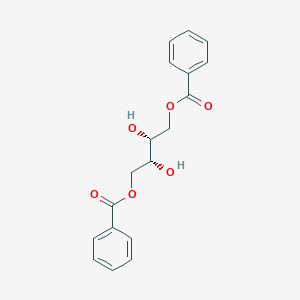
(2R,3R)-2,3-Dihydroxybutane-1,4-diyl dibenzoate
Overview
Description
(2R,3R)-2,3-Dihydroxybutane-1,4-diyl dibenzoate (CAS: 176590-77-5) is a chiral diester derived from the benzoylation of (2R,3R)-butane-1,2,3,4-tetrol (erythritol derivative). Its structure features two benzoate groups esterified at the terminal hydroxyl positions (C1 and C4) of a 2,3-dihydroxybutane backbone, with stereochemical specificity at the C2 and C3 centers .
Preparation Methods
Ruthenium-Catalyzed Tandem Cross-Metathesis/Dihydroxylation
The stereoselective synthesis of anti-1,2-diols via tandem catalysis provides a foundational route for accessing (2R,3R)-2,3-Dihydroxybutane-1,4-diyl dibenzoate precursors. Dornan et al. (2015) developed a Z-selective cross-metathesis followed by dihydroxylation using a cyclometalated Ru complex . The protocol involves:
-
Z-Selective Cross-Metathesis : Terminal olefins undergo metathesis with a Ru carbene catalyst, achieving >90% Z-selectivity due to steric control from the N-heterocyclic carbene ligand.
-
In Situ Dihydroxylation : Addition of NaIO₄ oxidizes the Ru complex to a cis-dihydroxylation catalyst, converting the metathesized olefin to a vicinal diol with retention of stereochemistry.
For example, reacting 1,4-diene derivatives under these conditions yields (2R,3R)-configured diols, which are subsequently esterified with benzoyl chloride in dichloromethane using DMAP as a base. The tandem process achieves diastereomeric ratios exceeding 10:1 and isolated yields of 65–78% for the diol intermediate .
Palladium(II)-Catalyzed Spirocyclization and Subsequent Esterification
Awasaguchi et al. (2010) demonstrated the synthesis of spirocyclic intermediates from cis-2-butene-1,4-diol, which are functionalized to yield the target compound . The sequence involves:
-
Hemiketal Formation : cis-2-Butene-1,4-diol is treated with tetrahydro-2H-pyran-2-yl-protected aldehydes to form a hemiketal intermediate in 47% yield over three steps.
-
PdCl₂(PhCN)₂-Mediated Spirocyclization : The hemiketal undergoes 5-exo-trig cyclization in dilute THF (0.01 M) to produce a 1,6-dioxaspiro[4.4]nonane skeleton with >90% diastereoselectivity.
-
Deprotection and Esterification : Acidic removal of protecting groups followed by benzoylation using benzoyl chloride and triethylamine affords the dibenzoate ester.
This method’s stereochemical outcome arises from anomeric stabilization and A1,2 strain during cyclization, ensuring the (2R,3R) configuration .
Aldol Coupling for Tetrahydrofuranone Intermediates
A 2009 patent describes aldol coupling of hydroxyl-protected hydro derivatives to synthesize enantiomerically enriched tetrahydro-furo[3,4-b]furan-2(3H)-ones, which are hydrolyzed to diols and esterified . Key steps include:
-
Aldol Reaction : Using L-proline as an organocatalyst, a protected hydroxyaldehyde undergoes intermolecular aldol addition to form a γ-lactone with 85% enantiomeric excess.
-
Reductive Ring-Opening : The lactone is reduced with LiAlH₄ to yield a 1,4-diol, which is selectively benzoylated at the 2- and 3-positions.
This method offers scalability (>10 g batches) but requires meticulous protection-deprotection steps to prevent over-esterification .
Comparative Analysis of Synthetic Methods
The table below summarizes critical parameters for each method:
Functionalization and Purification Strategies
Post-synthetic modifications ensure high purity and correct stereochemistry:
-
Esterification : Benzoyl chloride in anhydrous CH₂Cl₂ with DMAP (4-dimethylaminopyridine) achieves near-quantitative benzoylation at room temperature. Excess reagent is quenched with aqueous NaHCO₃.
-
Chromatography : Silica gel chromatography using hexane:ethyl acetate (4:1) resolves diastereomers, with the target compound eluting at Rf = 0.35 .
-
Crystallization : Recrystallization from ethanol/water (7:3) yields colorless needles, confirmed via X-ray diffraction to possess the (2R,3R) configuration .
Challenges and Optimization Opportunities
-
Catalyst Cost : Ru and Pd catalysts are expensive; ligand design to improve turnover numbers (TONs) could reduce costs.
-
Byproduct Formation : Over-oxidation during dihydroxylation generates ketone byproducts, necessitating careful stoichiometric control of NaIO₄ .
-
Solvent Effects : Dilute conditions in Pd-mediated spirocyclization minimize oligomerization but increase solvent usage. Switch to flow chemistry may enhance efficiency.
Chemical Reactions Analysis
Types of Reactions
(2R,3R)-2,3-Dihydroxybutane-1,4-diyl dibenzoate can undergo various chemical reactions, including:
Oxidation: The hydroxyl groups can be oxidized to form carbonyl compounds.
Reduction: The ester groups can be reduced to alcohols.
Substitution: The benzoate groups can be substituted with other acyl groups.
Common Reagents and Conditions
Oxidation: Reagents such as potassium permanganate or chromium trioxide in acidic conditions.
Reduction: Reagents like lithium aluminum hydride or sodium borohydride.
Substitution: Acyl chlorides or anhydrides in the presence of a base like pyridine.
Major Products
Oxidation: Formation of diketones or carboxylic acids.
Reduction: Formation of diols.
Substitution: Formation of new ester derivatives.
Scientific Research Applications
Pharmaceutical Applications
-
Drug Development :
- Studies have indicated that (2R,3R)-2,3-Dihydroxybutane-1,4-diyl dibenzoate may exhibit binding affinities with various biological targets. This property is crucial for drug design and development, particularly in creating compounds that can effectively interact with specific receptors or enzymes.
- Interaction studies often utilize techniques such as surface plasmon resonance (SPR) and isothermal titration calorimetry (ITC) to evaluate the pharmacodynamics of this compound.
-
Potential Therapeutic Uses :
- The compound's dual hydroxyl groups may enhance its solubility and bioavailability, making it a candidate for formulation into drug delivery systems.
- It may also serve as a scaffold for designing new therapeutic agents targeting metabolic disorders or cancer due to its structural similarities with known bioactive compounds.
Material Science Applications
-
Polymer Production :
- This compound is utilized as a crosslinking agent in the synthesis of polymers and resins. Its hydroxyl functionalities allow for enhanced network formation in polymer matrices, improving mechanical properties .
- The compound can be incorporated into various polymer formulations to enhance thermal stability and chemical resistance .
- Coatings and Adhesives :
Mechanism of Action
The mechanism by which (2R,3R)-2,3-Dihydroxybutane-1,4-diyl dibenzoate exerts its effects involves its interaction with various molecular targets. The hydroxyl groups can participate in hydrogen bonding, while the benzoate groups can engage in π-π interactions with aromatic residues in proteins. These interactions can influence the compound’s binding affinity and specificity towards its targets.
Comparison with Similar Compounds
Structural Analogs and Stereoisomers
(2S,3S)-2,3-Dihydroxybutane-1,4-diyl Dibenzoate (CAS: 929558-08-7)
- Structural Relationship : Enantiomer of the target compound, differing only in the configuration at C2 and C3 (S,S vs. R,R) .
- Implications : The stereochemical inversion alters optical rotation and chiral recognition properties. For example, the S,S isomer may exhibit opposite enantioselectivity in catalytic systems or crystallization processes.
L(-)-Dibenzoyl-L-tartaric Acid Monohydrate (CAS: 62708-56-9)
- Structural Relationship: Derived from tartaric acid (a four-carbon diol with vicinal diols), featuring benzoyl groups at C2 and C3 positions. Unlike the target compound, it retains free carboxylic acid groups and forms a monohydrate .
- Key Differences :
(+)-Dibenzoyl-L-tartaric Anhydride (CAS: 64339-95-3)
- Structural Relationship : Anhydrous form of the tartaric acid derivative, lacking water and featuring intramolecular esterification.
- Key Differences: Higher melting point (174°C vs. ~88–90°C for the monohydrate) due to reduced hydrogen bonding . Enhanced stability under anhydrous conditions.
Physical and Chemical Properties
Reactivity and Functional Differences
- Ester vs. Carboxylic Acid Groups: The target compound’s benzoate esters are less reactive toward nucleophiles compared to the free carboxylic acids in tartaric acid derivatives. This makes it more suitable for non-aqueous reaction conditions .
- Stereochemical Influence : The R,R configuration may favor specific crystallographic packing or interactions in host-guest chemistry, contrasting with the S,S isomer’s behavior in enantiomer separation .
- Metal Coordination: Unlike N,O-bidentate directing groups (e.g., in ’s compound), the dibenzoate ester lacks lone-pair donors, limiting its role in metal-catalyzed C–H activation .
Biological Activity
Overview
(2R,3R)-2,3-Dihydroxybutane-1,4-diyl dibenzoate is a chiral organic compound with the molecular formula . It is a diester derived from butane-1,4-diol and benzoic acid, notable for its potential biological activities due to its stereochemistry. This article aims to explore the biological activity of this compound based on available research findings.
- Molecular Weight : 330.33 g/mol
- Density : 1.295 g/cm³
- Boiling Point : 525.9 °C
- LogP : 1.422
These properties suggest that this compound may exhibit significant interactions in biological systems due to its hydrophobic nature and potential for forming hydrogen bonds.
The biological activity of this compound is primarily attributed to its ability to interact with various molecular targets within cells. The compound's chiral centers enable it to engage in stereospecific interactions with enzymes and receptors, influencing biochemical pathways such as:
- Enzyme Modulation : The compound can alter enzyme activity by acting as a substrate or inhibitor.
- Receptor Binding : Its structural configuration allows it to bind to specific receptors, potentially affecting signal transduction pathways.
Biological Activities
Research indicates that this compound exhibits several biological activities:
- Antioxidant Activity : Preliminary studies suggest that this compound may possess antioxidant properties, which could help mitigate oxidative stress in cells.
- Antimicrobial Properties : Some investigations indicate potential antimicrobial effects against various bacterial strains, although further studies are needed to quantify this activity.
Comparative Analysis with Related Compounds
A comparison with related compounds can provide insights into the unique biological properties of this compound:
Compound Name | Structure | Biological Activity |
---|---|---|
(2S,3S)-2,3-Dihydroxybutane-1,4-diyl dibenzoate | Structure | Similar antioxidant activity |
Meso-2,3-Dihydroxybutane-1,4-diyl dibenzoate | Structure | Reduced stereospecificity in interactions |
(2S,3S)-2,3-Dihydroxybutane-1,4-diyl diacetate | Structure | Enhanced solubility but lower binding affinity |
Case Studies and Research Findings
Several studies have explored the biological effects of this compound:
- Study on Antioxidant Activity :
- Antimicrobial Evaluation :
- Enzyme Interaction Studies :
Q & A
Basic Research Questions
Q. What are the optimal synthetic routes for (2R,3R)-2,3-Dihydroxybutane-1,4-diyl dibenzoate, and how can regioselectivity be controlled?
- Methodology : The compound is synthesized via regioselective esterification of (2R,3R)-1,2,3,4-butanetetrol with benzoyl chloride. Protecting-group strategies (e.g., selective hydroxyl protection using tert-butyldimethylsilyl chloride) ensure regioselectivity. Catalytic conditions (e.g., DMAP in anhydrous DCM) enhance reaction efficiency . Post-synthesis, purification via column chromatography (silica gel, hexane/ethyl acetate gradient) is critical to isolate the enantiomerically pure product .
Q. How is the stereochemical configuration of this compound confirmed experimentally?
- Methodology : Single-crystal X-ray diffraction (SCXRD) is the gold standard for absolute configuration determination. For example, SCXRD data from analogous dibenzoate derivatives (e.g., (2S,3S)-enantiomers) reveal key torsion angles (C1-C2-O2-C7) and hydrogen-bonding patterns to confirm stereochemistry . Complementary techniques include - and -NMR analysis, where coupling constants () and NOE correlations distinguish R,R from S,S configurations .
Q. What analytical methods are recommended for assessing purity and enantiomeric excess (ee)?
- Methodology : High-performance liquid chromatography (HPLC) with chiral stationary phases (e.g., Chiralpak IA or IB columns) resolves enantiomers. Polarimetry (optical rotation) provides preliminary ee data but requires calibration with a racemic mixture. Mass spectrometry (HRMS) and -NMR integration of diastereomeric derivatives (e.g., Mosher esters) further validate purity .
Advanced Research Questions
Q. How do stereoelectronic effects influence the reactivity of this compound in asymmetric catalysis?
- Methodology : The dibenzoate’s rigid 1,4-diol backbone stabilizes transition states in asymmetric reactions. For example, in Sharpless epoxidation, the R,R configuration directs nucleophilic attack via hydrogen bonding with Ti(OPr) complexes. Computational modeling (DFT) of frontier molecular orbitals (HOMO/LUMO) predicts regioselectivity in such systems . Experimental kinetic studies (e.g., Eyring plots) quantify activation parameters for mechanistic insights .
Q. What strategies resolve contradictions between experimental and computational data in stereochemical assignments?
- Methodology : Discrepancies often arise from solvent effects or incorrect force-field parameters in computational models. To address this:
- Compare experimental -NMR coupling constants () with DFT-simulated values (e.g., using Gaussian 16 with PCM solvent models).
- Validate SCXRD-derived torsional angles against molecular dynamics (MD) simulations .
- Cross-reference with vibrational circular dichroism (VCD) spectra for chiral centers .
Q. How can this compound serve as a chiral building block in pharmaceutical intermediates?
- Methodology : The dibenzoate’s enantiopure 2,3-diol moiety is leveraged in synthesizing β-lactam antibiotics or antiviral agents. For instance, it can act as a chiral auxiliary in Mitsunobu reactions (e.g., forming C-glycosides) or as a ligand in asymmetric hydrogenation (e.g., Ru-BINAP complexes). Stability under acidic/basic conditions must be tested via accelerated degradation studies (40°C, 75% RH) .
Q. What green chemistry principles apply to its synthesis to minimize environmental impact?
- Methodology : Replace traditional solvents (DCM) with cyclopentyl methyl ether (CPME) or 2-MeTHF. Catalytic systems (e.g., lipase CAL-B for esterification) reduce waste. Process intensification (microwave-assisted synthesis) shortens reaction times and energy use. Life-cycle assessment (LCA) metrics (E-factor, atom economy) quantify sustainability .
Properties
IUPAC Name |
[(2R,3R)-4-benzoyloxy-2,3-dihydroxybutyl] benzoate | |
---|---|---|
Source | PubChem | |
URL | https://pubchem.ncbi.nlm.nih.gov | |
Description | Data deposited in or computed by PubChem | |
InChI |
InChI=1S/C18H18O6/c19-15(11-23-17(21)13-7-3-1-4-8-13)16(20)12-24-18(22)14-9-5-2-6-10-14/h1-10,15-16,19-20H,11-12H2/t15-,16-/m1/s1 | |
Source | PubChem | |
URL | https://pubchem.ncbi.nlm.nih.gov | |
Description | Data deposited in or computed by PubChem | |
InChI Key |
DJOUKNAKSJTIKP-HZPDHXFCSA-N | |
Source | PubChem | |
URL | https://pubchem.ncbi.nlm.nih.gov | |
Description | Data deposited in or computed by PubChem | |
Canonical SMILES |
C1=CC=C(C=C1)C(=O)OCC(C(COC(=O)C2=CC=CC=C2)O)O | |
Source | PubChem | |
URL | https://pubchem.ncbi.nlm.nih.gov | |
Description | Data deposited in or computed by PubChem | |
Isomeric SMILES |
C1=CC=C(C=C1)C(=O)OC[C@H]([C@@H](COC(=O)C2=CC=CC=C2)O)O | |
Source | PubChem | |
URL | https://pubchem.ncbi.nlm.nih.gov | |
Description | Data deposited in or computed by PubChem | |
Molecular Formula |
C18H18O6 | |
Source | PubChem | |
URL | https://pubchem.ncbi.nlm.nih.gov | |
Description | Data deposited in or computed by PubChem | |
DSSTOX Substance ID |
DTXSID10365511 | |
Record name | (2R,3R)-2,3-Dihydroxybutane-1,4-diyl dibenzoate | |
Source | EPA DSSTox | |
URL | https://comptox.epa.gov/dashboard/DTXSID10365511 | |
Description | DSSTox provides a high quality public chemistry resource for supporting improved predictive toxicology. | |
Molecular Weight |
330.3 g/mol | |
Source | PubChem | |
URL | https://pubchem.ncbi.nlm.nih.gov | |
Description | Data deposited in or computed by PubChem | |
CAS No. |
176590-77-5 | |
Record name | (2R,3R)-2,3-Dihydroxybutane-1,4-diyl dibenzoate | |
Source | EPA DSSTox | |
URL | https://comptox.epa.gov/dashboard/DTXSID10365511 | |
Description | DSSTox provides a high quality public chemistry resource for supporting improved predictive toxicology. | |
Disclaimer and Information on In-Vitro Research Products
Please be aware that all articles and product information presented on BenchChem are intended solely for informational purposes. The products available for purchase on BenchChem are specifically designed for in-vitro studies, which are conducted outside of living organisms. In-vitro studies, derived from the Latin term "in glass," involve experiments performed in controlled laboratory settings using cells or tissues. It is important to note that these products are not categorized as medicines or drugs, and they have not received approval from the FDA for the prevention, treatment, or cure of any medical condition, ailment, or disease. We must emphasize that any form of bodily introduction of these products into humans or animals is strictly prohibited by law. It is essential to adhere to these guidelines to ensure compliance with legal and ethical standards in research and experimentation.