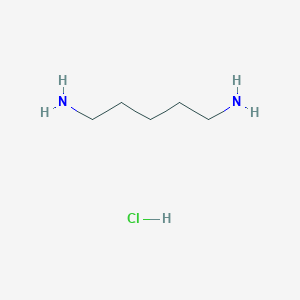
Cadaverine dihydrochloride
Overview
Description
Cadaverine dihydrochloride (C₅H₁₄N₂·2HCl) is the dihydrochloride salt of cadaverine (1,5-pentanediamine), a biogenic amine formed during the decarboxylation of lysine by bacterial action. It is a white crystalline solid with a molecular weight of 175.1 g/mol . The compound is highly water-soluble and stable in acidic conditions, making it suitable for laboratory and industrial applications such as microbial growth studies, enzymatic assays, and analytical standards .
Preparation Methods
Chemical Synthesis of Cadaverine Dihydrochloride
Decarboxylation of Lysine Hydrochloride
The most direct route to cadaverine involves the thermal decarboxylation of lysine hydrochloride. This reaction eliminates the carboxyl group from lysine, producing cadaverine and carbon dioxide. A proof-of-concept demonstration by a synthetic chemistry enthusiast showcased two variants of this method: basic heating and destructive distillation1.
In the basic heating approach, lysine hydrochloride is mixed with sodium bicarbonate to neutralize liberated hydrochloric acid during decomposition. The mixture is heated in an open system, but excessive temperatures often lead to partial combustion, reducing yields to below 20%1. The destructive distillation method, conducted under a nitrogen atmosphere, prevents oxidation and allows higher temperatures (up to 179°C) without combustion. This closed-system approach achieved a 40% yield, though the putrid odor of cadaverine necessitated immediate trapping in aqueous solution1.
The reaction mechanism involves the removal of the α-carboxyl group from lysine via a six-membered transition state, releasing CO₂ and forming the primary amine cadaverine1. Key parameters influencing yield include:
-
Base stoichiometry : Excess sodium bicarbonate (molar ratio 1:2 lysine:NaHCO₃) improves HCl neutralization1.
-
Atmosphere : Inert gases (e.g., nitrogen) suppress side reactions1.
Table 1: Comparison of Heating Methods for Lysine Decarboxylation
Parameter | Basic Heating | Destructive Distillation |
---|---|---|
Temperature Range | 120–150°C | 150–180°C |
Yield | <20% | ~40% |
Byproducts | Combustion residues | Minimal impurities |
Scalability | Low | Moderate |
Destructive Distillation Setup and Optimization
The destructive distillation apparatus described in experimental demonstrations consists of a nitrogen-flushed reaction tube connected to a water-cooled condenser1. Lysine hydrochloride and sodium bicarbonate are heated in a sealed environment, allowing vaporized cadaverine to condense into a water trap. This method minimizes product loss due to its closed-loop design, though post-reaction extraction remains challenging due to the compound’s high solubility in polar solvents1 .
Critical considerations for this method include:
-
Nitrogen flow rate : 0.5–1 L/min to maintain an oxygen-free environment1.
-
Trapping solvent : Water is preferred over ethanol due to flammability risks1.
-
Scaling limitations : Batch sizes exceeding 10 g risk incomplete distillation due to uneven heating1.
Biological Production via Metabolic Engineering
Engineering Escherichia coli for Cadaverine Biosynthesis
Recent advances in metabolic engineering have enabled the bio-based production of cadaverine using recombinant E. coli. By overexpressing lysine decarboxylase (CadA) and optimizing lysine biosynthesis, researchers achieved a fed-batch yield of 9.61 g/L cadaverine . Key genetic modifications include:
-
Deletion of degradation pathways : Knocking out genes responsible for cadaverine catabolism (e.g., ygiG) .
-
Promoter engineering : Replacing native promoters of dapA (dihydrodipicolinate synthase) with the strong trc promoter to enhance lysine flux .
-
Overexpression of CadA : Plasmid-based expression of cadA under the tac promoter .
Table 2: Performance of Engineered E. coli Strains
Strain | CadA Expression | Lysine Yield (g/L) | Cadaverine Yield (g/L) |
---|---|---|---|
Wild-type | Native | 1.2 | 0.08 |
XQ56 (p15CadA) | High | 4.8 | 9.61 |
Fermentation and Downstream Processing
Fed-batch fermentation using glucose mineral salts medium achieved a productivity of 0.32 g/L/h . Post-fermentation, cells are lysed, and cadaverine is recovered via centrifugation and filtration. The crude extract is then acidified with hydrochloric acid to precipitate this compound, which is purified through recrystallization .
Purification and Isolation Techniques
Solvent-Based Purification
A Chinese patent (CN105612257A) describes a high-boiling-point solvent method for purifying cadaverine . Impure cadaverine is dissolved in a solvent like ethylene glycol (bp 197°C) and subjected to vacuum distillation. This approach removes low-boiling impurities (e.g., ammonia) while retaining cadaverine in the solvent phase. Subsequent evaporation under reduced pressure yields >95% pure this compound .
Table 3: Solvent Performance in Cadaverine Purification
Solvent | Boiling Point (°C) | Purity (%) | Recovery (%) |
---|---|---|---|
Ethylene glycol | 197 | 95.2 | 89 |
Glycerol | 290 | 93.8 | 85 |
Distillation and Evaporation
Multi-stage distillation is critical for isolating this compound from aqueous solutions. The patent recommends fractional distillation at 80–90°C under 10–15 mmHg, followed by evaporation at 60°C to concentrate the product . This method reduces residual lysine and sodium chloride to <0.5% .
Comparative Analysis of Preparation Methods
Efficiency and Scalability
-
Chemical synthesis : Suitable for small-scale laboratory production but limited by low yields and hazardous byproducts1 .
-
Biological production : Scalable for industrial applications with higher yields, though dependent on costly fermentation infrastructure .
-
Purification : Solvent-based methods offer high purity but require energy-intensive distillation .
Chemical Reactions Analysis
Types of Reactions: Cadaverine dihydrochloride undergoes various chemical reactions, including:
Oxidation: Cadaverine can be oxidized to produce corresponding aldehydes and acids.
Reduction: It can be reduced to form primary amines.
Substitution: Cadaverine can participate in substitution reactions, where its amino groups are replaced by other functional groups.
Common Reagents and Conditions:
Oxidation: Common oxidizing agents include potassium permanganate and hydrogen peroxide.
Reduction: Reducing agents such as lithium aluminum hydride are used.
Substitution: Reagents like alkyl halides and acyl chlorides are commonly used.
Major Products:
Oxidation: Produces aldehydes and acids.
Reduction: Forms primary amines.
Substitution: Results in various substituted amines.
Scientific Research Applications
Cadaverine dihydrochloride has a wide range of applications in scientific research:
Chemistry: Used as a monomer for the development of high-performance polyamides, polyurethanes, and polyureas.
Biology: Participates in plant physiological processes such as cell division and growth, promoting the development of pistils and stamens, and regulating senescence.
Medicine: Used to treat arrhythmia, relieve hypoglycemia, and effectively cure dysentery.
Industry: Applied in the production of renewable polymers, such as nylon 5X materials, which have excellent mechanical strength, air permeability, moisture absorption, and flame retardancy
Mechanism of Action
Cadaverine dihydrochloride exerts its effects through various molecular targets and pathways. In zebrafish, the trace amine-associated receptor 13c (TAAR13c) has been identified as a high-affinity receptor for cadaverine . In humans, molecular modeling and docking experiments have shown that cadaverine fits into the binding pocket of the human TAAR6 and TAAR8 .
Comparison with Similar Compounds
Key Properties :
- Purity : ≥98% (HPLC-grade) .
- Nitrogen Assimilation : Acts as a sole nitrogen source for certain fungi and yeast, unlike nitrate or nitrite salts .
- Toxicity : At 200 mM (35 g/L), it reduces microbial growth rates by ~20% .
Cadaverine dihydrochloride belongs to the biogenic amine hydrochloride family, which includes putrescine dihydrochloride, spermidine trihydrochloride, and histamine dihydrochloride. Below is a comparative analysis based on structural, functional, and applicative properties.
Structural and Physical Properties
Notes:
- Cadaverine and putrescine are structurally similar (pentanediamine vs. butanediamine) but differ in chain length, affecting their biological roles .
- Histamine dihydrochloride has a higher nitrogen content due to its imidazole ring, enhancing its reactivity in allergic responses .
Functional Differences
Microbial Assimilation
- This compound : Assimilated as a nitrogen source by fungi (e.g., Candida spp.) but inhibits growth at >50 mM .
- Putrescine dihydrochloride : Similarly assimilated but with fewer inhibitory effects at equivalent concentrations .
- Ammonium chloride : A preferred nitrogen source for rapid microbial growth but lacks the signaling roles of polyamines .
Enzymatic Substrate Specificity
- This compound : Used as a substrate for diamine oxidase (DAO) assays at 97.8 mM .
- Histamine dihydrochloride : Substrate for histamine decarboxylase, critical in immune responses .
Analytical Considerations
- Standard Preparation :
- This compound requires 171.4 mg to prepare 1000 mg/L stock solutions, whereas putrescine dihydrochloride requires 182.7 mg due to differences in molecular weight .
- Miscalculations in molarity can occur if the dihydrochloride form is mistaken for the free base (e.g., this compound vs. cadaverine: 175.1 g/mol vs. 102.18 g/mol) .
Critical Notes
- Isomeric Differences : Cadaverine and saprine are structural isomers but differ in solubility and toxicity profiles .
- Analytical Precision : Studies using biogenic amine hydrochlorides must account for salt forms to avoid concentration errors, as highlighted in corrigenda .
- Toxicity Thresholds : While this compound is mildly inhibitory, histamine dihydrochloride exhibits stronger physiological effects due to receptor interactions .
Q & A
Basic Questions
Q. How can researchers verify the purity of cadaverine dihydrochloride for experimental use?
Methodology:
- Quantitative Nuclear Magnetic Resonance (qNMR): A validated method for purity determination involves using ethyl paraben as an internal standard. Optimal NMR parameters include a relaxation delay of 30 seconds and 16 scans to ensure accuracy. Purity values >98% are typically achieved .
- Certificates of Analysis (COA): Cross-check batch-specific COA documents for HPLC/UV-Vis data, ensuring consistency with published protocols (e.g., purity >99.50% in GC35848 batch standards) .
Q. What are the recommended storage conditions to maintain this compound stability?
Methodology:
- Store at –20°C in airtight, light-protected containers to prevent hydrolysis. Avoid exposure to strong acids/alkalis or oxidizing agents, which may degrade the compound .
- For long-term stability (>6 months), lyophilized forms in ethanol:water (2:98) solutions are preferable .
Advanced Research Questions
Q. How can conflicting data on this compound’s role in bacterial efflux pump inhibition be resolved?
Methodology:
- Comparative Assay Design: Use standardized efflux pump inhibitors (e.g., PAβN dihydrochloride ) as positive controls. Monitor cadaverine’s interaction with Gram-negative bacterial membranes via fluorescence-based transport assays.
- Data Reconciliation: Analyze batch-specific activity (e.g., isotopic labeling with [1,5-14C] this compound ) to isolate experimental variability from intrinsic biological effects.
Q. What experimental strategies are optimal for studying this compound’s interaction with plant polyamine pathways?
Methodology:
- Metabolic Profiling: Combine HPLC with dansyl chloride derivatization to quantify polyamines (e.g., putrescine, spermidine) in plant tissues. This compound competes with endogenous polyamines for biosynthesis enzymes .
- Gene Expression Analysis: Use RT-qPCR to monitor BrACO2 expression, a key enzyme in ethylene synthesis influenced by polyamine levels .
Q. How can researchers address the lack of toxicological data for this compound in mammalian cell models?
Methodology:
- Standardized Tox Screens: Follow MIACARM guidelines for cellular assays, including dose-response curves (0.1–100 µM) and endpoints like apoptosis (caspase-3 activation) and oxidative stress (H3K4/H3K9 methylation ).
- Cross-Validation: Compare results with structurally similar compounds (e.g., Radafaxine hydrochloride ) to infer potential toxicity mechanisms.
Methodological Contradictions and Solutions
- Stability vs. Reactivity: While claims stability under recommended conditions, notes decomposition in acidic environments. Resolution: Pre-test this compound in experimental buffers using LC-MS to detect degradation products .
- Purity Discrepancies: Commercial batches (e.g., GC35848 vs. GC68795) vary in purity thresholds. Resolution: Normalize concentrations via qNMR before comparative studies .
Properties
CAS No. |
1476-39-7 |
---|---|
Molecular Formula |
C5H15ClN2 |
Molecular Weight |
138.64 g/mol |
IUPAC Name |
pentane-1,5-diamine;hydrochloride |
InChI |
InChI=1S/C5H14N2.ClH/c6-4-2-1-3-5-7;/h1-7H2;1H |
InChI Key |
RLNAIWYXIAJDTN-UHFFFAOYSA-N |
SMILES |
C(CCN)CCN.Cl.Cl |
Canonical SMILES |
C(CCN)CCN.Cl |
Key on ui other cas no. |
1476-39-7 |
Pictograms |
Irritant |
Origin of Product |
United States |
Retrosynthesis Analysis
AI-Powered Synthesis Planning: Our tool employs the Template_relevance Pistachio, Template_relevance Bkms_metabolic, Template_relevance Pistachio_ringbreaker, Template_relevance Reaxys, Template_relevance Reaxys_biocatalysis model, leveraging a vast database of chemical reactions to predict feasible synthetic routes.
One-Step Synthesis Focus: Specifically designed for one-step synthesis, it provides concise and direct routes for your target compounds, streamlining the synthesis process.
Accurate Predictions: Utilizing the extensive PISTACHIO, BKMS_METABOLIC, PISTACHIO_RINGBREAKER, REAXYS, REAXYS_BIOCATALYSIS database, our tool offers high-accuracy predictions, reflecting the latest in chemical research and data.
Strategy Settings
Precursor scoring | Relevance Heuristic |
---|---|
Min. plausibility | 0.01 |
Model | Template_relevance |
Template Set | Pistachio/Bkms_metabolic/Pistachio_ringbreaker/Reaxys/Reaxys_biocatalysis |
Top-N result to add to graph | 6 |
Feasible Synthetic Routes
Disclaimer and Information on In-Vitro Research Products
Please be aware that all articles and product information presented on BenchChem are intended solely for informational purposes. The products available for purchase on BenchChem are specifically designed for in-vitro studies, which are conducted outside of living organisms. In-vitro studies, derived from the Latin term "in glass," involve experiments performed in controlled laboratory settings using cells or tissues. It is important to note that these products are not categorized as medicines or drugs, and they have not received approval from the FDA for the prevention, treatment, or cure of any medical condition, ailment, or disease. We must emphasize that any form of bodily introduction of these products into humans or animals is strictly prohibited by law. It is essential to adhere to these guidelines to ensure compliance with legal and ethical standards in research and experimentation.