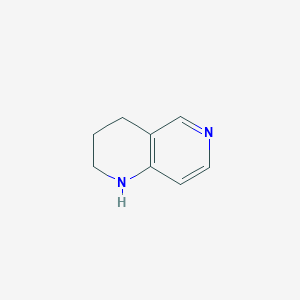
1,2,3,4-Tetrahydro-1,6-naphthyridine
Overview
Description
Synthesis Analysis
The synthesis of 1,2,3,4-tetrahydro-1,6-naphthyridine involves multiple steps, starting from simpler precursors such as 2-methylpyrazine. A notable method includes a six-step reaction process encompassing condensation, elimination, addition, and protection/removal of amino groups, yielding a total yield of 47.3% (Teng Da-wei, 2010). Another approach utilizes cobalt-catalyzed [2 + 2 + 2] cyclizations under microwave-promotion to efficiently synthesize 5,6,7,8-tetrahydro-1,6-naphthyridines, showcasing the adaptability in synthesizing these compounds (Ya Zhou, John A Porco, John K Snyder, 2007).
Molecular Structure Analysis
The molecular structure of 1,2,3,4-tetrahydro-1,6-naphthyridine and its derivatives has been confirmed through various analytical techniques, including 1H-NMR, 13C-NMR, elemental analysis, and mass spectrometry. These techniques ensure the accurate determination of the compound's structure and the success of the synthetic methods employed.
Chemical Reactions and Properties
1,2,3,4-Tetrahydro-1,6-naphthyridine undergoes various chemical reactions, including condensation with amines, cycloaddition reactions, and heteroannulation processes. For instance, a novel four-component strategy led to the efficient synthesis of unprecedented dipyrazolo-fused 2,6-naphthyridines, demonstrating the compound's versatility in forming tetra-heterocyclic scaffolds (Wei-Qiang Fan et al., 2013).
Physical Properties Analysis
The physical properties of 1,2,3,4-tetrahydro-1,6-naphthyridine derivatives vary widely depending on the substituents and the synthetic route used. These properties include solubility in various solvents, melting points, and crystalline forms, which are crucial for their application in different fields of research and industry.
Chemical Properties Analysis
The chemical properties of 1,2,3,4-tetrahydro-1,6-naphthyridine are influenced by its naphthyridine core and the tetrahydro moiety. This structure imparts reactivity towards nucleophiles, electrophiles, and the ability to participate in cycloaddition reactions. The compound's versatility is further demonstrated by its reactivity in tandem synthesis approaches, enabling the regioselective synthesis of highly functionalized derivatives (A. Verma et al., 2013).
Scientific Research Applications
Synthesis Methods :
- Teng Da-wei (2010) discussed a six-step synthesis method for 1,2,3,4-tetrahydro-2,6-naphthyridine, starting from 2-methylpyrazine (Teng, 2010).
- Armarego (1967) explored the reduction of naphthyridines with sodium and ethanol, including the synthesis of 1,2,3,4-tetrahydro-derivatives (Armarego, 1967).
- Other studies, such as by Zhou, Porco, and Snyder (2007), and Puchakayala Bharath Kumar Reddy et al. (2018), have also reported on the synthesis of various tetrahydro-1,6-naphthyridines (Zhou, Porco, & Snyder, 2007); (Reddy, Ravi, Mahesh, & Leelavathi, 2018).
Biomedical Applications :
- M. Lavanya et al. (2021) discussed the pharmacological activities of 1,6-naphthyridines, highlighting their potential in anticancer, anti-HIV, antimicrobial, analgesic, anti-inflammatory, and antioxidant applications (Lavanya et al., 2021).
- A study by Almansour et al. (2015) indicated that hexahydro-1,6-naphthyridines could inhibit acetylcholinesterase, a target for Alzheimer’s disease treatment (Almansour et al., 2015).
Chemical Properties and Reactions :
- Jianwei Zhang et al. (2015) developed an asymmetric hydrogenation of 1,5-naphthyridines to produce 1,2,3,4-tetrahydro-1,5-naphthyridines, useful in asymmetric synthesis (Zhang, Chen, He, & Fan, 2015).
- A. Shiozawa et al. (1984) synthesized 5,6,7,8-tetrahydro-1,6-naphthyridine methyl homologs, exploring their potential as antivertigo agents (Shiozawa, Ichikawa, Komuro, Kurashige, Miyazaki, Yamanaka, & Sakamoto, 1984).
Environmental Applications :
- A. A. Abdel Hameed (2015) reported a solvent-free and catalyst-free synthesis of 1,6-naphthyridine derivatives, highlighting an environmentally friendly approach (Hameed, 2015).
Safety And Hazards
Future Directions
properties
IUPAC Name |
1,2,3,4-tetrahydro-1,6-naphthyridine | |
---|---|---|
Source | PubChem | |
URL | https://pubchem.ncbi.nlm.nih.gov | |
Description | Data deposited in or computed by PubChem | |
InChI |
InChI=1S/C8H10N2/c1-2-7-6-9-5-3-8(7)10-4-1/h3,5-6,10H,1-2,4H2 | |
Source | PubChem | |
URL | https://pubchem.ncbi.nlm.nih.gov | |
Description | Data deposited in or computed by PubChem | |
InChI Key |
ARCSZHIPWBDSGJ-UHFFFAOYSA-N | |
Source | PubChem | |
URL | https://pubchem.ncbi.nlm.nih.gov | |
Description | Data deposited in or computed by PubChem | |
Canonical SMILES |
C1CC2=C(C=CN=C2)NC1 | |
Source | PubChem | |
URL | https://pubchem.ncbi.nlm.nih.gov | |
Description | Data deposited in or computed by PubChem | |
Molecular Formula |
C8H10N2 | |
Source | PubChem | |
URL | https://pubchem.ncbi.nlm.nih.gov | |
Description | Data deposited in or computed by PubChem | |
DSSTOX Substance ID |
DTXSID20475913 | |
Record name | 1,2,3,4-TETRAHYDRO-1,6-NAPHTHYRIDINE | |
Source | EPA DSSTox | |
URL | https://comptox.epa.gov/dashboard/DTXSID20475913 | |
Description | DSSTox provides a high quality public chemistry resource for supporting improved predictive toxicology. | |
Molecular Weight |
134.18 g/mol | |
Source | PubChem | |
URL | https://pubchem.ncbi.nlm.nih.gov | |
Description | Data deposited in or computed by PubChem | |
Product Name |
1,2,3,4-Tetrahydro-1,6-naphthyridine | |
CAS RN |
13623-84-2 | |
Record name | 1,2,3,4-Tetrahydro-1,6-naphthyridine | |
Source | CAS Common Chemistry | |
URL | https://commonchemistry.cas.org/detail?cas_rn=13623-84-2 | |
Description | CAS Common Chemistry is an open community resource for accessing chemical information. Nearly 500,000 chemical substances from CAS REGISTRY cover areas of community interest, including common and frequently regulated chemicals, and those relevant to high school and undergraduate chemistry classes. This chemical information, curated by our expert scientists, is provided in alignment with our mission as a division of the American Chemical Society. | |
Explanation | The data from CAS Common Chemistry is provided under a CC-BY-NC 4.0 license, unless otherwise stated. | |
Record name | 1,2,3,4-TETRAHYDRO-1,6-NAPHTHYRIDINE | |
Source | EPA DSSTox | |
URL | https://comptox.epa.gov/dashboard/DTXSID20475913 | |
Description | DSSTox provides a high quality public chemistry resource for supporting improved predictive toxicology. | |
Retrosynthesis Analysis
AI-Powered Synthesis Planning: Our tool employs the Template_relevance Pistachio, Template_relevance Bkms_metabolic, Template_relevance Pistachio_ringbreaker, Template_relevance Reaxys, Template_relevance Reaxys_biocatalysis model, leveraging a vast database of chemical reactions to predict feasible synthetic routes.
One-Step Synthesis Focus: Specifically designed for one-step synthesis, it provides concise and direct routes for your target compounds, streamlining the synthesis process.
Accurate Predictions: Utilizing the extensive PISTACHIO, BKMS_METABOLIC, PISTACHIO_RINGBREAKER, REAXYS, REAXYS_BIOCATALYSIS database, our tool offers high-accuracy predictions, reflecting the latest in chemical research and data.
Strategy Settings
Precursor scoring | Relevance Heuristic |
---|---|
Min. plausibility | 0.01 |
Model | Template_relevance |
Template Set | Pistachio/Bkms_metabolic/Pistachio_ringbreaker/Reaxys/Reaxys_biocatalysis |
Top-N result to add to graph | 6 |
Feasible Synthetic Routes
Citations
Disclaimer and Information on In-Vitro Research Products
Please be aware that all articles and product information presented on BenchChem are intended solely for informational purposes. The products available for purchase on BenchChem are specifically designed for in-vitro studies, which are conducted outside of living organisms. In-vitro studies, derived from the Latin term "in glass," involve experiments performed in controlled laboratory settings using cells or tissues. It is important to note that these products are not categorized as medicines or drugs, and they have not received approval from the FDA for the prevention, treatment, or cure of any medical condition, ailment, or disease. We must emphasize that any form of bodily introduction of these products into humans or animals is strictly prohibited by law. It is essential to adhere to these guidelines to ensure compliance with legal and ethical standards in research and experimentation.