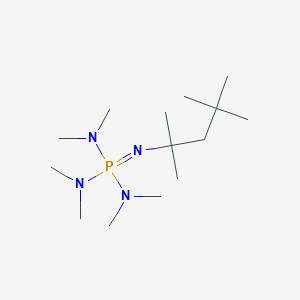
Phosphazene base P1-t-Oct
Overview
Description
Phosphazene base P1-t-Oct (tert-octylimino-tris(dimethylamino)phosphorane, CAS 161118-69-0) is a member of the P1-phosphazene family, characterized by a central phosphorus atom bonded to a tert-octylimino group and three dimethylamino substituents . Its structure combines high basicity with significant steric bulk due to the tert-octyl group, making it a valuable reagent in deprotonation and proton-transfer reactions. P1-t-Oct exhibits a pKa comparable to P1-t-Bu (tert-butylimino-tris(dimethylamino)phosphorane) but with enhanced steric hindrance, which influences reaction kinetics and substrate selectivity .
Preparation Methods
Synthetic Routes and Reaction Conditions
The synthesis of P1-t-Oct primarily involves nucleophilic substitution reactions between tert-octylamine and phosphorus-containing precursors. A widely adopted method utilizes tris(dimethylamino)phosphine (P(NMe₂)₃) as the starting material. The reaction proceeds via a two-step mechanism:
-
Formation of the Phosphazene Intermediate :
Tris(dimethylamino)phosphine reacts with tert-octylamine (t-C₈H₁₇NH₂) under anhydrous conditions, typically in tetrahydrofuran (THF) or hexane. The reaction is conducted at low temperatures (−20°C to 0°C) to mitigate side reactions, with rigorous exclusion of moisture and oxygen to prevent hydrolysis or oxidation . -
Purification and Isolation :
Crude product is purified via fractional distillation under reduced pressure (68°C at 0.05 mmHg) , yielding a colorless liquid with ≥97% purity .
Critical Parameters :
-
Stoichiometry : A 1:1 molar ratio of P(NMe₂)₃ to tert-octylamine ensures complete substitution.
-
Solvent Choice : THF enhances reaction homogeneity, while hexane facilitates easier isolation.
-
Catalysts : No external catalysts are required, but reaction rates improve with slight excesses of tert-octylamine.
Industrial-Scale Production
Industrial synthesis scales the laboratory protocol while addressing challenges in heat management and purity control:
-
Continuous Flow Reactors : Enable precise temperature control and reduce batch variability.
-
Distillation Columns : Multi-stage distillation under high vacuum (0.01–0.05 mmHg) removes residual amines and solvents .
-
Quality Control : Purity is verified via ³¹P NMR (singlet at δ ~20 ppm) and gas chromatography .
Parameter | Laboratory Scale | Industrial Scale |
---|---|---|
Reaction Volume | 5–50 mL | 100–500 L |
Temperature | −20°C to 0°C | −10°C to 5°C |
Yield | 75–85% | 80–90% |
Purity | ≥97% | ≥99% |
Mechanistic Studies and Kinetic Profiling
The reaction mechanism involves nucleophilic attack by tert-octylamine on the electrophilic phosphorus center of P(NMe₂)₃. Density functional theory (DFT) calculations reveal:
-
Transition State Stabilization : The tert-octyl group’s steric bulk lowers activation energy (ΔG‡ ≈ 18 kcal/mol) by stabilizing the trigonal bipyramidal transition state .
-
Solvent Effects : THF’s polarity stabilizes charged intermediates, accelerating the reaction compared to nonpolar solvents like hexane .
Kinetic Data :
Solvent | Rate Constant (k, s⁻¹) | Activation Energy (ΔH‡, kJ/mol) |
---|---|---|
THF | 3.2 × 10⁻³ | 24.5 |
Hexane | 1.8 × 10⁻³ | 28.1 |
Comparative Analysis with Analogous Bases
P1-t-Oct’s preparation contrasts with smaller phosphazenes like P1-t-Bu:
Base | Precursor Amine | Reaction Time (h) | Yield (%) |
---|---|---|---|
P1-t-Oct | tert-Octylamine | 24–48 | 75–85 |
P1-t-Bu | tert-Butylamine | 12–24 | 80–90 |
The extended reaction time for P1-t-Oct arises from steric hindrance slowing nucleophilic attack .
Emerging Methodologies and Innovations
Recent advances focus on solvent-free synthesis and green chemistry principles:
Chemical Reactions Analysis
Proton Transfer Reactions
P1-t-Oct exhibits exceptional efficiency in deprotonating carbon acids, such as nitroalkanes, due to its strong basicity and low nucleophilicity. Kinetic and thermodynamic studies in tetrahydrofuran (THF) reveal:
Equilibrium Constants (K)
Substrate | Temperature (°C) | K (dm³·mol⁻¹) |
---|---|---|
MNPNP⁺ | 15 | 7.6 ± 1.7 |
MNPNP | 25 | 5 ± 1 |
MNPNP | 45 | 2.4 ± 0.6 |
MNPNP : 1-nitro-1-(4-nitrophenyl)propane.
Equilibrium constants decrease with rising temperature, indicating an exothermic process (ΔH = −36 ± 1 kJ·mol⁻¹) .
Kinetic Parameters
Reactions with nitroalkanes follow second-order kinetics:
Substrate | Rate Constant (dm³·mol⁻¹·s⁻¹) | ΔH‡ (kJ·mol⁻¹) | ΔS‡ (J·mol⁻¹·K⁻¹) |
---|---|---|---|
NPNE‡ | 0.99 ± 0.03 | 27.3 ± 1.2 | −153 ± 4 |
MNPNP | 5 ± 1 | − | −211 |
NPNE : 1-nitro-1-phenylethane.
Large negative entropies of activation (ΔS‡) suggest highly ordered transition states influenced by steric hindrance .
Fluorination Reactions
P1-t-Oct forms stable hydrofluorides (e.g., P1-t-Oct·HF) for nucleophilic fluorination. Key findings include:
Reactivity Comparison
Substrate Type | P1-t-Oct·HF Yield (%) | P4tBu·HF Yield (%) |
---|---|---|
Alkyl Chlorides | 76–98 | 76–98 |
Electron-Deficient Arenes | <50 | <50 |
P1-t-Oct·HF shows instability toward autofluorolysis compared to bulkier analogs like P4tBu·HF, attributed to lower steric protection and higher electrophilicity .
Comparative Analysis with Other Phosphazene Bases
P1-t-Oct’s steric bulk (tert-octyl group) differentiates it from analogs:
Base | pKa Range | Steric Hindrance | Key Applications |
---|---|---|---|
P1-t-Oct | 26–42 | High | Proton transfer, fluorination |
P1-t-Bu | 26–42 | Moderate | Darzens condensations |
BTPP | 30–40 | High | Stronger basicity in polar solvents |
P1-t-Oct’s hindered structure reduces nucleophilicity, making it ideal for reactions requiring selective deprotonation without side reactions .
Staudinger Reaction
P1-t-Oct is synthesized via the Staudinger reaction between phosphazene precursors and tert-octylamine. This method introduces steric bulk, enhancing thermal stability and basicity .
Activation Parameters and Isotope Effects
Reaction System | Primary D-KIE⁂ | Interpretation |
---|---|---|
P1-t-Oct + NPNE | 1.8–2.2 | Moderate tunneling contribution |
P1-t-Oct + MNPNP | 1.5–1.7 | Steric desolvation effects |
D-KIE : Deuterium Kinetic Isotope Effect.
Lower D-KIE values compared to less hindered bases suggest steric effects dominate over tunneling .
Stability and Limitations
P1-t-Oct’s autofluorolysis in polar solvents limits its use in fluorination, whereas its hydrolytic stability in THF (>24 hours) ensures robust performance in organic synthesis .
Key Takeaways
- P1-t-Oct excels in deprotonating sterically hindered substrates.
- Its fluorination utility is constrained by stability but remains viable in anhydrous conditions.
- Comparative studies highlight its niche in high-steric-demand environments.
Experimental data underscore its role in advancing methodologies for complex molecule synthesis and materials science .
Scientific Research Applications
Chemical Properties and Characteristics
- Chemical Formula : C14H35N4P
- Molecular Weight : 290.43 g/mol
- CAS Number : 161118-69-0
- Basicity : Comparable to other strong bases like BEMP, with a pKBH+ value of approximately 26.5 in acetonitrile, indicating its strong nucleophilic character .
- Physical State : Liquid at room temperature with a boiling point of 68 °C at 0.05 mmHg .
Organic Synthesis
This compound is widely utilized in organic synthesis due to its ability to generate highly reactive anions in situ. This is particularly useful in reactions where traditional ionic bases may cause solubility issues or lead to unwanted side reactions.
- Alkylation Reactions : P1-t-Oct facilitates alkylation processes by deprotonating substrates effectively .
- Michael Additions : It has been successfully employed in Michael addition reactions, enhancing product yields and selectivity .
- Aldol Reactions : The steric hindrance of P1-t-Oct minimizes side reactions common with ionic bases, making it ideal for aldol condensations .
Catalysis
P1-t-Oct serves as a catalyst in various multi-component reactions, significantly improving reaction efficiency.
- Telescopic Three-Component Reactions : Recent studies demonstrate its effectiveness in catalyzing complex reactions involving diethyl phosphite and imines, showcasing its utility in synthetic methodologies .
Material Science
The stability and solubilizing properties of P1-t-Oct make it suitable for applications in material science.
- Polymerization Processes : It is increasingly used in anionic copolymerization techniques due to its ability to stabilize reactive intermediates .
- Solid-phase Synthesis : The compound has been integrated into solid-phase synthesis protocols, particularly in combinatorial chemistry, facilitating high-throughput screening of compounds .
Comparative Data Table
Property/Characteristic | This compound | BEMP (For Comparison) |
---|---|---|
Basicity (pKBH+) | 26.5 | 27.6 |
Solubility | High in apolar solvents | Moderate |
Stability | High against hydrolysis | Moderate |
Steric Hindrance | High | Moderate |
Case Study 1: Alkylation Efficiency
A study demonstrated that using P1-t-Oct for the alkylation of alcohols resulted in higher yields compared to traditional bases. The sterically hindered nature of the base reduced side reactions, allowing for cleaner product isolation.
Case Study 2: Michael Addition Optimization
In a series of Michael addition reactions involving α,β-unsaturated carbonyl compounds, the use of P1-t-Oct significantly improved reaction times and yields compared to conventional methods, highlighting its advantages as a superior base.
Mechanism of Action
Phosphazene base P1-t-Oct exerts its effects through its strong basicity, which allows it to deprotonate weakly acidic compounds effectively. The nitrogen-phosphorus hybrid structure provides a highly reactive anionic species associated with a very soft counterion. This unique structure enables it to participate in various organic transformations, including cyclization, annulation, and nucleophilic substitution reactions .
Comparison with Similar Compounds
Comparative Analysis with Similar Compounds
Structural and Basicity Comparisons
Phosphazene bases are classified by their substituents and backbone complexity. Key analogs of P1-t-Oct include:
Compound | Structure | Basicity (Relative Strength) | Key Substituents |
---|---|---|---|
P1-t-Oct | tert-octylimino-tris(dimethylamino)phosphorane | High | tert-octyl, dimethylamino |
P1-t-Bu | tert-butylimino-tris(dimethylamino)phosphorane | Similar to P1-t-Oct | tert-butyl, dimethylamino |
BTPP | tert-butylimino-tris(pyrrolidino)phosphorane | Strongest in P1 series | tert-butyl, pyrrolidino |
BEMP | 2-tert-butylimino-2-diethylamino-1,3-dimethyl-perhydrodiazaphosphorine | Intermediate | tert-butyl, diethylamino, phosphorine |
DBU (Guanidine) | 1,8-Diazabicycloundec-7-ene | Lower | Bicyclic guanidine |
Key Findings :
- BTPP exhibits the highest basicity in the P1 series due to electron-donating pyrrolidino groups, which enhance proton affinity .
- P1-t-Oct and P1-t-Bu share similar pKa values, but the tert-octyl group in P1-t-Oct introduces greater steric hindrance, slowing substrate approach in certain reactions .
- BEMP ’s phosphorine ring reduces steric bulk compared to P1-t-Oct, enabling faster kinetics in less hindered environments .
Reaction Kinetics and Activation Parameters
Studies on proton transfer reactions with nitroalkanes (e.g., NPNE and MNPNP) reveal distinct kinetic behaviors:
Table 2: Activation Parameters for MNPNP Deprotonation
Base | ∆S‡ (J mol⁻¹K⁻¹) | Energy Barrier (kJ mol⁻¹) |
---|---|---|
P1-t-Oct | –22 | 22.0 |
BTPP | –211 | Not reported |
DBU | –146 | 27.2 (for NPNE) |
Observations :
- P1-t-Oct shows a less negative entropy of activation (∆S‡ = –22 J mol⁻¹K⁻¹) compared to BTPP (∆S‡ = –211 J mol⁻¹K⁻¹), suggesting a less ordered transition state due to steric shielding .
- The energy barrier for MNPNP deprotonation with P1-t-Oct (22.0 kJ mol⁻¹) is lower than with P1-t-Bu (11–18 kJ mol⁻¹), indicating that steric bulk may paradoxically reduce energy costs in specific cases .
Steric and Electronic Effects on Reactivity
- Steric Hindrance : The tert-octyl group in P1-t-Oct slows deprotonation of bulky carbon acids (e.g., MNPNP) but enhances selectivity in sterically congested systems .
- Reversibility : P1-t-Oct achieves 95% reversibility in deprotonation of 1a-H+, outperforming analogs like BTPP (85% for 1b-H+) due to its balanced basicity and steric profile .
- Isomerization Control : In reactions with nitroalkanes, P1-t-Oct minimizes unwanted isomerization pathways, a limitation observed with guanidines like TBD .
Biological Activity
Phosphazene bases, particularly P1-t-Oct, are a class of highly basic compounds that have garnered attention for their diverse applications in organic synthesis and potential biological activities. This article explores the biological activity of P1-t-Oct, focusing on its antimicrobial properties, interactions with DNA, and implications for medicinal chemistry.
Overview of Phosphazene Base P1-t-Oct
This compound is characterized by its high basicity and steric hindrance, which makes it an effective catalyst in various chemical reactions. Its basicity is significantly higher than that of traditional bases like DBU (1,8-Diazabicyclo[5.4.0]undec-7-ene), making it suitable for deprotonation reactions in organic synthesis .
Antimicrobial Activity
Recent studies have highlighted the antimicrobial properties of phosphazene derivatives, including P1-t-Oct. In a comparative study of various phosphazene compounds, P1-t-Oct exhibited notable activity against both Gram-positive and Gram-negative bacteria.
Compound | Minimum Inhibitory Concentration (MIC) | Target Organism |
---|---|---|
P1-t-Oct | 125–500 μM | Pseudomonas aeruginosa |
Other derivatives | Varies (specific data not provided) | Various bacterial strains |
The results indicate that P1-t-Oct can inhibit the growth of pathogens such as Pseudomonas aeruginosa, which is known for its resistance to multiple antibiotics . The mechanism of action appears to involve disruption of bacterial cell membranes and interference with cellular processes.
Interaction with DNA
Phosphazene bases have also been studied for their interactions with nucleic acids. Specifically, research has shown that P1-t-Oct can interact with plasmid DNA, leading to cleavage of the double helix structure. This property is significant as it suggests potential applications in gene therapy and molecular biology.
- Experimental Findings :
Case Studies and Research Findings
Several case studies have provided insights into the biological applications of phosphazene bases:
- Antimicrobial Efficacy : A study evaluated the effectiveness of various phosphazene derivatives against a panel of bacterial strains. P1-t-Oct was among the most potent, particularly against resistant strains like Pseudomonas aeruginosa.
- DNA Cleavage : In another investigation, researchers explored the ability of phosphazenes to induce DNA cleavage. The findings revealed that compounds including P1-t-Oct could serve as tools for genetic engineering by facilitating targeted DNA modifications.
- Catalytic Applications : Beyond biological activity, P1-t-Oct has been successfully employed as a catalyst in organic reactions, demonstrating its versatility in both synthetic and biological contexts .
Q & A
Basic Research Questions
Q. What are the key physicochemical properties of P1-t-Oct that distinguish it from other phosphazene bases?
- Methodological Answer : P1-t-Oct is characterized by its high basicity (pKa ~29 in THF) and steric hindrance due to the bulky tert-octyl substituent at the imine nitrogen. Comparative studies with P1-t-Bu reveal similar basicity but increased steric bulk in P1-t-Oct, which reduces aggregation and enhances selectivity in deprotonation reactions. Key properties include solubility in aprotic solvents (e.g., THF), thermal stability, and kinetic activity in proton transfer reactions. Characterization typically involves NMR spectroscopy, mass spectrometry, and kinetic profiling using nitroalkanes as carbon acids .
Property | P1-t-Oct | P1-t-Bu | BEMP |
---|---|---|---|
Basicity (pKa, THF) | ~29 | ~28.5 | ~26 |
Substituent | tert-octyl | tert-butyl | tert-butyl + phosphorine ring |
Steric Hindrance | High | Moderate | Moderate |
Kinetic Reactivity | Slower due to sterics | Faster | Intermediate |
Q. How is P1-t-Oct synthesized, and what experimental protocols ensure reproducibility?
- Methodological Answer : P1-t-Oct is synthesized via nucleophilic substitution of hexachlorocyclotriphosphazene with tert-octylamine under anhydrous conditions. Critical steps include strict moisture control, inert atmosphere (argon/glovebox), and purification by recrystallization from hexane. Reproducibility hinges on precise stoichiometry (1:6 molar ratio of phosphazene to amine) and characterization via P NMR to confirm complete substitution (singlet at δ ~20 ppm). Detailed protocols are outlined in peer-reviewed syntheses, with validation through comparison of kinetic data from proton transfer reactions .
Q. What role does P1-t-Oct play in proton transfer reactions, and how is this experimentally validated?
- Methodological Answer : P1-t-Oct acts as a strong, sterically hindered base in deprotonating carbon acids (e.g., nitroalkanes). Experimental validation involves:
- Equilibrium Studies : Measuring via UV-Vis spectroscopy for nitroalkane deprotonation in THF.
- Kinetic Profiling : Using stopped-flow techniques to determine rate constants () and activation parameters (ΔH‡, ΔS‡) via Eyring plots.
Results are benchmarked against less hindered bases (e.g., BEMP) to isolate steric effects .
Advanced Research Questions
Q. How can researchers design experiments to isolate steric vs. electronic effects of P1-t-Oct in catalytic systems?
- Methodological Answer : Apply the PICOT framework to structure the research question:
- Population : Nitroalkanes (e.g., NPNE) as carbon acids.
- Intervention : Deprotonation using P1-t-Oct vs. structurally analogous bases (e.g., P1-t-Bu, BTPP).
- Comparison : Kinetic/thermodynamic data across bases with varying steric/electronic profiles.
- Outcome : Quantify contributions of steric hindrance (via substituent bulk) vs. basicity (via pKa).
- Timeframe : Reaction completion monitored over 24–72 hours.
Control variables include solvent polarity, temperature, and acid concentration. Use multivariate regression to deconvolute steric/electronic factors .
Q. What methodological challenges arise in kinetic studies of P1-t-Oct-mediated reactions, and how are they resolved?
- Methodological Answer : Key challenges include:
- Reproducibility : Moisture sensitivity of phosphazenes necessitates strict anhydrous protocols.
- Data Contradictions : Discrepancies in rate constants may stem from solvent impurities or incomplete base activation. Mitigation involves pre-drying solvents over molecular sieves and validating base activity via titration with benzoic acid.
- Aggregation Effects : Use low concentrations (<1 mM) to minimize base self-association, confirmed by dynamic light scattering (DLS) .
Q. How should researchers analyze contradictory data in comparative studies of P1-t-Oct and guanidine bases?
- Methodological Answer : Contradictions often arise from differences in reaction mechanisms (e.g., concerted vs. stepwise proton transfer). To resolve:
Mechanistic Probes : Isotopic labeling (N/H) to track proton transfer pathways.
Computational Modeling : DFT calculations (e.g., Gaussian) to compare transition states.
Statistical Validation : Use ANOVA to assess significance of rate differences across bases.
Cross-reference findings with solvent parameters (e.g., THF vs. DMSO) to contextualize results .
Q. What strategies optimize the use of P1-t-Oct in stereoselective synthesis?
- Methodological Answer : Leverage steric bulk to control enantioselectivity in deprotonation steps:
- Chiral Additives : Pair P1-t-Oct with chiral crown ethers to induce asymmetry.
- Temperature Modulation : Lower temperatures (-78°C) enhance stereochemical outcomes by slowing competing pathways.
- Substrate Engineering : Design nitroalkanes with proximal stereogenic centers to exploit steric guidance.
Monitor outcomes via chiral HPLC and H NMR with chiral shift reagents .
Q. Methodological Frameworks and Data Collection
Q. Which characterization techniques are critical for validating P1-t-Oct’s purity and structural integrity?
- Methodological Answer :
- NMR Spectroscopy : P NMR for phosphorus environment; H/C NMR for organic substituents.
- Mass Spectrometry : High-resolution ESI-MS to confirm molecular weight.
- Elemental Analysis : Carbon/hydrogen/nitrogen ratios to verify stoichiometry.
- Kinetic Consistency : Benchmark deprotonation rates against literature values (e.g., for NPNE in THF) .
Q. How can researchers systematically collect and interpret kinetic data for P1-t-Oct in multi-step reactions?
- Methodological Answer :
Data Collection : Use stopped-flow UV-Vis for rapid kinetics; integrate with HPLC for product quantification.
Data Structuring : Organize results into relational databases (e.g., SQL) with fields for solvent, temperature, and substrate structure.
Interpretation Tools : Apply Eyring-Polanyi equations for activation parameters and machine learning (e.g., Python’s Scikit-learn) to identify rate-determining steps .
Q. What ethical and reproducibility guidelines apply to publishing studies involving P1-t-Oct?
- Methodological Answer : Follow FAIR principles (Findable, Accessible, Interoperable, Reusable):
- Documentation : Publish full synthetic protocols, including glovebox procedures and solvent purification steps.
- Data Sharing : Deposit raw kinetic data in repositories (e.g., Zenodo) with DOI links.
- Peer Review : Disclose potential conflicts (e.g., commercial phosphazene suppliers) and validate methods via inter-laboratory reproducibility trials .
Properties
IUPAC Name |
N-[bis(dimethylamino)-(2,4,4-trimethylpentan-2-ylimino)-λ5-phosphanyl]-N-methylmethanamine | |
---|---|---|
Source | PubChem | |
URL | https://pubchem.ncbi.nlm.nih.gov | |
Description | Data deposited in or computed by PubChem | |
InChI |
InChI=1S/C14H35N4P/c1-13(2,3)12-14(4,5)15-19(16(6)7,17(8)9)18(10)11/h12H2,1-11H3 | |
Source | PubChem | |
URL | https://pubchem.ncbi.nlm.nih.gov | |
Description | Data deposited in or computed by PubChem | |
InChI Key |
DSCJCKAURXOQPX-UHFFFAOYSA-N | |
Source | PubChem | |
URL | https://pubchem.ncbi.nlm.nih.gov | |
Description | Data deposited in or computed by PubChem | |
Canonical SMILES |
CC(C)(C)CC(C)(C)N=P(N(C)C)(N(C)C)N(C)C | |
Source | PubChem | |
URL | https://pubchem.ncbi.nlm.nih.gov | |
Description | Data deposited in or computed by PubChem | |
Molecular Formula |
C14H35N4P | |
Source | PubChem | |
URL | https://pubchem.ncbi.nlm.nih.gov | |
Description | Data deposited in or computed by PubChem | |
DSSTOX Substance ID |
DTXSID90408011 | |
Record name | Phosphazene base P1-t-Oct | |
Source | EPA DSSTox | |
URL | https://comptox.epa.gov/dashboard/DTXSID90408011 | |
Description | DSSTox provides a high quality public chemistry resource for supporting improved predictive toxicology. | |
Molecular Weight |
290.43 g/mol | |
Source | PubChem | |
URL | https://pubchem.ncbi.nlm.nih.gov | |
Description | Data deposited in or computed by PubChem | |
CAS No. |
161118-69-0 | |
Record name | Phosphazene base P1-t-Oct | |
Source | EPA DSSTox | |
URL | https://comptox.epa.gov/dashboard/DTXSID90408011 | |
Description | DSSTox provides a high quality public chemistry resource for supporting improved predictive toxicology. | |
Record name | Phosphazene base P1-t-Oct | |
Source | European Chemicals Agency (ECHA) | |
URL | https://echa.europa.eu/information-on-chemicals | |
Description | The European Chemicals Agency (ECHA) is an agency of the European Union which is the driving force among regulatory authorities in implementing the EU's groundbreaking chemicals legislation for the benefit of human health and the environment as well as for innovation and competitiveness. | |
Explanation | Use of the information, documents and data from the ECHA website is subject to the terms and conditions of this Legal Notice, and subject to other binding limitations provided for under applicable law, the information, documents and data made available on the ECHA website may be reproduced, distributed and/or used, totally or in part, for non-commercial purposes provided that ECHA is acknowledged as the source: "Source: European Chemicals Agency, http://echa.europa.eu/". Such acknowledgement must be included in each copy of the material. ECHA permits and encourages organisations and individuals to create links to the ECHA website under the following cumulative conditions: Links can only be made to webpages that provide a link to the Legal Notice page. | |
Disclaimer and Information on In-Vitro Research Products
Please be aware that all articles and product information presented on BenchChem are intended solely for informational purposes. The products available for purchase on BenchChem are specifically designed for in-vitro studies, which are conducted outside of living organisms. In-vitro studies, derived from the Latin term "in glass," involve experiments performed in controlled laboratory settings using cells or tissues. It is important to note that these products are not categorized as medicines or drugs, and they have not received approval from the FDA for the prevention, treatment, or cure of any medical condition, ailment, or disease. We must emphasize that any form of bodily introduction of these products into humans or animals is strictly prohibited by law. It is essential to adhere to these guidelines to ensure compliance with legal and ethical standards in research and experimentation.