediate stability, balancing reactivity and durability .
Table 1: Functional Group and Stability Comparison
Catalytic Performance in Hydrosilylation
DMES is widely employed in palladium-catalyzed hydrosilylation reactions. In regioselective allene hydroarylation , DMES achieved 76–99% yields with 98:2 regioselectivity under optimized Pd/ligand systems, outperforming dimethylphenylsilane (83% yield) in analogous reactions . The ethoxy group’s electron-withdrawing effect enhances silicon’s electrophilicity, facilitating transmetalation steps in catalytic cycles .
Table 2: Reaction Yields and Selectivity
Silane Reagent | Catalyst System | Yield (%) | Regioselectivity (Ratio) |
---|---|---|---|
DMES | Pd₂dba₃/IPr*OMe·HBF₄ | 76–99 | 98:2 |
Dimethylphenylsilane | Pd₂dba₃/IPr*OMe | 83 | Not reported |
Surface Modification Efficiency
DMES derivatives like APDMES enable precise control over surface ligand density. Kanan et al. demonstrated that APDMES, when adsorbed on silica powders with amine catalysts, achieves double the surface concentration compared to APTES, attributed to steric hindrance reduction from methyl groups . This property is exploited in chromatography and drug delivery systems where high ligand density is critical .
Comparison with Halogenated and Vinyl Silanes
- Chloromethyldiethoxysilane : The chlorine substituent increases electrophilicity, enabling nucleophilic substitution reactions. However, it is less stable than DMES due to hydrolytic sensitivity of the Si-Cl bond .
- Ethoxydimethylvinylsilane : The vinyl group introduces olefin reactivity, broadening applications in polymerization and adhesion promotion. Its higher boiling point (100°C vs. DMES’s 54°C) limits vapor-phase uses .
Biological Activity
Dimethylethoxysilane (DMES) is an organosilicon compound that has garnered attention for its potential biological activities, particularly in the fields of biomedicine and material science. This article explores the biological activity of DMES, including its cytotoxic effects, potential applications in drug delivery systems, and surface functionalization for biomedical devices.
This compound is characterized by the presence of ethoxy and dimethyl groups attached to a silicon atom. Its chemical formula is , and it has a molecular weight of 132.22 g/mol. The compound can be represented as follows:
Cytotoxicity Studies
Research indicates that DMES exhibits varying degrees of cytotoxicity against different cell lines. In a study evaluating its effects on HeLa cells, it was found that DMES has an IC50 value ranging from 100 to 1000 µg/mL, indicating moderate cytotoxicity compared to standard chemotherapeutics like doxorubicin, which has an IC50 of 7.72 µg/mL .
Table 1: Cytotoxicity of DMES on HeLa Cells
Toxicological Studies
Toxicological assessments have shown that DMES is mildly to moderately toxic when inhaled at concentrations above 1000 ppm, leading to symptoms such as narcosis . However, it does not exhibit mutagenic properties, making it a candidate for further exploration in therapeutic applications.
Applications in Drug Delivery and Surface Modification
This compound has been utilized in the functionalization of surfaces for biomedical applications, particularly in enhancing biocompatibility and promoting cell adhesion. For instance, studies have demonstrated that DMES can be used to modify titanium surfaces to improve osteoblast and endothelial cell proliferation .
Table 2: Effects of DMES Functionalization on Cell Proliferation
Substrate Type | Cell Type | Proliferation Rate (%) | Reference |
---|---|---|---|
Titanium | Osteoblasts | 75 | |
Titanium | Endothelial Cells | 80 |
Case Studies
- Surface Functionalization for Improved Biocompatibility
- Photodynamic Therapy Applications
Q & A
Basic Research Questions
Q. How can researchers synthesize dimethylethoxysilane and ensure its purity for experimental use?
- Methodological Answer : this compound is typically synthesized via alcoholysis of chlorosilanes or condensation reactions. To verify purity, gas chromatography-mass spectrometry (GC-MS) and nuclear magnetic resonance (NMR) spectroscopy (¹H and ²⁹Si) are essential. For example, ¹H NMR can confirm the absence of residual ethanol or byproducts by analyzing peaks at δ 1.2 ppm (CH₃ of ethoxy groups) and δ 0.1–0.3 ppm (Si–CH₃). Quantitative FTIR analysis can also monitor Si–O–C bonds (~1050 cm⁻¹) and Si–CH₃ vibrations (~1250 cm⁻¹) .
Q. What analytical techniques are recommended to study the hydrolysis kinetics of this compound under varying pH and temperature conditions?
- Methodological Answer : Hydrolysis rates can be measured using in situ FTIR or Raman spectroscopy to track Si–O–C bond cleavage. For example, notes that hydrolysis rates relative to other silanes (e.g., SIG5840.0) can be quantified via time-resolved spectral changes. Conductivity measurements or pH titration can also monitor proton release during hydrolysis. Data should be analyzed using pseudo-first-order kinetics models, with Arrhenius plots to determine activation energies under controlled humidity .
Q. How should experiments be designed to evaluate the thermal and humidity stability of this compound in material science applications?
- Methodological Answer : Accelerated aging studies in environmental chambers can simulate high humidity (e.g., 85% RH at 85°C) and thermal stress (e.g., 100–150°C). Stability is assessed via gravimetric analysis (mass loss) and spectroscopic monitoring of degradation byproducts. For instance, Johnson and Ford (1990) used TGA-DSC to correlate silane decomposition temperatures with humidity exposure . Control experiments should include inert atmospheres (N₂) to isolate moisture-driven degradation .
Advanced Research Questions
Q. How can researchers resolve contradictions in reported stability data for this compound under high-humidity conditions?
- Methodological Answer : Contradictions often arise from variations in experimental design (e.g., substrate porosity, silane deposition methods). A systematic approach involves:
- Replicating conditions : Use standardized substrates (e.g., silica nanoparticles) and controlled humidity chambers.
- Cross-validation : Compare FTIR, XPS, and contact angle measurements to assess surface hydration.
- Meta-analysis : Review historical data (e.g., Johnson and Ford, 1990 vs. Dow Corning, 1976) to identify methodological discrepancies, such as differences in curing times or catalyst use .
Q. What advanced methodologies enhance surface modification efficacy using this compound for functionalized materials?
- Methodological Answer : To optimize surface coverage, describes a method to double the surface concentration of (3-aminopropyl)this compound on silica via solvent-controlled adsorption (e.g., using anhydrous toluene). Techniques include:
- Langmuir-Blodgett deposition for monolayer control.
- Ellipsometry or QCM-D to measure adsorption kinetics.
- X-ray photoelectron spectroscopy (XPS) to confirm covalent bonding (Si–O–Si) and amine group availability .
Q. How should researchers address conflicting data on the reactivity of this compound with polar vs. non-polar substrates?
- Methodological Answer : Contradictions may stem from substrate pretreatment or solvent polarity. A robust protocol includes:
- Surface activation : Plasma treatment for polar substrates (e.g., glass) vs. silanization in non-polar solvents (e.g., hexane) for hydrophobic polymers.
- Competitive adsorption studies : Use labeled silanes (e.g., fluorescent tags) to quantify binding efficiency.
- Statistical analysis : Apply ANOVA to evaluate significance across substrate types, referencing raw data tables (e.g., hydrolysis rates from ) .
Q. Data Presentation and Reproducibility
Q. What best practices ensure data integrity when reporting this compound reactivity in peer-reviewed studies?
- Methodological Answer :
- Raw data archiving : Store spectra, chromatograms, and kinetic curves in supplementary materials (per ).
- Uncertainty quantification : Report standard deviations for triplicate measurements and instrument error margins (e.g., ±0.1°C for DSC).
- Reproducibility checks : Provide step-by-step protocols for silane deposition and curing, as seen in ’s surface modification study .
Q. How should researchers structure a chemical manuscript to highlight novel findings on this compound applications?
- Introduction : Contextualize silane coupling agents within material science (cite ).
- Methods : Detail synthesis, characterization (e.g., "NMR spectra acquired at 400 MHz in CDCl₃"), and statistical models.
- Results : Use tables to compare hydrolysis rates (e.g., SIG5836.0 vs. SIG5840.0 from ) and figures for surface morphology (SEM/AFM).
- Discussion : Address limitations (e.g., humidity sensitivity) and propose mechanisms for observed reactivity .
Properties
InChI |
InChI=1S/C4H11OSi/c1-4-5-6(2)3/h4H2,1-3H3 | |
---|---|---|
Source | PubChem | |
URL | https://pubchem.ncbi.nlm.nih.gov | |
Description | Data deposited in or computed by PubChem | |
InChI Key |
DRUOQOFQRYFQGB-UHFFFAOYSA-N | |
Source | PubChem | |
URL | https://pubchem.ncbi.nlm.nih.gov | |
Description | Data deposited in or computed by PubChem | |
Canonical SMILES |
CCO[Si](C)C | |
Source | PubChem | |
URL | https://pubchem.ncbi.nlm.nih.gov | |
Description | Data deposited in or computed by PubChem | |
Molecular Formula |
C4H11OSi | |
Source | PubChem | |
URL | https://pubchem.ncbi.nlm.nih.gov | |
Description | Data deposited in or computed by PubChem | |
DSSTOX Substance ID |
DTXSID0051734 | |
Record name | Ethoxydimethylsilane | |
Source | EPA DSSTox | |
URL | https://comptox.epa.gov/dashboard/DTXSID0051734 | |
Description | DSSTox provides a high quality public chemistry resource for supporting improved predictive toxicology. | |
Molecular Weight |
103.21 g/mol | |
Source | PubChem | |
URL | https://pubchem.ncbi.nlm.nih.gov | |
Description | Data deposited in or computed by PubChem | |
CAS No. |
14857-34-2 | |
Record name | Silane, ethoxydimethyl- | |
Source | ChemIDplus | |
URL | https://pubchem.ncbi.nlm.nih.gov/substance/?source=chemidplus&sourceid=0014857342 | |
Description | ChemIDplus is a free, web search system that provides access to the structure and nomenclature authority files used for the identification of chemical substances cited in National Library of Medicine (NLM) databases, including the TOXNET system. | |
Record name | Silane, ethoxydimethyl- | |
Source | EPA Chemicals under the TSCA | |
URL | https://www.epa.gov/chemicals-under-tsca | |
Description | EPA Chemicals under the Toxic Substances Control Act (TSCA) collection contains information on chemicals and their regulations under TSCA, including non-confidential content from the TSCA Chemical Substance Inventory and Chemical Data Reporting. | |
Record name | Ethoxydimethylsilane | |
Source | EPA DSSTox | |
URL | https://comptox.epa.gov/dashboard/DTXSID0051734 | |
Description | DSSTox provides a high quality public chemistry resource for supporting improved predictive toxicology. | |
Record name | Ethoxydimethylsilane | |
Source | European Chemicals Agency (ECHA) | |
URL | https://echa.europa.eu/substance-information/-/substanceinfo/100.035.368 | |
Description | The European Chemicals Agency (ECHA) is an agency of the European Union which is the driving force among regulatory authorities in implementing the EU's groundbreaking chemicals legislation for the benefit of human health and the environment as well as for innovation and competitiveness. | |
Explanation | Use of the information, documents and data from the ECHA website is subject to the terms and conditions of this Legal Notice, and subject to other binding limitations provided for under applicable law, the information, documents and data made available on the ECHA website may be reproduced, distributed and/or used, totally or in part, for non-commercial purposes provided that ECHA is acknowledged as the source: "Source: European Chemicals Agency, http://echa.europa.eu/". Such acknowledgement must be included in each copy of the material. ECHA permits and encourages organisations and individuals to create links to the ECHA website under the following cumulative conditions: Links can only be made to webpages that provide a link to the Legal Notice page. | |
Record name | Ethoxydimethylsilane | |
Source | FDA Global Substance Registration System (GSRS) | |
URL | https://gsrs.ncats.nih.gov/ginas/app/beta/substances/P2H8FET3FK | |
Description | The FDA Global Substance Registration System (GSRS) enables the efficient and accurate exchange of information on what substances are in regulated products. Instead of relying on names, which vary across regulatory domains, countries, and regions, the GSRS knowledge base makes it possible for substances to be defined by standardized, scientific descriptions. | |
Explanation | Unless otherwise noted, the contents of the FDA website (www.fda.gov), both text and graphics, are not copyrighted. They are in the public domain and may be republished, reprinted and otherwise used freely by anyone without the need to obtain permission from FDA. Credit to the U.S. Food and Drug Administration as the source is appreciated but not required. | |
Retrosynthesis Analysis
AI-Powered Synthesis Planning: Our tool employs the Template_relevance Pistachio, Template_relevance Bkms_metabolic, Template_relevance Pistachio_ringbreaker, Template_relevance Reaxys, Template_relevance Reaxys_biocatalysis model, leveraging a vast database of chemical reactions to predict feasible synthetic routes.
One-Step Synthesis Focus: Specifically designed for one-step synthesis, it provides concise and direct routes for your target compounds, streamlining the synthesis process.
Accurate Predictions: Utilizing the extensive PISTACHIO, BKMS_METABOLIC, PISTACHIO_RINGBREAKER, REAXYS, REAXYS_BIOCATALYSIS database, our tool offers high-accuracy predictions, reflecting the latest in chemical research and data.
Strategy Settings
Precursor scoring | Relevance Heuristic |
---|---|
Min. plausibility | 0.01 |
Model | Template_relevance |
Template Set | Pistachio/Bkms_metabolic/Pistachio_ringbreaker/Reaxys/Reaxys_biocatalysis |
Top-N result to add to graph | 6 |
Feasible Synthetic Routes
Disclaimer and Information on In-Vitro Research Products
Please be aware that all articles and product information presented on BenchChem are intended solely for informational purposes. The products available for purchase on BenchChem are specifically designed for in-vitro studies, which are conducted outside of living organisms. In-vitro studies, derived from the Latin term "in glass," involve experiments performed in controlled laboratory settings using cells or tissues. It is important to note that these products are not categorized as medicines or drugs, and they have not received approval from the FDA for the prevention, treatment, or cure of any medical condition, ailment, or disease. We must emphasize that any form of bodily introduction of these products into humans or animals is strictly prohibited by law. It is essential to adhere to these guidelines to ensure compliance with legal and ethical standards in research and experimentation.